The focus of health care delivery is shifting toward modalities that provide the best value for patients, which requires optimization of the physical, emotional, and economic aspects of care. Se Assuming oncologic outcomes are maintained, the adoption of minimally invasive surgery (MIS) appears to provide maximum value for patients requiring surgery for gynecologic cancers. Since the first reports of laparoscopic pelvic and para-aortic lymphadenectomy in the early 1990s (1,2), MIS techniques have been successfully applied to the surgical treatment of most gynecologic cancers. Laparoscopic approaches have been described for endometrial cancer (3), cervical cancer (4), and ovarian cancer (5,6), involving complex procedures such as radical hysterectomy (7), pelvic and para-aortic lymphadenectomy and, less successfully, ovarian cancer debulking (8,9). Numerous retrospective series have highlighted the advantages of laparoscopy over laparotomy for both endometrial cancer (10,11) and cervical cancer (12). Several prospective randomized studies have demonstrated that treatment of endometrial cancer by laparoscopy significantly reduces morbidity without sacrificing oncologic outcome (13–15). Benefits include shorter hospitalization, reduced postoperative complications, improved quality of life, and reduced risk of major surgical adverse events (16–18).
Surgeons have encountered a long and steep learning curve for laparoscopy as a result of the limited degree of motion and dexterity offered by the rigid instruments, and the dependence, until very recently, on two-dimensional video images. This has resulted in longer operative times, leading to significant surgeon fatigue, physical strain, and even injury (19). Although experienced, highly skilled laparoscopic surgeons have challenged the limits of laparoscopy for gynecologic malignancies over the past 20 years, until recently adoption of MIS has been limited. Members of the Society of Gynecologic Oncology (SGO) were surveyed in 2002 and although 84% performed some laparoscopic surgery, 80% of these cases were either for diagnosis of adnexal mass or prophylactic bilateral salpingo-oophorectomy for women at high risk. Only 56% considered laparoscopy appropriate for endometrial cancer staging, and only 3% of respondents used laparoscopy for more than 50% of their surgeries (20). The broad application of laparoscopy for gynecologic oncology has been hindered by the heterogeneity of gynecologic cancer patients, who are often elderly, obese, and have multiple comorbidities. The largest randomized trial comparing laparoscopy to laparotomy for endometrial cancer staging (LAP2) showed that the rate of conversion to laparotomy for women with body mass index (BMI) >40 kg/m2 was 57%, and for each 10-year increase in age, the chance of conversion increased by 30% (13). Overall, the complexity of oncologic procedures and the resulting long learning curve have made it difficult to safely transition to MIS.
Figure 22.1 Adoption of minimally invasive surgical techniques for endometrial and cervical cancer surgery. Introduction of a robotics program in 2007 increased use of MIS from 17% to 66% within 12 months and to over 95% thereafter. (McGill University, Jewish General Hospital, Montreal, Canada) (36).
For many years, laparoscopy appeared to represent an alternative approach offered by expert surgeons with special skills that benefitted only a small number of patients. With the development of new techniques and technologies, it has been possible for a greater number of surgeons to apply MIS to more complex surgery, and to extend the associated benefits to a greater proportion of patients. Examples include the development improved optics, enhanced laparoscopic instrumentation such as bipolar coagulators (e.g., harmonic scalpel, Ligasure, and Plasma Kinetic) (21), and strong endoscopic bags, which allow complex masses and bulky uteri to be removed without spillage of tumor tissue (22). A major advancement leading to increased application of MIS in gynecologic oncology has been the development and implementation of robotic surgical technology. Since the advent of robotics, there has been a very rapid increase in the use of MIS for women with gynecologic malignancies (Fig. 22.1). The aim of this chapter is to evaluate how robotics can complement laparoscopy, and further decrease the use of laparotomy for the treatment of gynecologic malignancies.
Robotic Surgery: A Novel MIS Technology
The term “robotics” refers to the introduction of a computer interface and the integration of mechanical arms between the surgeon and the patient. This technology provides increased 7-degree freedom in instrumental range of motion, three-dimensional stereoscopic immersion optics, and wristed instrumentation that allows increased dexterity and tremor reduction, while improving surgeon comfort and reducing surgeon fatigue (23). Robotics has permitted surgeons to apply open surgical techniques to the MIS setting, thereby increasing adoption of MIS, with a lower rate of conversion to laparotomy even in complicated, elderly, and obese patients (24,25).
Robotic surgical systems were originally developed by NASA (US National Aeronautics and Space Administration) to permit surgery in space. They were adopted by the United States military to allow field surgery from a distance (26). After this prototype robotic system was acquired by the surgeon and entrepreneur Frederic H. Moll in 1995, it was incorporated into a new company called Intuitive Surgical Devices, Inc. (Sunnyvale, California), and by the turn of the century, it had been transformed into the first commercially available surgical robot, named the da Vinci Surgical System.
The first series of robotic hysterectomies was reported in 2002 using the da Vinci system, which included one hysterectomy for endometrial cancer (27). In 2005, several robotic surgical series for gynecologic cancer were reported (28,29), and the da Vinci system was approved by the FDA (U.S. Food and Drug Administration) for MIS in gynecologic surgery. By 2008, the feasibility of robotic surgery for endometrial cancer staging was well established, and was shown to have significantly less postoperative morbidity than laparotomy (30).
The introduction of robotics has corresponded with a rapid increase in the application of MIS to endometrial and cervical cancer in particular (31–33). A role has been reported for the staging of early ovarian cancer, and for the treatment of advanced and recurrent disease (34). The use of robotics has helped increase the proportion of women who are able to benefit from the advantages of MIS, particularly for a certain subset of patients, including the elderly and those with an elevated BMI (25,35,36). The improved ergonomics afforded by the seated position of the surgeon may decrease surgeon fatigue and injury (37). While many groups report rapid utilization after acquisition of robotic systems, with increases in some centers from 17% to 98% within 2 years (Fig. 22.1) (32), others have questioned whether the technology has been implemented without adequate scientific support (38). As with many innovations, the adoption of robotics for gynecologic cancer surgery has stimulated controversy, particularly regarding the economics of this expensive technology (39).
Robotic System: da Vinci Surgical System
Although there are several robotic systems in development, the only commercially available system is the da Vinci Surgical System (Intuitive Surgical, Inc., Sunnyvale, California, USA), and all publications regarding robotic surgery for gynecologic cancers have utilized this system. The da Vinci surgical system comprises three major components (Fig. 22.2):
1. Patient-side Cart
2. Computer stand-interface
3. Surgeon console
The surgeon’s movements are transmitted from the Surgeon Console through the Computer Interface, and result in movement of the instrument arms on the Patient-side Cart. The camera images are relayed from the camera arm on the Patient-side Cart, through the Computer Interface, and are displayed on the image visor of the Surgeon Console.
Figure 22.2 Components of the latest generation da Vinci Robot, the Si Model: (A) Patient-side Cart (B) Computer Stand/interface (C) Surgeon Console. Arm 3 can be placed on the right (as presented here) or to the left of the column depending on the surgeon’s preference.
Figure 22.3 Three generations of the da Vinci robot: Standard Model (2000), S Model (2006), and Si Model (2009).
There have been four generations of the da Vinci Surgical System, named the Standard, S, Si, and Xi (Fig. 22.3–Xi not shown). Each generation has incorporated a variety of improvements, including more efficient docking clamps, improved ergonomics at the surgeon console, and finger clutching to allow more efficient master control adjustments. Although the current da Vinci systems lack haptic/tactile feedback, surgeons report that the 3D stereoscopic vision system allows for adequate assessment of tissue consistency and control of instrument tension. The new da Vinci Xi version, just introduced in 2014, has a similar console; but the interface and the patient-side cart have been significantly upgraded, with new trocars, camera, and docking mechanisms.
Robotic System Components
Patient-side Cart
This mobile unit (Fig. 22.2A) is positioned beside the patient (Fig. 22.4), and supports the central camera arm and three instrument arms, which are numbered 1, 2, and 3. The Patient-side Cart is “docked” to the patient by latching each of these arms to special robotic trocars, which are inserted into the patient’s abdomen, similar to laparoscopy. The third instrument arm can be moved to either the right or left side of the Patient-side Cart, depending on the planned procedure. The camera is inserted into the central camera arm, and selected operative instruments can be inserted through instrument arms 1 and 3.
Figure 22.4 Operating room with robotic system set-up (left-sided docking).
Computer Stand-interface
A computer interface is placed between the Surgeon Console and the Patient-side Cart (Fig. 22.2B). It integrates and transmits information between the surgeon and the Patient-side Cart. This tower includes the gas insufflator and energy source controls.
Surgeon Console
The surgeon sits at the Surgeon Console (Fig. 22.2C) and views the operative field through a high definition, three-dimensional immersion image visor (Fig. 22.5A). None of the robotic arms can move until the surgeon’s face is placed against the image visor. Switches on the left side of the Surgeon Console control ergonomic adjustments of the image visor, arm board, and foot pedals to maximize surgeon comfort, allowing the surgeon to maintain a comfortable position throughout the procedure (Fig. 22.5C). A selection screen on the arm board allows for adjustments to a variety of system parameters (e.g., audio, video, and mechanical) and allows for designation of instrument control if using a Dual-Console for teaching (Fig. 22.5E). The Surgeon Console includes hand controls (Fig. 22.5F) and foot pedals (Fig. 22.6), which give the surgeon complete control over the camera and instruments while performing surgical tasks. A clutching mechanism allows the surgeon to adjust the arms and hands to a comfortable position without moving the robotic instruments, allowing the surgeon to continuously operate “in front of her/him,” irrespective of how the robotic arms are oriented in the patient’s abdomen.
Figure 22.5 da Vinci Robot Si Model surgeon console components.
Figure 22.6 da Vinci Robot Si Model: Surgeon Console Foot Pedal components and functions.
Surgeon Console—Hand Controls
There are master control handles into which fingers from each of the surgeon’s hands fit into one of two master handles to control the movement of the robotic arms and instrument’s tips (Fig. 22.5F). In conjunction with the camera pedals, they control camera functions. Twisting the control handles causes the tips of the instruments to rotate, while squeezing the control handles results in opening/closing of the instrument tips. With the newest Si model, pulling on the finger-clutch levers allows for comfortable adjustment of the surgeon’s arms and hands, similar to the foot clutch.
Surgeon Console—Foot Pedals
The ground board contains multiple foot pedals that activate functions associated with different instrument arms (Fig. 22.6):
(a) Toggle pedal—this side pedal toggles control of the three instrument arms, such that at any time two arms can be moved. The third inactivated arm is maintained in the position it was last located, including any grasps.
(b) Camera pedal—when this foot pedal is activated and held, the robotic arms are immobilized and the hand movements control the camera, including focus, zoom, and camera movements.
(c) Instrument Foot-clutch pedal—when the instrument clutch is activated, the arms can be moved without the instrument arms moving, allowing the surgeon to place arms back into a comfortable position wherever operating in the abdomen. On the newer Si model, the clutch is available as a hand clutch (Fig. 22.5G) or a foot clutch, while on the S mode, it is only available as a foot clutch (Fig. 22.6C).
(d) Right-sided foot pedal lower—usually allocated to monopolar coagulation (e.g., Curved scissors, Fig. 22.7A).
(e) Left-sided foot pedal lower—usually allocated to bipolar cautery (e.g., Maryland grasper, or Fenestrated Grasper, Fig. 22.7B).
(f) Right-sided foot pedal upper—usually allocated to monopolar cutting.
(g) Left-sided foot pedal upper—multiple use pedal—for additional allocations.
Figure 22.7 Commonly used instruments in gynecologic cancer surgeries.
Patient Positioning, Docking, and Instrumentation
Preparation and positioning is particularly important for robotic surgery because after the robot is docked, the Patient-side Cart and operative table cannot be moved without undocking. Successful application of robotics requires a well-trained operating room team to ensure that preparation and docking occurs efficiently. For most gynecologic oncologic procedures, the patient is placed in the lithotomy position with legs in adjustable stirrups, and is secured to the bed with gel pads or shoulder bolsters to prevent movement after placement into steep Trendelenburg position.
The procedure is initiated in a similar fashion to traditional laparoscopy by insufflating the abdomen with carbon dioxide gas, using a Veress needle, blunt trocar entry, or by inserting a trocar under direct vision via an open laparoscopic technique, or using transparent trocars and a camera. The exact location for port placement will depend on the planned procedure. A general position of trocars needed to complete a hysterectomy or radical hysterectomy with pelvic and para-aortic lymphadenectomy is shown in Figure 22.8A. This includes a 12-mm port for the camera (Fig. 22.8B) and three 8-mm ports for the robotic instrument arms (Fig. 22.8C). Most surgeons will also place one 12-mm accessory port for a bedside assistant, who can use suction, pass sutures or surgical clips, or remove specimens.
Docking technique and trocar placement are particularly important components of robotic surgery, because the arms are set in place after docking. Although the original technique for hysterectomy involved docking the Patient-side Cart between the patient’s legs (front-docking), many surgeons now use side docking or parallel docking because this permits access to the vagina for uterine manipulation or the removal of specimens (22). Side docking and parallel docking can be performed from either the right or left side of the patient depending on which side the surgeon would prefer to swing the third arm (Fig. 22.9A,B). One challenge for robotic gynecologic oncologic surgery has been accessing multiple quadrants to permit resection of more advanced disease, mainly in the upper abdomen. Surgeons have found that by undocking, rotating the patient and/or the patient cart during the surgery and redocking over the patient’s shoulder (Fig. 22.9C), it is possible to access the upper abdominal quadrants for dissection of organs such as the diaphragm, liver, and spleen.
Figure 22.8 A: Typical trocar placement sites for robotic hysterectomy and lymphadenectomy: Camera can be placed at either blue markings, avoiding the falciform ligament. The assistant port moves in accordance. Robotic trocar sites are indicated by numbers. For left-sided docking, the port site locations are reversed. B: 12-mm and accessory trocars, C: 8-mm robotic trocars.
Figure 22.9 Docking Techniques: A: Left-Side docking, B: Right-Side docking, C: Over shoulder/head docking for upper abdominal resection.
After the instrument arms have been docked to the trocars, the camera and surgical instruments can be introduced through the trocars and secured to the robotic arms. The camera arm can be fitted with either an 8.5-mm or 12-mm high-definition endoscope (0° or 30°). A variety of instruments have been developed including those for grasping, dissecting, and suturing (examples shown in Fig. 22.7). In addition to these standard instruments, a variety of specialty instruments have been adapted for use with the robotic platform, such as Clip Appliers, Vessel Sealers, Harmonic ACE, PK Dissecting Forceps, and bowel stapling devices. Many surgeons will also use a uterine manipulator to assist with positioning of the uterus during the dissection, and to facilitate the colpotomy. Commonly used uterine manipulators include the RUMI (Cooper Surgical, Turnbull, CT, USA), V-CARE (ConMed, Utica, NY, USA), and HOHL manipulator (Karl Storz, Tuttlingen, GE). Although some have hypothesized that placing a manipulation tool into the uterine cavity could result in dissemination of cancer cells into the peritoneal cavity, published data indicate this has no clinical significance (40–42). Alternatives without intrauterine components have been proposed to alleviate this potential issue (22).
Robotic Applications in Gynecologic Oncology
Robotic surgery has been used to treat uterine cancer, cervical cancer, and ovarian cancer. Robotic techniques have been described for most components of gynecologic cancer surgery, including extrafascial hysterectomy, radical hysterectomy, radical trachelectomy, staging with pelvic and para-aortic lymphadenectomy, debulking procedures, and pelvic exenteration. Although there have been numerous publications demonstrating the feasibility and advantages of robotic techniques, there have been no randomized trials comparing robotic surgery with either laparotomy or laparoscopy. Most publications compare a series of robotic cases to a contemporary cohort of cases done by laparoscopy and/or laparotomy, or to a group of historical controls. Despite being very encouraging, interpreting these studies can be difficult, as they are limited by weaknesses such as selection bias, reporting bias, and learning curve differences.
Table 22.1 Studies of Robotics for Unselected Patients with Endometrial Cancer
Endometrial Cancer
Most of the first reports of robotic gynecologic oncologic surgery involved hysterectomy and staging of endometrial cancer. Numerous series have reported the success of robotics for endometrial cancer staging, and have shown decreased morbidity compared to laparotomy, with low rates of conversion (Table 22.1). In addition, there have been two systematic reviews of the safety and effectiveness of robotic surgery for endometrial cancer (43,44). Both of these reviews have demonstrated that compared to laparotomy, robotic or laparoscopic surgery for endometrial cancer is associated with increased operative time, but significantly decreased blood loss, need for transfusion, hospital stay, and postoperative complications. Although the accuracy, reproducibility, and comparison between pathology laboratories is questionable, pelvic and para-aortic lymph node counts with robotics is at least equivalent and possibly better than laparotomy. A comparison between robotics and standard laparoscopy has demonstrated lower postoperative pain and pain medication requirements when robotic surgery has been used for endometrial cancer staging (45). One important observation from robotic studies is the low rate of conversion to laparotomy, which ranges from 1–12%, even among subgroups known to be technically challenging for MIS approaches, such as the obese and elderly populations (Table 22.1).
Robotics in Obese Patients
Endometrial cancer frequently affects women with high BMI, who are also at increased surgical risk as a result of associated comorbidities such as diabetes, hypertension, and atherosclerosis. Although the elevated surgical risk in these patients makes them ideal candidates for MIS, conversion rates with laparoscopy have been high in these women. In the LAP2 study, the overall conversion rate from laparoscopy to laparotomy was 25%, but increased to 57% in patients with BMI > 40 (17). Robotic surgery has been particularly useful in facilitating MIS for women with increased BMI (Table 22.2). Lau et al. compared endometrial cancer staging procedures for women with BMI < 30, 30 to 39, and 40 to 59 and demonstrated that, despite more frequent comorbidities in the more obese subgroup, there were no significant differences in surgical console time, major postoperative complications, overall wound complications, or length of hospitalization (25). The conversion rate was only 10% in those with BMI 40 to 59, and all the conversions were by mini laparotomy to remove an enlarged uterus that could not be removed intact through the vagina. When compared to laparotomy, the use of robotic endometrial cancer staging for women with an elevated BMI is associated with fewer complications, shorter hospital stay, and equivalent lymph node counts, with a conversion rate around 15% (24,35). A retrospective comparison of robotic and laparoscopic endometrial cancer surgery for obese women showed shorter operative times, less blood loss, increased lymph node counts, and shorter hospital stay with the robotic approach (57).
Table 22.2 Studies of Robotics for Obese and Elderly Patients with Endometrial Cancer
Robotics in Elderly Patients
Another group who may particularly benefit from robotic surgery is elderly women (Table 22.2). The endometrial cancers diagnosed in elderly women are frequently of high-grade aggressive histology, and optimal surgery may require extensive lymphadenectomy in patients with additional complex comorbidities. In the LAP2 study, the conversion rate increased by 30% for every additional decade of age (13). In contrast, a comparison of robotic surgery for women younger than 70 years of age to women greater than 70 demonstrated similar operative times, blood loss, and overall perioperative morbidity, with a conversion rate of only 5% in women older than 70 years (36) and 3.7% in women over the age of 80 (58). Overall, robotic surgery can help facilitate thorough surgery in fragile, elderly patients with aggressive endometrial cancers.
In summary, although randomized clinical trials are lacking, multiple studies have shown decreased morbidity compared to laparotomy, with low rates of conversion. Two studies have shown equivalent recurrence-free survival (32,59), and one has shown equivalent 5-year survival following robotic surgery for endometrial cancer (60).
Cervical Cancer
Radical Hysterectomy
Compared to endometrial cancer, the transition to MIS for treatment of cervical cancer has been slower, which is likely related to the technical complexity and steep learning curve of conventional laparoscopic radical hysterectomy. However, since the introduction of robotics, there has been a rapid increase in the use of MIS for radical hysterectomy and treatment of cervical cancer. Based on the small retrospective series published, it appears that robotic radical hysterectomy is feasible, associated with less morbidity than laparotomy, and seems to be easier to implement than traditional laparoscopy (Table 22.3). A systematic review comparing 327 robotic, 1,339 laparoscopic, and 1,552 open radical hysterectomies showed that the MIS approaches had significantly less blood loss, transfusion requirements, and postoperative infectious morbidity, with similar oncologic outcomes (12). Robotic surgery was also associated with less postoperative narcotic pain medication, quicker resumption of regular diet, and shorter hospitalization (61).
The pathologic features of radical hysterectomy specimens are similar between robotic, laparoscopy, and laparotomy, including parametrial and vaginal resection margin lengths, lymph node counts, and risk of positive surgical margins (64,68). Thus, there is no difference in the proportion of patients requiring adjuvant therapy after robotic radical hysterectomy. Based on available data, progression-free and overall survival after robotic radical hysterectomy for cervical cancer appears to be at least equivalent to laparotomy (66). A multicenter Phase III randomized clinical trial is currently accruing patients, comparing MIS radical hysterectomy (total laparoscopic or robotic) with open radical hysterectomy for Stage IA1 to IB1 cervical cancer (NCT00614211) with an estimated completion date in 2017 (74).
In summary, evidence from available retrospective series for cervical cancer reveals decreased morbidity with robotics and similar recurrence rates, progression-free survival, and disease-specific survival, when compared to open laparotomy (66).
Radical Trachelectomy
Women with cervical cancer are frequently diagnosed in the reproductive age group, so fertility preservation can be an important issue. The use of radical trachelectomy for early cervical cancer has been shown to have similar efficacy and safety compared with radical hysterectomy (75). Plante et al. showed that vaginal radical trachelectomy with laparoscopic lymphadenectomy is oncologically safe for women with cervical tumors less than 2 cm in size, and has good fertility and obstetrical outcomes (76). The feasibility of robotic radical trachelectomy with sparing of the main branches of the uterine artery was described in 2008 (77), and the possible benefits of the robotic technique have been elucidated in several small series (78–81). Persson et al. compared robotic radical trachelectomy in 13 patients with vaginal radical trachelectomy in 12 patients and found that the cervical length was equal, but there was a more accurate placement of the cervical cerclage with the robotic approach (78). Nick et al. reported on 37 patients who underwent attempted radical trachelectomy (12 robotic and 25 laparotomy) and found that 5 required conversion to complete hysterectomy as a result of close endocervical margins (4 robotic, 1 open). The robotic cases had less blood loss and decreased hospitalization, with no difference in surgical time or serious morbidity (82).
Table 22.3 Studies of Robotic Radical Hysterectomy for Cervical Cancer
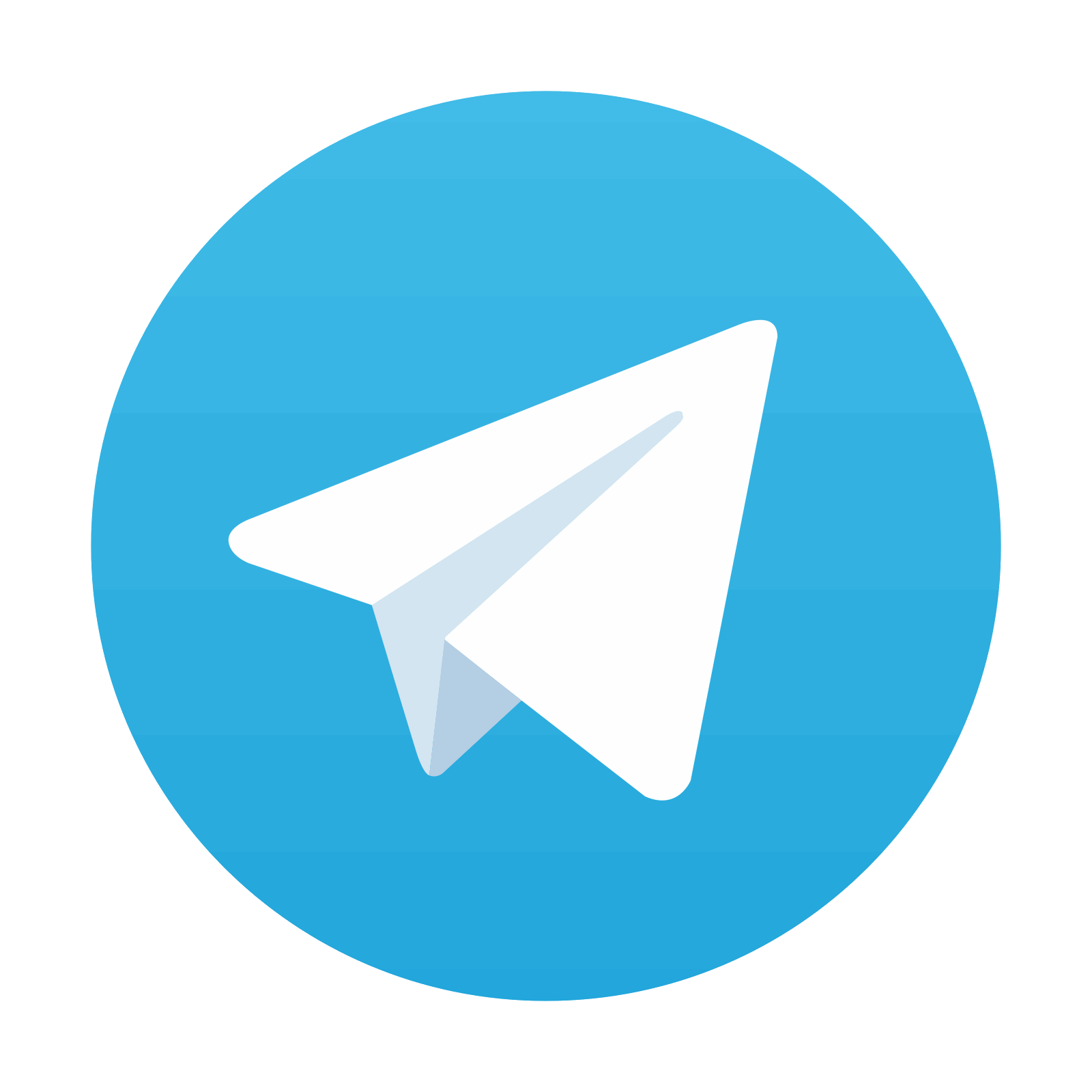
Stay updated, free articles. Join our Telegram channel

Full access? Get Clinical Tree
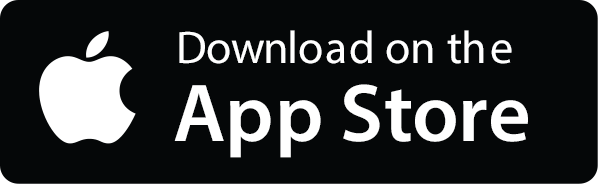
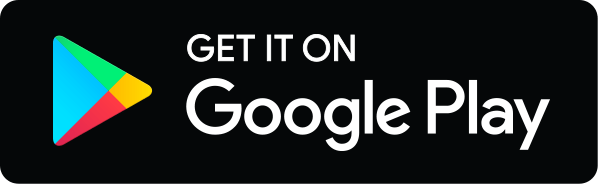