COMPLICATIONS OF CANCER AND ITS TREATMENT
The complications of cancer and its treatment represent a broad and diverse group of disorders that are covered in detail throughout this textbook. In the context of cancer rehabilitation, several specific disorders are of interest because they can heavily impact the safety and effectiveness of rehabilitative efforts. It is imperative for the clinician to fully understand that there are no absolutes in oncology and that every decision made with or on behalf of a patient has an implicit cost-benefit analysis underlying it. This is as true for the physical or occupational therapist deciding if it is safe to ambulate a patient as it is for the neurosurgeon deciding whether to attempt resection of metastatic disease compressing the spinal cord. Poor outcomes are common in cancer and can happen at any time, often unexpectedly and occasionally dramatically. The challenge for the rehabilitation specialist is to maximize function and quality of life while minimizing, to the best of their ability and knowledge, any potential adverse outcomes.
Direct Effects of Cancer
The direct effects of cancer are often obvious, with the clinical impact on function being dependent largely on the location and extent of disease. Examples are a large lung mass compromising pulmonary function and, subsequently, endurance and stamina, or an epidural metastasis to the spine compressing the spinal cord causing weakness and pain. A working understanding of several of the direct complications of cancer including its effects on the central and peripheral nervous system and on bony integrity, are of great importance in cancer rehabilitation and will be discussed in detail in subsequent sections.
Paraneoplastic Effects
Paraneoplastic syndromes (PNS) are rare disorders (<1% of cancer patients) that cause tissue or organ damage via remote effects of a primary or metastatic cancer.6 Most PNS occur because the tumor secretes a substance that mimics normal hormones or that interferes with circulating proteins. Systemic paraneoplastic disorders include cancer cachexia, hypercalcemia, Cushing syndrome, and Trousseaus syndrome.7–10 A number of PNS affect the central nervous system (CNS) (limbic encephalitis, encephalomyelitis, cerebellar degeneration, opsoclonus-myoclonus, optic neuropathy/retinopathy, brain stem encephalitis, stiff person syndrome, motor neuron disease, necrotizing myopathy) and peripheral nervous system (sensory neuronopathy, acute sensorimotor neuropathy, chronic sensorimotor neuropathy, neuromyotonia, chronic gastrointestinal pseudo-obstruction/autonomic neuropathy, Lambert-Eaton myasthenic syndrome, myasthenia gravis, inflammatory myopathy) with potentially devastating impacts on function and quality of life.11 These disorders, although relatively rare, represent a significant therapeutic challenge to the rehabilitation specialist.
Chemotherapy
Chemotherapy is a cornerstone of cancer treatment for many malignancies and may be given either with intent to cure or to prolong life. The myriad complications of the ever growing number of agents are discussed at length in other chapters. The impact of these complications on function and quality of life depends on the specific derangement or complications induced by the agent. Anemia, neutropenia, thrombocytopenia, myopathy, cardiomyopathy, neuropathy, contracture, fatigue, thromboembolism, nausea, and edema are just a few of the common disorders resulting from chemotherapy that can have a major impact on rehabilitation efforts. Several of these complications and their specific implications for rehabilitation will be discussed in subsequent sections.
Radiotherapy
Approximately 50% of cancer patients will be treated with radiation therapy at some point during the course of their disease, and radiation may play a critical role in as many as 25% of cancer cures.12 Despite the important beneficial effects of radiation in cancer care, its adverse effects on normal tissue are a major source of disability for both patients with active cancer and cancer survivors.13 Evaluations and treatment of the long-term complications of radiation are extremely important components of the clinical activities of the cancer rehabilitation specialist and will be discussed in detail in the following paragraphs.
Surgery
Postsurgical impairments depend not only on the anatomic site and extent of the procedure, but also on any medical comorbidity the patient has. Surgical complications are common in the cancer setting and impact heavily on overall outcome as well as rehabilitation needs. Rehabilitation is often instrumental in returning function and quality of life to patients following surgery. Efforts may be directed at the restoration of functional disorders ranging from diminished stamina following prolonged bed rest to restoration of a limb following hemipelvectomy. The postsurgical rehabilitation needs of any given patient are unique.
Thromboembolism
There is considerable and high quality data available on the prevention and treatment of thromboembolism, including comprehensive guidelines specific to the cancer and rehabilitation settings.14,15 There is comparatively little data concerning the safe and effective rehabilitation of patients with thromboembolism. The dogma from the not too distant past was to immobilize patients with deep vein thrombosis (DVT) and range of motion (ROM) exercises for anywhere from 2 to 10 days presumably to allow the clot to mature by the formation of fibrin cross-links and to thereby decrease the risk of dislodging the clot to form a pulmonary embolism (PE).16 This commonly followed, long-held, and nonevidence-based recommendation was ultimately supported by a limited study suggesting that immobilization of the affected limb for at least 48 to 72 hours while the patient is anticoagulated was justified and prudent to prevent PE.17 Partsch16 describes a survey of 31 international experts who gave the recommendation to immobilize patients for 4 to 5 days prior to intensifying physical activity. The development of low–molecular-weight heparin (LMWH) prompted a reevaluation of the bed rest recommendation as patients with acute DVT treated with LMWH injections at home did at least as well in terms of recurrent thromboembolism and bleeding as did patients treated in the hospital with unfractionated heparin delivered intravenously.18,19 Although not directly applicable to patients in either the inpatient or outpatient rehabilitation setting, the realization that no increase in morbidity or mortality was seen in patients with acute DVT treated with LMWH injections served as justification for liberalization of the bed rest recommendation that had inhibited rehabilitation efforts, particularly on inpatient rehabilitation units.
Although the question of when a patient with lower extremity DVT can begin to ambulate has not been answered with the same evidence-based rigor as other questions concerning thromboembolism, there is a growing body of clinical trials on the topic that support the safety and potential benefits of early mobilization.16,20–25 Several reviews and one meta-analysis are also available and support the safety and benefits of early mobilization following both DVT and PE while dispelling the recommendation for bed rest as part of the early management of thromboembolism.16,26–31
Upper extremity DVT (UEDVT) represents about 10% of DVTs and can be idiopathic but is more often associated with central venous catheter use, pacemakers, or cancer.32 The relative incidence of UEDVT may be rising due to increased use of central venous catheters and is associated with PE in up to one-third of patients. Other conditions associated with UEDVT include postthrombotic syndrome, superior vena cava syndrome, septic thrombophlebitis, thoracic duct obstruction, and brachial plexopathy.33 Despite the high incidence of conversion of UEDVT to PE, no studies are available to address the multitude of questions concerning the rehabilitation of patients with UEDVT. This lack of data concerns such basic issues as the safe timing and type of exercise that can be safely done following the diagnosis of UEDVT as well as if and when decongestive therapy (massage, wrapping, pumps) for upper extremity swelling disorders such as lymphedema can be performed. Lack of even basic data on these matters represents a major shortcoming in our overall knowledge on the topic of cancer rehabilitation and challenges our ability to guide risk–benefit analyses for patients with UEDVT who will likely benefit from physical therapy (PT), occupational therapy (OT), or decongestive therapy.
Although not strictly evidence based due to major shortcomings in the literature concerning the rehabilitation of patients with thromboembolism, the guidance developed by the author and used at the Memorial Sloan-Kettering Cancer Center (MSKCC) is presented in Table 152.1. It is critical to remember that each patient and situation is unique and that these recommendations are only starting points that should not supersede clinical judgment and assessment of the risks and benefits to the patient of engaging in a given activity.
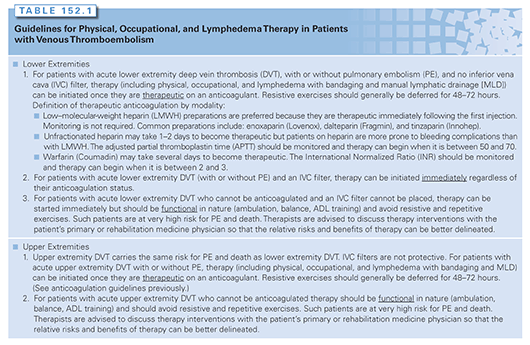
Other Medical Disorders
The evidence base for the safe and effective rehabilitation of patients with a variety of general medical disorders is also limited. Despite this limitation, guidance with widespread clinical use has evolved on such issues as thrombocytopenia, anemia, neutropenia, cardiac dysfunction, pulmonary dysfunction, and electrolyte abnormalities. In general, these recommendations applied to rehabilitation are based on indirect evidence from the medical literature. Exercise precautions for cancer patients with a variety of disorders are presented in Table 152.2. Again, the potential risks and benefits of pursuing any course of therapy must be carefully weighed.
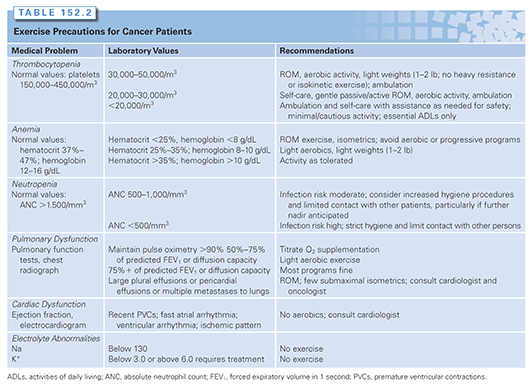
NEUROMUSCULAR COMPLICATIONS OF CANCER AND CANCER TREATMENT
The treatment of neuromuscular complications of cancer is a major component of the services offered by both the rehabilitation medicine physician and the physical and occupational therapists. Such disorders include those of the brain, spinal cord, nerve roots, plexus, peripheral nerve, and muscle.34
Brain Dysfunction
The brain, along with the myriad cognitive and physical functions that it serves, is often compromised in the cancer setting. Such dysfunction can result from direct effects of the tumor, radiation, radiation necrosis, paraneoplastic effects, or other medical disorders.
Brain tumors vary widely in aggressiveness and prognosis. Even a benign or relatively low-grade lesion may have severe functional consequences depending on its location. It is unclear to what extent tumor type or location impacts rehabilitation prognosis; however, some studies suggest a tendency for better gains in meningioma patients and in those with left hemispheric lesions.35,36
The most common neurologic deficits in brain tumor patients undergoing acute rehabilitation include impaired cognition (80%), weakness (78%), and visual–perceptual impairment (53%) with most patients having multiple impairments.37 Although overall prognosis may be a factor, most rehabilitation disposition decisions are guided by the patient’s neurologic status, clinical course, and activity tolerance. A patient whose neurologic status is actively worsening will likely not benefit from intensive rehabilitation.
Specific rehabilitation interventions depend on the combination of deficits and are generally similar to measures used for patients with other etiologies of brain disorders, such as traumatic brain injury or stroke. Rehabilitation efforts are directed toward such goals as the improvement of bed mobility, transfers, self-care, ambulation, and if necessary, wheelchair training. Strategies are employed to promote functional cognition, clear communication, bowel and bladder continence, safe swallowing, adequate nutritional intake, optimal sensory input (including vision and hearing), and restorative sleep. Attention must be paid to the prevention of complications of impaired mobility such as skin breakdown or DVT. Similarly, complications related to the primary tumor and other comorbidities such as pain, neurologic decline, seizure, and depression should be evaluated and treated. The hemiplegic patient will generally benefit from gait training with an assistive device and a brace such as an ankle–foot orthosis (AFO) when appropriate. Proper arm positioning, with support of the shoulder to prevent pain in a flaccid or spastic limb, should be used. A variety of adaptive equipment are available for individuals who must perform tasks one handed, such as reachers, sock donners, and elastic shoelaces.
Cognitive strategies often rely on compensations such as keeping a regular routine and maintaining a journal or memory notebook. The patient’s insight into cognitive deficits may be impaired, and education regarding these deficits can allow the patient to more effectively compensate and allow family, friends, and caregivers to have appropriate expectations. In some cases, especially those in which cognitive status is anticipated to remain fairly stable, in which functional expectations are high, or where conflict or confusion exists about the patient’s abilities, neuropsychological testing helps to more fully define the extent of cognitive deficits and also to elucidate cognitive strengths. For example, determining whether an individual learns best with auditory, written, or nonverbal presentations can facilitate the most efficient compensation for cognitive deficits. Pharmacologic and nonpharmacologic therapies to address pain, spasticity, mood, bowel/bladder, and sleep/wake issues are often indicated.
Functional recovery is similar in patients with brain tumors as compared with acute stroke.38 Better functional improvement is a significant predictor of longer survival in patients with brain metastases and glioblastoma multiforme (GBM). The literature suggests that the length of stay for inpatient rehabilitation for brain tumor patients is generally shorter than patients with other brain disorders such as traumatic brain injury and stroke.35,36 This may relate to better initial functional status; other possible contributing factors include fewer behavioral sequelae, better social supports, and expedited discharge planning due to prognostic factors.39 In one study, although functional status improved during rehabilitation, quality-of-life scores did not improve until the discharge home. The functional independence measure (FIM) and the disability rating scale are more sensitive than the Karnofsky performance status scale in detecting changes in functional status.40
Spinal Cord Dysfunction
Symptomatic spinal cord injury (SCI) due to spinal metastasis occurs in up to 5% of all cancer patients.41 Patients with cancer can develop SCI as a direct result of their cancer, its treatment (e.g., radiation), and other preexisting or acquired conditions. SCI in the cancer setting can present a significant decision-making and management challenge.42 This challenge is compounded as cancer-related SCI most often occurs as a late complication when poor overall prognosis and declining medical status can significantly and adversely impact rehabilitation interventions and functional prognosis.
Life expectancy following spinal surgery varies with cancer type. One study demonstrated a median survival of 7.7 months for all cancer types combined, with colon cancer patients surviving only 3.3 months and lung cancer patients only 3.6 months. Sarcoma, prostate, renal cell, and breast carcinoma did better, with median survival ranging from 7.8 to 15.4 months.43 A retrospective evaluation of patients with neoplastic SCI admitted for inpatient rehabilitation demonstrated a combined median overall survival time of only 4.1 months from the date of rehabilitation admission to death. Patients with gastrointestinal cancer had an even poorer prognosis with a median survival of only 0.6 months.44 The phase of cancer treatment can have a profound impact on survival and prognosis for functional recovery. A patient with metastatic prostate or breast cancer, for instance, whose initial presentation is with SCI and has not received systemic therapy, can be expected to have a much better prognosis for survival and functional restoration than a patient who has undergone and failed multiple treatment options.
SCI in the cancer setting can result directly from the cancer (epidural, leptomeningeal, or intramedullary tumor; Fig. 152.2), indirectly from the cancer (paraneoplastic phenomenon), or from treatment of the cancer (radiation, chemotherapy, surgery). Other causes of SCI in the cancer setting may include infection (meningitis, epidural abscess, discitis/osteomyelitis), vascular disorders (hemorrhage, infarct), degenerative disorders (cervical spinal stenosis), and even metabolic disorders (osteoporotic compression fractures).
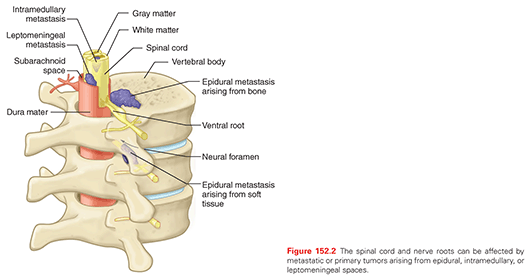
The vertebral body is the most common site of metastasis within the spine, largely reflecting its volume relative to other spinal structures. Metastatic tumors of the spine are more common than primary spine tumors. Cancers that commonly metastasize to the spine are those of the breast, lungs, prostate, and kidneys.45 Epidural tumors are the most common cause of SCI in cancer and can arise from the vertebra, enter the spinal canal through the neural foramen or be deposited hematogenously in the epidural space. Leptomeningeal metastases occur in 3% to 8% of all cancer patients and can be associated with significant neurologic dysfunction. The most common tumors that metastasize to the leptomeninges include leukemia, lymphoma, melanoma, and breast and lung cancers.46 Intramedullary tumors are the rarest of the tumors that affect the CNS but can produce profound neurologic deficits that vary depending on the location and pathology of the tumor.
SCI from epidural-based tumors generally affects all spinal tracts either completely or to varying degrees. Asymmetric compression does occur and can have unpredictable patterns in early compression but generally progresses to affect all components of the spinal cord. Clinically, such patients present with weakness, bowel and bladder dysfunction, and multimodal sensory loss including light touch, pain, vibration, and proprioception. Pain, if present, is usually local.
The level of injury significantly affects the potential for functional recovery. It should be noted that the degree of functional recovery anticipated in a patient with a nonmalignant cause of SCI (such as trauma from a motor vehicle accident) is generally greater than that anticipated in malignant SCI. This is because patients with cancer tend to develop SCI later in their disease and usually progress in their cancer and other medical comorbidities.
The care of patients with acute SCI includes the maintenance of ventilation in those with high levels of injury. It also includes the treatment of pain, autonomic dysregulation, and bowel and bladder dysfunction. Patients are at risk of developing DVT, PE, pulmonary and urinary tract infections, fecal impaction, decubiti, immobilization hypocalcemia, spasticity, contractures, and osteoporosis. A comprehensive treatment plan includes DVT and PE prophylaxis, spirometry, and chest physiotherapy; the initiation of intermittent urinary catheterization every 4 to 6 hours when urine volumes are less than 2,000 mL per day; the initiation of a bowel program; turning every 2 hours; and use of high-airflow mattresses, use of heel protectors, and daily PT and ROM.15,47
The use of LMWH is currently recommended for DVT and PE prophylaxis during the acute management phase of SCI.15 The independent use of low-dose unfractionated heparin, elastic compression stockings, and intermittent pneumatic compression devices is not recommended. When used alone, they appear to be relatively ineffective. It is recommended that LMWH be continued or converted to full-dose oral anticoagulation for a minimum of 3 months or at least until completion of the rehabilitation phase.48
Patients with injuries above the T6 level are at risk of developing autonomic dysreflexia, which is defined as an increase in blood pressure of greater than 20 mm Hg above baseline. It results from stimulation of the splanchnic division of the sympathetic nervous system by a noxious stimulus below the level of the lesion and causes the sympathetic responses of vasoconstriction and hypertension. Autonomic dysreflexia constitutes a medical emergency and must be evaluated and treated immediately.
The patient’s ability to participate in rehabilitation therapies while undergoing further treatments must be considered when an acute or subacute inpatient rehabilitation program is sought. Rehabilitation programs for those with SCI due to spinal tumors have been shown to improve mobility and self-care.49 Research indicates that clinical and functional status is a valuable prognostic factor for survival of those with SCI due to metastatic tumor disease.50 The rehabilitation program should be started as early as possible and should be of short duration.44 Thus, the selection of patients who have the potential to improve function may improve survivability as well as quality of life and ability to perform activities of daily living (ADL) and mobility.
Peripheral Nervous System Dysfunction
Radiculopathy can result from a variety of disorders that affect one or more nerve roots and is a very common cause of pain and disability in the cancer setting. Radiculopathy can occur from compressive or less common noncompressive etiologies. Although the incidence of radiculopathy in cancer patients and survivors is unknown, it is likely that most nerve root pathology results from the same degenerative disorders, largely spondyloarthropathies, that affect the general population with a prevalence of 3% to 5%.51 One large study on the epidemiology of cervical radiculopathy in a population of patients in Rochester, Minnesota placed the average annual age-adjusted incidence at 83.2 per 100,000.52 Degenerative causes of radiculopathy can be discogenic (e.g., herniated nucleus pulposus), from hypertrophy of ligaments and other soft tissues (e.g., ligamentum flavum), or from excess bone formation (e.g., osteoarthritis). The narrowing of the spinal canal or neural foramen with subsequent compression of neural and vascular structures resulting from one or more degenerative processes is called spinal stenosis. Lumbar spinal stenosis is estimated to affect 1 in 1,000 persons older than 65 years of age.53
Compressive radiculopathy resulting directly from cancer most commonly results from an epidural tumor that can arise from the vertebral body, paravertebral structures, or the epidural space itself.54 Intramedullary tumors, including leptomeningeal and intramedullary disease, can also cause compressive radiculopathy. Of these, compression by leptomeningeal disease is far more common affecting 5% to 8% of patients with cancer.54
Noncompressive causes of radiculopathy include radiation, chemotherapy, and paraneoplastic syndromes as well as disorders such as acute idiopathic demyelinating polyradiculoneuropathy (AIDP), a subtype of Guillain-Barré syndrome (GBS), chronic idiopathic demyelinating polyradiculoneuropathy (CIDP), amyloidosis, sarcoidosis, diabetes mellitus, and rheumatologic disorders (Fig. 152.3).1 Herpes zoster is also a common cause of radiculopathy in the cancer setting causing a dermatomal vesicular rash known as shingles.55 Herpes zoster causing dermatomal pain without a rash is termed zoster sine herpete.56 An eruption of Herpes zoster is related to immunosuppression from cancer, steroids, and chemotherapy with 1% to 2% of cancer patients having at least one infection during the course of their disease.57 Polyradiculopathy from radiation may mimic a leptomeningeal tumor because the cauda equina may demonstrate enhancement on magnetic resonance imaging (MRI; see Fig. 152.3).58 Although relatively uncommon, radiculopathy from radiation has been reported as sequelae of testicular cancer, vertebral metastases, and lymphoma.59–64 In the author’s clinical experience, radiation-induced radiculopathy is most commonly seen in Hodgkin lymphoma (HL) survivors who have received mantle field (MF) or periaortic radiation and head and neck cancer patients often in conjunction with plexopathy. Additionally, in rare instances, radiculoplexopathy can result from single fraction (2,400 cGy) radiation. Neurotoxic chemotherapy can also adversely affect the nerve root as part of a more generalized neuropathy. Patients with preexisting radiculopathy from a degenerative or other cause may be particularly predisposed to the adverse effects of neurotoxic chemotherapy.65,66 Subacute motor neuronopathy is a paraneoplastic disorder described in patients with HL, non-Hodgkin lymphoma, and thymoma as a subacute lower motor neuron syndrome that primarily involves the lower extremities without pain, significant sensory loss, or upper motor neuron findings. Secondary thinning of the ventral nerve roots and widespread patch segmental demyelination of spinal nerve roots and the brachial and lumbosacral plexus can be seen histologically in addition to patchy degeneration and loss of anterior horn cells and occasional inflammatory infiltrates.67 Non–cancer-related causes of noncompressive radiculopathy including diabetes, rheumatologic disorders, and idiopathic radiculoneuropathies such as GBS and CIDP not uncommon in the general population should be included in the differential diagnosis when evaluating cancer patients and survivors with signs and symptoms suggestive of radiculopathy.
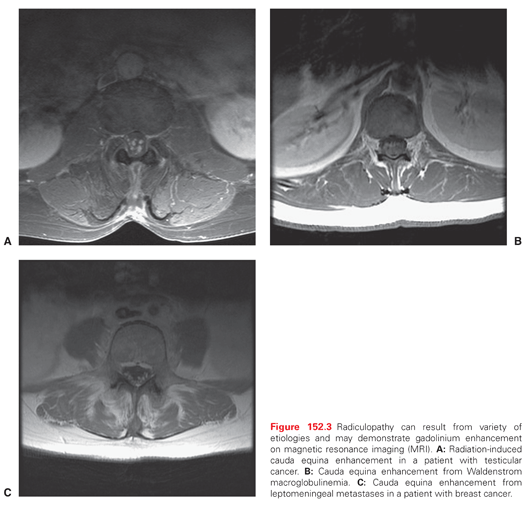
Plexopathy affects as many as 1% of cancer patients.68 The cervical, brachial, or lumbosacral plexus can be involved and the etiology can be neoplastic or radiation induced as well as idiopathic (idiopathic brachial neuritis, CIDP, GBS), diabetic (diabetic radiculoplexus neuropathy), or traumatic (surgery). Malignancy most commonly affects the brachial plexus followed by the lumbosacral plexus and, least commonly, the cervical plexus.69 Benign tumors of the plexus are usually reported to be more common than malignant ones.70–72 Radiation plexopathy is a common component of the radiation fibrosis syndrome (RFS) that is characterized by insidious fibrosis with axonal demyelination and, ultimately, axonal loss. Radiation plexopathy is commonly seen with radiculopathy. Radiation to the plexus can rarely cause a mild reversible syndrome but is more commonly a delayed and progressive one.73 The risk of brachial plexopathy in the adjuvant breast cancer setting is <1% when the dose per fraction is 2 Gy and the total dose is 50 Gy or the dose per fraction is 2.2 Gy to 2.5 Gy and the total dose is 34 Gy to 40 Gy. However, if the dose per fraction is 2.2 Gy to 4.58 Gy and the total dose is 43.5 Gy to 60 Gy, the brachial plexopathy risk increases to 1.7% to 73%.74 Delayed radiation-induced brachial plexopathy usually occurs after a latent interval that can vary from a few months to several years with a peak onset of neurologic symptoms occurring between 2 and 4 years after radiation.75 The upper plexus is commonly affected preferentially in HL survivors treated with MF radiation and head and neck cancer patients possibly due to the longer course of the upper trunk and lateral cord of the brachial plexus through the neck as well as the pyramidal shape of the thorax providing less protective tissue. Additionally, the upper plexus may represent the most caudal extent of radiation delivered for some head and neck cancer patients.
Differentiating radiation induced from malignant plexopathy is a diagnostic challenge and both etiologies may be present together. Imaging with gadolinium-enhanced MRI is the diagnostic test of choice when excluding a tumor of the brachial plexus.76 Positron-emission tomography/computed tomography (PET-CT) has a role to play in selected cases. Malignant etiologies of plexopathy are generally more painful than radiation-induced plexopathy, although either can manifest severe pain.77 Myokymia on needle electromyography is suggestive of a radiation-induced etiology but does not exclude a malignant component.78
Idiopathic brachial neuritis (IBN), also known as neuralgic amyotrophy, Parsonage-Turner syndrome, and other synonyms, have an incidence of approximately 2 to 3 per 100,000 person-years.79 The disorder can be idiopathic or hereditary. The idiopathic form is often precipitated by antecedent events such as infection, immunization, strenuous exercise, surgery, and a variety of other causes.79 Clinically, IBN is classically characterized by severe and acute burning pain in the shoulder and arm that may last for several days or weeks and is followed by muscle weakness, atrophy, and sensory loss. IBN may go unrecognized in cancer patients if only the obvious cancer and treatment-related causes of brachial plexopathy are considered. Similarly, diabetic radiculoplexus neuropathies can affect the cervical, thoracic, and lumbosacral nerve roots as well as the brachial and lumbosacral plexus. Perhaps the best known of these disorders is diabetic lumbosacral radiculoplexus neuropathy (DLRPN), also known as diabetic amyotrophy among other synonyms. DLRPN usually presents clinically with unilateral acute to subacute and severe lower extremity pain in the thigh or leg that may spread to the entire extremity.80 Like IBN, DLRPN may go unrecognized in the cancer setting if only neoplastic or treatment-related etiologies are considered and can occur even with relatively low blood glucose levels as might be seen with steroid-induced hyperglycemia.
Neuropathy is any condition arising from the damage and dysfunction of the peripheral nerves including the motor, sensory, and autonomic nerves that connect the CNS (brain and spinal cord) to the rest of the body.81 The peripheral nerve includes the nerve root and plexus as well as the peripheral nerve proper. Peripheral neuropathy is common in the cancer setting as a result of neurotoxic chemotherapy or radiation as well as from direct and paraneoplastic effects of cancer. Neuropathy is also very common in the general population with diverse etiologies that can affect cancer patients even if unrelated to malignancy or its treatment. Such etiologies include toxic or metabolic derangements (diabetes mellitus, vitamin B-12 deficiency, alcoholism), inherited disorders (Charcot-Marie-Tooth), paraprotein disorders (monoclonal gammopathy of unknown significance [MGUS]), critical illness neuropathy, and idiopathic disorders such as CIDP or GBS just to name a few.82 The clinical manifestations of neuropathy may include any combination of weakness, sensory deficits, pain, and autonomic dysfunction with resultant gait and other functional deficits. Often, multiple peripheral nervous system disorders such as radiculopathy and neuropathy coexist, compounding the clinical features of each disorder. It is important to remember that, as described previously, any preexisting pathology at any part of the neuron (nerve root, plexus, or peripheral nerve), even if asymptomatic, may predispose the patient to the development of weakness, paresthesias, pain, and other signs and symptoms commonly described as neuropathy when challenged with a neurotoxic chemotherapy or another insult to the peripheral nervous system. This phenomenon is termed neuronal predisposition.65,66
Peripheral neuropathy is one of the most common chronic complications of cancer and its treatment. It may be caused by a variety of chemotherapeutic agents including the taxanes (paclitaxel, docetaxel), the vinca alkaloids (vincristine, vinorelbine, vinblastine), the platinum analogs (cisplatin, carboplatin, oxaliplatin), bortezomib, thalidomide, and others.65,83–85 The clinical, pathophysiologic, and electrodiagnostic features of the various neurotoxic chemotherapeutics vary significantly. The taxanes and vinca alkaloids are tubulin inhibitors and cause a predominately length-dependent motor and sensory axonal polyneuropathy in a distal symmetric distribution.83 Clinically, this causes paresthesias, pain, sensory ataxia, and potentially, weakness during and immediately following treatment that usually improves when the treatment is stopped. The platinum analogs, however, damage the cell body of the sensory nerves in the dorsal root ganglion, and at higher doses can damage the anterior horn cell within the spinal cord. It is the accumulation of the platinum analogs within the neuron cell body with subsequent DNA damage and altered cellular activities that underlie the toxicity.86 The clinical manifestations include severe sensory deficits including sensory ataxia and pain usually without significant weakness. Because the platinum analogs accumulate in the cell body, the deficits can progress for months following treatment, a phenomenon known as coasting. Electrophysiologically, neuropathy due to platinum analogs, may demonstrate a widespread decrease in sensory nerve action potential (SNAP) amplitudes without a proximal to distal gradient. In such cases of non–length-dependent neuropathy, it is possible to see an electrophysiologic pattern wherein the SNAP amplitudes in the upper extremities (shorter nerves) are lower than those in the lower extremity nerves (longer nerves).87 This pattern is useful not only in differentiating non–length-dependent from length-dependent neuropathies associated with tubulin inhibitors, but also from other causes of peripheral nervous system dysfunction likely to be encountered in the oncology setting including radiculopathy, chronic idiopathic demyelinating polyradiculopathy, and diabetic neuropathy.
The need for an evaluation of neuropathy is largely dependent on the context in which a patient develops neuropathic signs and symptoms. If a patient develops the expected signs and symptoms from chemotherapy or other treatment that is expected to produce those symptoms, then no further investigation is generally indicated. It is when a patient develops signs and/or symptoms that exceed what is generally anticipated, or at a time when they would not have been expected to have complications of treatment that a work-up is indicated. For instance, a patient with metastatic prostate cancer who develops lower extremity pain and weakness on hormonal therapy needs an evaluation as opposed to one who develops the same symptoms on paclitaxel. In the former case, it is prudent to consider a very broad differential diagnosis and eliminate possibilities systematically and logically because many of the causes of such symptoms are curable or highly treatable. Oncologic causes such as epidural spinal cord compression, leptomeningeal metastases, intramedullary metastases, and paraneoplastic disorders should be considered along with degenerative causes of lower extremity pain and weakness such as spinal stenosis, disk herniation, facet arthropathy, and compression fracture. Similarly, idiopathic and acquired causes of neuropathy should be considered including disorders such as diabetic amyotrophy, vitamin B-12 deficiency, Lyme disease, alcoholism, paraprotein neuropathies (MGUS), and CIDP. As with the nerve root and plexus, radiation can cause damage to any peripheral nerve in the radiation field. Such damage is usually obvious when it affects one or more named nerves in an extremity but may be less obvious when it affects nerves on the trunk such as the dorsal scapular nerve to the rhomboid muscles or the supraspinatus nerve to the supra and infraspinatus muscles. These nerves are commonly affected by MF radiation and radiation for head and neck cancer. Radiculopathy mimicking peripheral neuropathy is an extremely common cause of lower extremity symptoms in cancer patients due to their often advanced age and predisposition to spinal degenerative disease. Acquired neuropathy, including MGUS and CIDP, are also relatively common and should be considered in the evaluation of patients with idiopathic neuropathies.82,88
A prospective neuropathy assessment algorithm intended to be used before and during the administration of neurotoxic chemotherapy in breast cancer patients is presented in Figure 152.4.66 Although intended for breast cancer patients, this algorithm, including the evaluation and assessment outlined, is widely applicable.
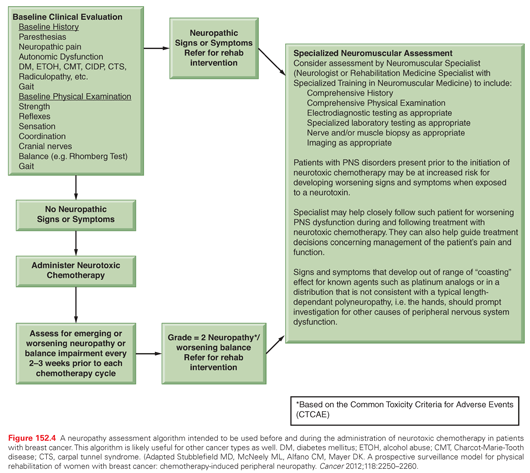
Myopathy
Myopathy in the cancer setting can occur as a result of a variety of processes including direct invasion by a primary or metastatic tumor, paraneoplastic disorders, inflammatory disorders (polymyositis, dermatomyositis, inclusion body myositis), infiltrative disorders (amyloid), toxins, or more commonly as a result of corticosteroid use or critical illness. Radiation can cause a focal nemaline rod myopathy.89 Skeletal muscle metastases are relatively rare but can occur from a variety of tumor types including lung, breast, melanoma, thyroid, and liver.90 Paraneoplastic necrotizing myopathy characterized by rapidly progressive proximal muscle weakness that occurs over months can be seen in association with breast, small cell lung, gastrointestinal, genitourinary, bladder, ovarian, and prostate carcinomas as well as melanoma, multiple myeloma, and head and neck cancer.91 Each of the inflammatory myopathies has a distinct clinical phenotype and unique myopathologic features.92 Amyloid myopathy is rare but can be seen as a complication of multiple myeloma or primary amyloidosis.93,94 Vincristine, alcohol, and a variety of other agents can cause a toxic myopathy.95
By far, the most common therapy-induced myopathy is steroid myopathy from glucocorticoid use. Steroid-induced myopathy is characterized by fast-twitch or type II muscle fiber atrophy with decreased fiber cross-sectional areas and reduced myofibrillar protein content.96 Steroid use is associated with a variety of adverse side effects that are related to the total and daily dose, duration of therapy, and poorly understood patient susceptibility factors.97 In one small prospective study, glucocorticoid use was found to contribute to proximal muscle weakness in 9 out of 15 (60%) patients and was severe enough to interfere with ADLs in 6 out of 15 (40%) treated patients.98 The development of steroid myopathy was rapid, occurring within 15 days in 8 out of 9 patients who developed weakness. Of 16 patients, 10 (63%) also demonstrated a significant decline in respiratory function. Steroid myopathy is painless and usually demonstrates proximal weakness most evident on physical examination to affect the shoulder and hip girdle muscles with relative preservation of distal muscle strength. This pattern of weakness helps differentiate steroid myopathy from most types of neuropathy, which cause distal relative to proximal weakness. In addition, sensation and reflexes should be preserved in steroid myopathy but which is abnormal in neuropathy. Although most cases of steroid myopathy are mild to moderate, at its extreme measure, it can cause complete tetraplegia and respirator dependence. Most patients with steroid myopathy recover, some completely; however, the course of resolution can be on the order of months to years.
Critical illness myopathy is not unique to the cancer setting, but due to the nature of cancer, is commonly seen. Critical illness myopathy is often associated with critical illness polyneuropathy. Often, the first sign of these disorders is difficulty in weaning a patient from a ventilator that is not explained by pulmonary, cardiovascular, or another disorder.99 The clinical features and time course for resolution of critical illness myopathy are similar to those of steroid myopathy.
Evaluation of Neuromuscular Disorders
The key components of neuromuscular evaluation are history and physical examination. The etiology of most disorders can be elucidated with accuracy and specificity by a skilled clinician in the majority of instances, even in the cancer setting. Additional evaluations with imaging and electrodiagnostic and laboratory testing is indicated when competing diagnoses are being considered and require exclusion. Imaging is extremely useful to exclude a tumor as a source of nerve compression, particularly of the spinal cord and nerve root. Although a variety of tests have utility in select situations, magnetic resonance imaging (MRI) is usually the modality of choice for imaging the central and peripheral nervous systems.100,101 Intravenous gadolinium is useful in the identification of peripheral nerve and plexus tumors, leptomeningeal disease, and intramedullary spinal cord tumors as well as to differentiate scar from tumor, exclude infection, and identify radiation changes.46,102 Nerve roots may enhance with gadolinium in AIDP and CIDP as well as radiation-induced radiculopathy (see Fig. 152.3).59–64,82 Electrodiagnostic testing is often extremely useful in clarifying the etiology of symptoms (radiculopathy versus plexopathy versus neuropathy versus myopathy), clarifying the type of neuropathy (i.e., axonal versus. demyelinating, polyneuropathy versus mononeuropathy multiplex versus mononeuropathy), localizing a lesion (i.e. femoral mononeuropathy at the inguinal ligament versus intrapelvic). Electrodiagnostic testing can also assist in chemotherapeutic or radiotherapeutic decision making, predict neurologic prognosis, and exclude disorders in the differential diagnosis. Specialized expertise is useful in the electrophysiologic assessment of cancer patients.81 Laboratory testing is often indicated in the evaluation of peripheral nervous system dysfunction, particularly neuropathy. Such tests may include complete blood count, erythrocyte sedimentation rate or C-reactive protein, vitamin B-12, folate (methylmalonic acid with or without homocysteine for low normal vitamin B-12 levels), a comprehensive metabolic panel (fasting blood glucose, renal and liver function), thyroid function tests, serum protein immunofixation electrophoresis, glucose tolerance test if indicated to evaluate for impaired glucose tolerance, and urine protein electrophoresis with immunofixation.103 Specialized laboratory investigations may also be indicated depending on other clinical features exhibited or expressed by the patient. Evaluations may include but are not limited to those for connective tissue diseases with vasculitis (antinuclear antigen profile, rheumatoid factor, anti-Ro/SSA, anti-La/SSB, antineutrophil cytoplasmic antigen antibody, cryoglobulins), infections (hepatitis B and C, HIV, cytomegalovirus), sarcoidosis (serum angiotensin converting enzyme), dysimmune disorders (antiganglioside antibody profile [GM1, GDla, GDlb, GD3, GQ1b GT1b, antimyelin-associated glycoprotein]), and a paraneoplastic panel (anti-Hu, anti-CV2).103
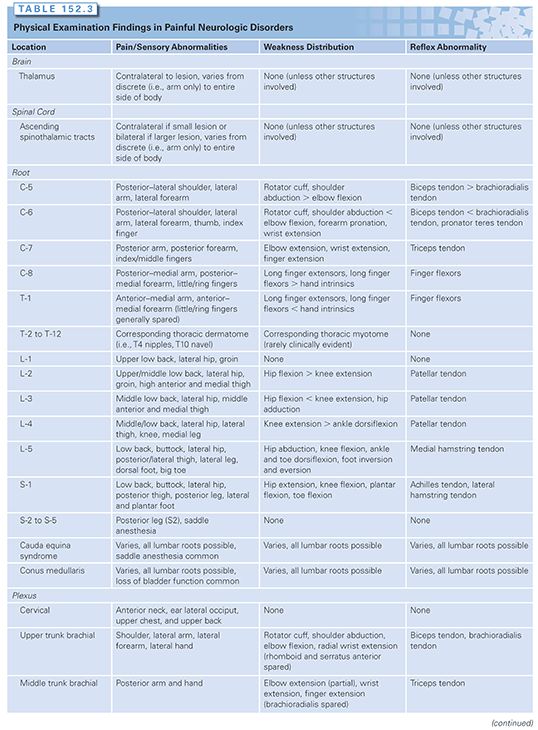
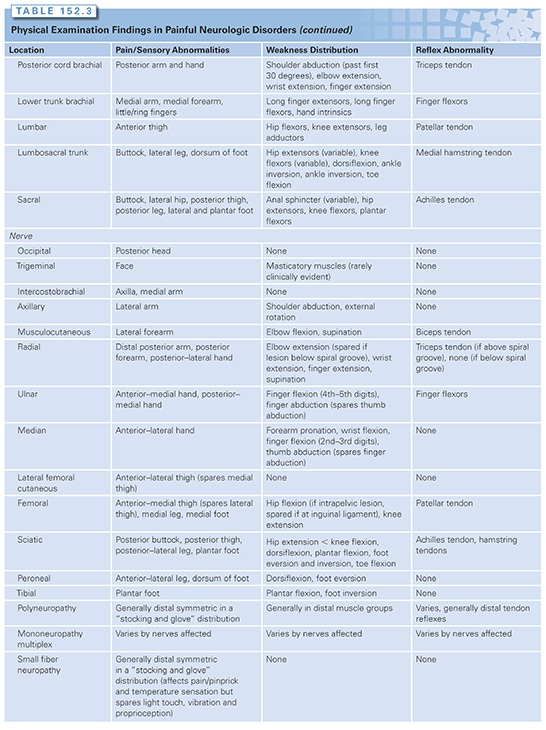
Treatment of Neuromuscular Disorders
The treatment of peripheral nervous system disorders can be highly specific (e.g., injection of a median mononeuropathy at the wrist) or extremely general (e.g., prescription of opioids for pain). The most common impairments experienced by patients with peripheral nervous system dysfunction include pain and weakness with subsequent disorders of mobility and ADLs. Skin breakdown, secondary musculoskeletal derangements (i.e., Charcot joint, rotator cuff tendonitis/adhesive capsulitis), contracture, osteoporosis, and other conditions can also result. Dysfunction of the peripheral components of the autonomic nervous system can cause orthostatic hypotension, gastroparesis, constipation, urinary retention, erectile difficulties, and a variety of other autonomic derangements.
Specific treatments depend largely on accuracy of diagnosis of the underlying disorder. For instance, severe and disabling hand pain may be from a CNS cause (thalamic or funicular pain), radiculopathy, plexopathy, a polyneuropathy, or a mononeuropathy such as the median mononeuropathy responsible for carpel tunnel syndrome. In addition to neuromuscular causes of pain, a variety of musculoskeletal disorders such as osteoarthritis and tenosynovitis, as well as infections and neoplasms, should be considered in the differential diagnosis because they can coexist with or mimic neuromuscular dysfunction.
A primary responsibility of the cancer rehabilitation physician is to be a diagnostician. An accurate diagnosis of pain and functional disorders is often instrumental in guiding oncologic colleagues on such matters as whether to continue neurotoxic chemotherapy or if a lesion requires chemotherapy. For instance, the treatment of hand pain that is due to carpel tunnel syndrome is very different from the treatment offered for a metastasis to the brachial plexus. A guide to the expected physical examination findings (pain/sensory abnormalities, weakness pattern, reflex abnormalities) anticipated from the various common central and peripheral nervous system abnormalities are listed in Table 152.3. A full accounting of the specific treatments for each of these disorders is beyond the scope of this chapter due to space limitations.
A comprehensive discussion on the management of neuropathic pain is discussed elsewhere in this textbook. Again, the first step in the effective treatment of neuropathic pain is its identification. Treatments may include behavior modification, PT and/or OT, modalities such as transcutaneous electric nerve stimulation (TENS) or ultrasound, splints and braces, injections, and other procedures and medications. Weakness and difficulties with ADLs can often be improved with PT and/or OT. Bracing may be required to compensate for weakness such as foot dorsiflexors where an AFO is often indicated. Discussions on nonpharmacologic pain management and orthotic use are presented in the following section.
MUSCULOSKELETAL COMPLICATIONS OF CANCER AND CANCER TREATMENT
Musculoskeletal disorders represent a diverse group that includes derangement of muscle, ligament, tendon, joint, cartilage, bone, and associated tissues. Included are arthritis, tendonitis, tenosynovitis, bursitis, myofascial pain, and countless other disorders. The pain associated with musculoskeletal disorders is somatic from activation of nociceptors in affected structures as opposed to neuropathic. There may be considerable overlap as when neuromuscular disorders (e.g., C-5 radiculopathy) play a causal role in the development of musculoskeletal disorders (e.g., rotator cuff tendonitis).104 As with neuromuscular disorders, musculoskeletal disorders are extremely common in the general population as well as in the cancer setting. The accurate assessment of musculoskeletal disorders, including the differentiation of benign from malignant etiologies of pain, is an important component of the cancer rehabilitation physician’s duties. It is often the case that benign musculoskeletal disorders cause as much morbidity as malignant ones. The successful identification and treatment of these disorders can help improve the function and quality of life of cancer patients and survivors. A full accounting of the assessment and treatment of the countless musculoskeletal disorders is not possible due to space limitations.
Bony Metastases
Bone metastases are a common complication of cancer, occurring in 69% of patients with advanced breast cancer.105 The primary risk of bony metastases is fracture with subsequent pain and disability. A long-bone fracture poses perhaps the greatest risk to the patient because it may prevent their ability to ambulate and perform ADLs. Preventing fractures before they occur is instrumental to maintaining function and quality of life in cancer patients. Improvements in oncologic treatments, such as hormonal and biologic therapies, have changed the time course to fix many bony metastases because patients with certain malignancies may go for months or even years with widely metastatic disease and no bony pain. When they do ultimately progress, the treatment of symptomatic bony metastases is radiation and/or surgery depending on where the lesion is located.
The concept of prophylactically fixing impending fractures was first presented by Griessman in 1947.106 Although unproven, the potential benefits include a technically easier operation, decreased operating time and blood loss, fewer complications, reduced pain, and convenience. Several strategies have been developed to predict the risk of sustaining a pathologic fracture. These predictive models are only useful in the long bones.107 Mirels proposed a scoring system to quantify the risk of sustaining a pathologic fracture through a metastatic lesion in a long bone based on the site (upper limb, lower limb, peritrochanter), pain (mild, moderate, functional), lesion type (blastic, mixed, lytic), and size relative to the diameter of affected bone (<1/3, 1/3 to 2/3, >2/3).108 Each of these four variables was given a score of 1, 2, or 3 according to the degree of risk. It was determined that lesions with a cumulative score of 7 or lower could be safely irradiated without risk of fracture, but lesions with a score of 8 or higher required prophylactic internal fixation prior to irradiation. A more recent study comparing various methods found only axial cortical involvement of more than 30 mm and circumferential cortical involvement as predictive of fracture; the former measure has the advantage of being accessible via plain x-ray.109 In practice, the actual lesion size may be difficult to delineate because of an infiltrative, permeating pattern and surrounding osteopenia. Factors such as histology (with highly vascular or lytic lesions perhaps at highest risk) and location (importance to weight bearing) are also considerations.110 Perhaps the best practical determinant of fracture risk is the degree of pain experienced by the patient both spontaneously and with evocative maneuvers such as the FABER (Flexion Abduction External Rotation) test for intrinsic pathology of the hip joint. Patients with symptomatic lesions without systemic or radiotherapeutic treatment options are likely to progress and should generally have long-bone lesions corrected surgically.
Spine stability in the oncology setting is handled very differently from the traumatic setting. The Spine Oncology Study Group (SOSG) defines spinal instability as the “loss of spinal integrity as a result of a neoplastic process that is associated with movement related pain, symptomatic or progressive deformity, and/or neural compromise under physiologic loads.”111 The assessment of spinal instability is determined based on clinical and radiographic criteria. SOGS has developed an 18-point Spinal Instability Neoplastic Score (SINS) to aid clinicians in the diagnosis of neoplastic spinal instability. This grading scheme includes elements from six parameters: location, pain, alignment, osteolysis, vertebral body collapse, and posterior element involvement. Lesions with a low SINS (0 to 6) are considered stable and do not require surgery. Lesions with a high SINS (13 to 18) are considered unstable and likely need surgery. Lesions with an intermediate SINS require a further assessment. In an analysis, the SINS demonstrated excellent inter-and intraobserver reliability for detecting clinically relevant spinal stability.112
Bilsky has developed a conceptual framework to guide therapeutic decision making with respect to surgical, radiotherapeutic, and chemotherapeutic options for spine tumors.113,114
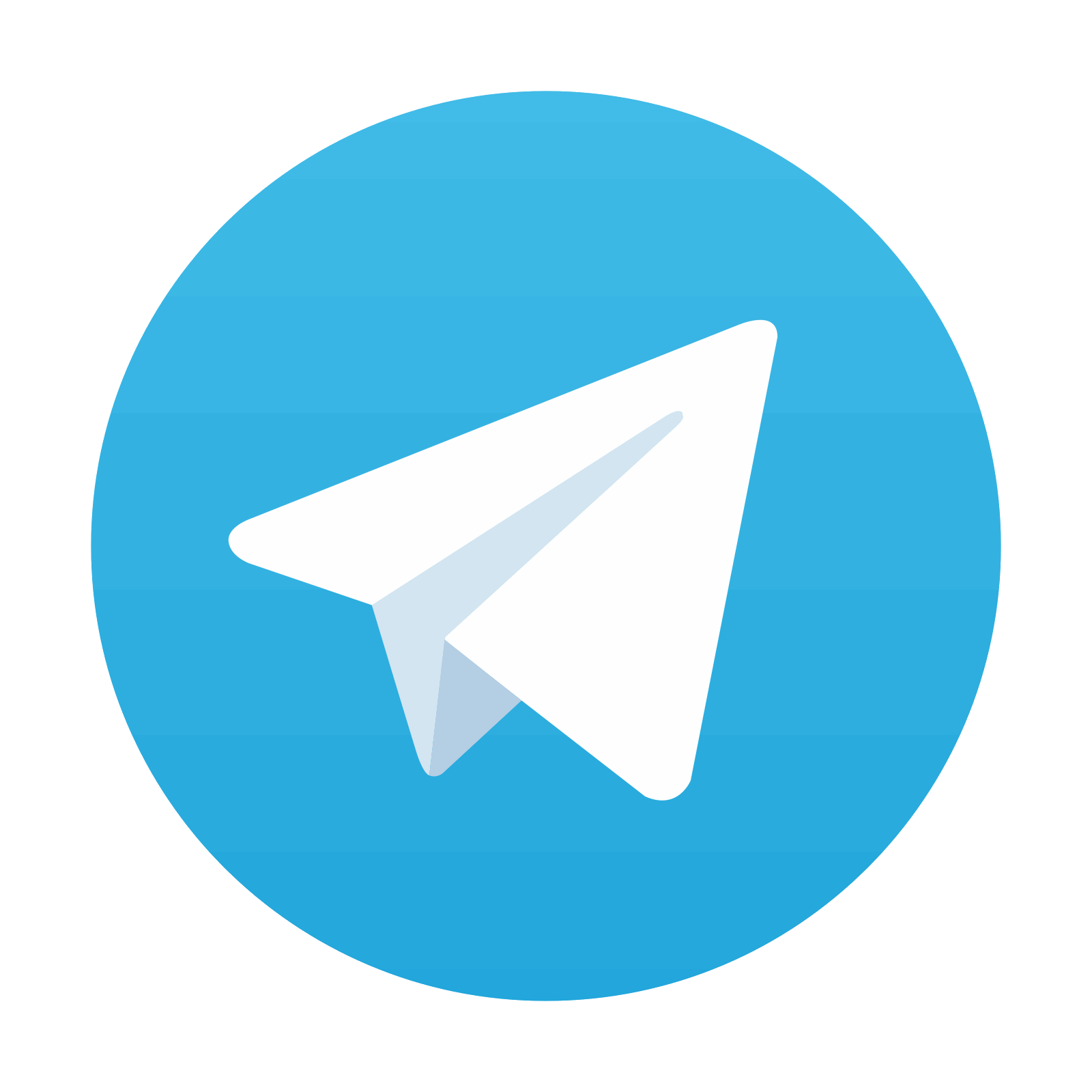
Stay updated, free articles. Join our Telegram channel

Full access? Get Clinical Tree
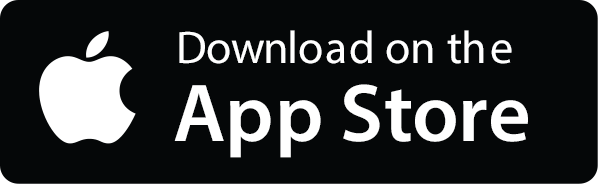
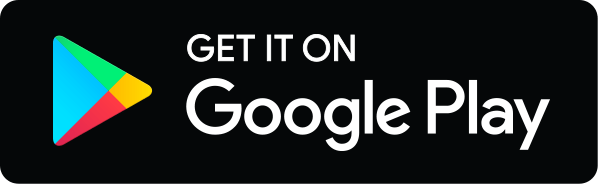