Replacement of disease-causing stem cells with healthy ones has been achieved clinically via hematopoietic cell transplantation (HCT) for the last 40 years, as a treatment modality for a variety of cancers and immunodeficiencies with moderate, but increasing, success. This procedure has traditionally included transplantation of mixed hematopoietic populations that include hematopoietic stem cells (HSC) and other cells, such as T cells. This article explores and delineates the potential expansion of this technique to treat a variety of inherited diseases of immune function, the current barriers in HCT and pure HSC transplantation, and the up-and-coming strategies to combat these obstacles.
Severe combined immunodeficiency (SCID), systemic lupus erythematosus (SLE), and type 1 diabetes share one commonality: these diverse disorders can all be attributed to faulty immune effector cells largely caused by genetic mutations that alter hematopoietic cell-intrinsic function. These defective immune cells inherit their genetic deficiencies from hematopoietic stem cells (HSCs) as they differentiate. Thus, each of these unique diseases should be theoretically curable through the same strategy: replacement of patients’ HSCs carrying the problematic mutation with normal HSCs from disease-free donors, thereby generating entire new, healthy hematolymphoid systems. Replacement of disease-causing stem cells with healthy ones has been achieved clinically via hematopoietic cell transplantation (HCT) for the last 40 years, as a treatment modality for a variety of cancers and immunodeficiencies with moderate, but increasing success. This modality has traditionally included transplantation of mixed hematopoietic populations that include HSCs and other cells, such as T cells. This article explores and delineates the potential expansion of this technique to treat a variety of inherited diseases of immune function, the current barriers in HCT and pure HSC transplantation, and the up-and-coming strategies to combat these obstacles.
Advantages of purified allogeneic hematopoietic stem cell transplantation
HSCs are the only cells within the body that at a clonal level have the ability to self-renew for life as well as give rise to all the different distinct mature effectors cells that comprise the blood and immune system. These 2 properties give HSCs the sole responsibility for the proper lifelong maintenance of hematopoietic homeostasis. However, genetic abnormalities within HSCs can result in diseases such as immunodeficiency, autoimmunity, hemoglobinopathies, or hematologic malignancies, as these defects are passed down from the HSCs to their mature cell progeny, which then generate the diseased blood or immune system.
The first successful hematopoietic cell transplant involving reconstitution of an infant immunologic deficiency was accomplished by Good and colleagues in 1968. Since then, HCT has been employed as an effective strategy to treat a multitude of hematolymphoid diseases. This procedure, more commonly known as allogeneic bone marrow transplantation, replaces mutant HSCs with functional ones from donor bone marrow grafts, which thereafter give rise to a complete normal hematolymphoid system that if stably engrafted persists for life. Although allogeneic HCT can be an effective cure for most hematopoietic-intrinsic blood or immune diseases, it is rarely performed clinically except for life-threatening diseases and in near-death scenarios because of the toxicity of the procedure. Under current practices, allogeneic HCT has a transplant mortality rate of approximately 10% to 20%, far too high to justify its routine use in most nonmalignant settings.
One of the most frequent and dangerous complications associated with allogeneic HCT is graft versus host disease (GvHD). GvHD is a complex, immunologically mediated, host-directed, inflammatory response that is attributed to transplanted donor cells genetically disparate to their host. During GvHD, grafted mature T cells, having undergone tolerization on donor rather than host thymic epithelium, upon infusion into the host result in a violent immunologic response and particularly react against host lymphoid organs, skin, liver, and gut. Although the likelihood and severity of GvHD can be minimized by transplantation from donors that are a close histocompatible match, the risks and effects of GvHD remain unacceptably high and dramatically limit HCT.
Based on presentation of symptoms, GvHD has historically been classified into 2 distinct classes: acute and chronic. Acute GvHD is rapid, occurring within 100 days of HCT and presenting as a syndrome of dermatitis, enteritis, and/or hepatitis. Chronic GvHD occurs at later time points and differs drastically from acute GvHD, often consisting of an autoimmune-like syndrome combining impairment of multiple organs or organ systems. To these 2 commonly studied subsets of GvHD is added a third important subtype, subclinical but immunosuppressive GvHD (see later discussion). Although T cells have been shown to play a dominant role in these severe complications of HCT, the exact molecular and cellular mechanisms underlying each subtype remain largely unknown.
Despite a lack of complete understanding of the pathogenesis of GvHD, one potential solution to prevent its occurrence is to transplant purified HSCs. Often the terms hematopoietic stem cell transplantation (HSCT) and HCT/bone marrow transplantation (BMT) are used interchangeably in the literature, but in reality the clinical methodology differs dramatically. Although the efficacy of BMT relies on the activity of HSC, bone marrow is composed of a heterogeneous mixture of cells, including stem, multipotent progenitors, and mature blood cells, all of which are transferred to the patient in BMT. In contrast, HSCT refers to transfer of a highly purified population of strictly HSCs obtained from the donor bone marrow. The inclusion of cell populations other than HSCs and their resulting effects are what differentiate HCT/BMT from HSCT.
HSCs are defined as cells that can give rise to long-term multilineage reconstitution, as demonstrated when they are transferred into a hematolymphoid-depleted, irradiated host. Separation based on expression of discrete phenotypic cell surface markers and verification of their functionality in this manner led to identification and isolation of human and murine HSCs. HSCs are exceedingly rare cells, making up less than 0.1% of a bone marrow graft. Based on the efforts of multiple scientific groups, the HSC population has been prospectively isolated and refined to purity. All long-term HSC activity in adult mouse bone marrow is believed to be contained within a population marked by the composite phenotype of c-Kit + , Thy-1.1 lo , lineage marker −/lo , Sca-1 + , Slamf1 + , Flk2 − , and CD34 − . Similarly, the phenotypic profile of human HSCs was validated to consist of CD34 + and Thy-1 + , in addition to lacking CD38 − , CD45RA − , and mature lineage markers. Cells with these specific phenotypes are capable of giving rise to lifelong hematopoiesis on transplantation at the single mouse-cell level into congenic myeloablated mice, and at the 10 human-cell level in xenogeneic models with myeloablated immunodeficient mice. Validation of in vivo human HSC activity with cells of this phenotype was confirmed in several phase 1 clinical trials, which showed autologous HSC-rescued blood formation in myeloablated recipients and provided sustained, prolonged hematopoiesis.
Isolation of HSCs based on the cell surface markers listed above can be accomplished by combining magnetic bead selection and fluorescence activated cells sorting (FACS) methods, yielding purified HSCs that are depleted of other polluting hematopoietic populations such as T cells. Prospective isolation of HSCs in this manner is the only effective way to completely purge grafts of contaminating, unwanted populations from clinically transplantable HSC populations. In the case of autologous transplantation to treat malignancy, human HSCs purified in this manner provide long-term hematolymphoid repopulating activity and are free of contaminating resident or metastasized cancer cells. However, in allogeneic transplantation for malignancies, HSC purification eliminates T cells that may function against the cancer and be responsible for the beneficial graft versus tumor (GvT) effect.
In allogeneic HCT for nonmalignant diseases, purification of HSCs can be profoundly beneficial and can lead to significantly diminished procedure-related toxicity. Purified HSCT decreases the adverse outcomes of HCT/BMT; because removal of T cells from allografts completely eliminates GvHD. Purification of HSCs from a graft eliminates the possibility of cotransplantation of host-reactive mature donor T cells, which are often contained within a graft and are primarily responsible for both acute and chronic GvHD. In addition to the gross lesions associated with transplantation of T cells, low doses of T cells within a graft also contribute to underappreciated subclinical GvHD. In HCT, delays in immune reconstitution can be observed even in the setting where GvHD is not readily recognized, attributable to subclinical GvHD. Even after transplantation of grafts containing minimal contaminating T cells, donor T cells attack host lymphoid tissue and destroy tissue architecture, leaving the recipient vulnerable to opportunistic infections. Transplantation of purified HSCs eliminates subclinical GvHD and results in significantly accelerated immune reconstitution, further increasing transplantation safety. As such, the complications and toxicities of BMT and HSCT are distinct, and further advocate for the transplantation of purified hematopoietic stem cells especially in nonmalignant settings.
Application of HSCT: curing a variety of nonmalignant hematolymphoid diseases
Toxicity associated with HCT has dramatically restricted its current practice to life-threatening disorders such as hematologic malignancies and bone marrow failure states, where few other therapeutic options exist. However, HCT has other important potential applications beyond its current uses if HCT-associated toxicity could be eliminated. HCT has been shown to effectively reverse nonmalignant genetic hematologic disorders such as sickle cell anemia and β-thalassemia, as well as primary immune deficiencies, if sufficient hematopoietic chimerism is achieved. In addition, early experimentation in rodents revealed that marrow transplantation could not only protect against irradiation death and prevent hematopoietic failure, but in the process could induce immune tolerance, resulting in the creation of hematopoietic chimeras that would accept skin grafts from the donor or host strain. These and subsequent studies opened the opportunity to expand this technique as a therapeutic modality for a variety of immunologic diseases, and provided a potential alternative to lifelong administration of immunosuppressive drugs following organ transplantation—the aims of transplant biologists and clinicians now for over half a century.
This phenomenon of permanent transplant tolerance is attributable to the elimination of donor-reactive T cells, primarily through negative selection in the thymus of developing T cells with donor-reactive antigen receptors. Transplantation of donor HSCs results in new immune cell generation on a chimeric microenvironment, leading to deletion of reactive immune effector cells against both host (via the thymic medullary epithelium) and donor (via donor derived thymic dendritic cells). Recent studies illustrate that allotransplantation of purified HSCs either before or concurrent with transplantation of matched donor heart tissue precludes injury and subsequent rejection of donor organs. Due to cotransplantation of either tissue organs and/or tissue stem cells with HSCs, long-term immune tolerance to donor tissues by the host can be achieved and the need for hazardous lifelong immunosuppression eliminated, as best illustrated in recent trials of kidney/bone marrow transplant patients. The use of HSCT in this manner may significantly abrogate complications of solid organ transplantation, extending organ longevity and decreasing infection susceptibility. Future cotransplantation of HSCs and solid organ tissue generated in vitro from the same embryonic or induced pluripotent stem cell may be possible, expanding the pool of transplant candidates.
The concept of induced immune tolerance by HSCT can additionally be extended to the treatment of autoimmune diseases. HCT and HSCT have been demonstrated to have utility in blocking disease pathogenesis of a wide variety of autoimmune disorders such as diabetes mellitus type 1, multiple sclerosis, and SLE. These autoimmune diseases are complex, multifactorial diseases often containing an environmental component; however, they also bear a genetic element and involve HSCs predisposed to generating self-reactive T-cell and/or B-cell clones that can react against and attack host tissues. Transplantation of the disease can be achieved by transplantation of HSCs from donors predisposed to or bearing the disorder into otherwise healthy recipients. Conversely, allogeneic transplantation of normal donor HSCs into diseased recipients generates tolerance and prevents attack of otherwise reactive tissues.
Cure of these diseases can be achieved by elimination of the host’s reactive T cells, and subsequent generation of a new nonself-reactive T-cell compartment from the disease-resistant donor. Current transplantation procedures eliminate host immune cells and thus at least initially suppress the autoimmune disease, regardless of whether autologous or allogeneic HCT is performed. However, in these autoimmune disorders the HSCs are defective and predisposed to generating self-reactive immune cells, thus autologous transplantation as illustrated in mouse models of type 1 diabetes, allergic encephalomyelitis, and SLE is not curative. As such, syngeneic transplantation of purified HSCs in a mouse model of spontaneous autoimmune diabetes mellitus provides no long-term survival benefit. Conversely, transplanted allogeneic HSCs are predisposed to generating nonself-reacting immune cells, and indeed in the same model completely prevent diabetes development throughout life. Clinical data regarding autologous transplantation for autoimmune diseases is variable. In such settings, a naïve immune system is transplanted and, depending on environmental factors, may not always result in rapid re-creation of the diseased state. Some patients show excellent and long-lived clinical remission of disease, whereas others enjoy initial symptomatic benefit with subsequent relapse.
Autologous transplantation reintroduces the host’s defective HSCs, and therefore may not result in long-term cure. As the molecular basis for various monogenic hematolymphoid diseases is determined, gene therapy may become a realistic strategy to correct autologous HSCs before transplantation. On transplantation, these few modified HSCs could reconstitute a complete, corrected hematolymphoid system that persists for life. This strategy would be instrumental in the treatment of immune diseases, in addition to genetic and acquired nonmalignant blood diseases, such as sickle cell anemia for which currently allogeneic HCT is occasionally performed. In addition, gene therapy for HSCs may play a pivotal role in generating HSCs that produce immune cells predisposed to attacking tumors. However, to date gene therapy is individual specific, and is limited by the current inability to achieve reliable and rapid gene transduction with vectors that do not by insertional mutagenesis induce diseases such LMO2-activated acute lymphocytic leukemia.
Furthermore, transplantation of HSCs generating mature cells resistant to infectious agents may prove an effective strategy to combat a magnitude of viral agents. Case reports of human immunodeficiency virus (HIV)-infected patients transplanted with HSCs from donors resistant to the disease resulted in at least preliminary cure of these patients. In these select scenarios, transplanted donor HSCs generated donor T cells bearing CCR5 defects, making them impenetrable to HIV. Long-term outcome of these studies is unknown and the feasibility of such treatments using currently available transplantation strategies is questionable. However, these studies illustrate a potential new therapeutic use of HCT if other hurdles such as supply of resistant-matched donor cells are overcome.
HCT has been repeatedly confirmed to be the singular curative therapy for this plethora of blood and immune diseases. To date, however, HCT has not been routinely applied in these manners to treat the hundreds of thousands of patients who suffer from these ailments, primarily because of concerns regarding the morbidity and mortality of allografting procedures. With elimination of GvHD by transplantation of purified HSCs that are debulked of reactive T cells, therapy of this nature may become a mainstream reality.
Application of HSCT: curing a variety of nonmalignant hematolymphoid diseases
Toxicity associated with HCT has dramatically restricted its current practice to life-threatening disorders such as hematologic malignancies and bone marrow failure states, where few other therapeutic options exist. However, HCT has other important potential applications beyond its current uses if HCT-associated toxicity could be eliminated. HCT has been shown to effectively reverse nonmalignant genetic hematologic disorders such as sickle cell anemia and β-thalassemia, as well as primary immune deficiencies, if sufficient hematopoietic chimerism is achieved. In addition, early experimentation in rodents revealed that marrow transplantation could not only protect against irradiation death and prevent hematopoietic failure, but in the process could induce immune tolerance, resulting in the creation of hematopoietic chimeras that would accept skin grafts from the donor or host strain. These and subsequent studies opened the opportunity to expand this technique as a therapeutic modality for a variety of immunologic diseases, and provided a potential alternative to lifelong administration of immunosuppressive drugs following organ transplantation—the aims of transplant biologists and clinicians now for over half a century.
This phenomenon of permanent transplant tolerance is attributable to the elimination of donor-reactive T cells, primarily through negative selection in the thymus of developing T cells with donor-reactive antigen receptors. Transplantation of donor HSCs results in new immune cell generation on a chimeric microenvironment, leading to deletion of reactive immune effector cells against both host (via the thymic medullary epithelium) and donor (via donor derived thymic dendritic cells). Recent studies illustrate that allotransplantation of purified HSCs either before or concurrent with transplantation of matched donor heart tissue precludes injury and subsequent rejection of donor organs. Due to cotransplantation of either tissue organs and/or tissue stem cells with HSCs, long-term immune tolerance to donor tissues by the host can be achieved and the need for hazardous lifelong immunosuppression eliminated, as best illustrated in recent trials of kidney/bone marrow transplant patients. The use of HSCT in this manner may significantly abrogate complications of solid organ transplantation, extending organ longevity and decreasing infection susceptibility. Future cotransplantation of HSCs and solid organ tissue generated in vitro from the same embryonic or induced pluripotent stem cell may be possible, expanding the pool of transplant candidates.
The concept of induced immune tolerance by HSCT can additionally be extended to the treatment of autoimmune diseases. HCT and HSCT have been demonstrated to have utility in blocking disease pathogenesis of a wide variety of autoimmune disorders such as diabetes mellitus type 1, multiple sclerosis, and SLE. These autoimmune diseases are complex, multifactorial diseases often containing an environmental component; however, they also bear a genetic element and involve HSCs predisposed to generating self-reactive T-cell and/or B-cell clones that can react against and attack host tissues. Transplantation of the disease can be achieved by transplantation of HSCs from donors predisposed to or bearing the disorder into otherwise healthy recipients. Conversely, allogeneic transplantation of normal donor HSCs into diseased recipients generates tolerance and prevents attack of otherwise reactive tissues.
Cure of these diseases can be achieved by elimination of the host’s reactive T cells, and subsequent generation of a new nonself-reactive T-cell compartment from the disease-resistant donor. Current transplantation procedures eliminate host immune cells and thus at least initially suppress the autoimmune disease, regardless of whether autologous or allogeneic HCT is performed. However, in these autoimmune disorders the HSCs are defective and predisposed to generating self-reactive immune cells, thus autologous transplantation as illustrated in mouse models of type 1 diabetes, allergic encephalomyelitis, and SLE is not curative. As such, syngeneic transplantation of purified HSCs in a mouse model of spontaneous autoimmune diabetes mellitus provides no long-term survival benefit. Conversely, transplanted allogeneic HSCs are predisposed to generating nonself-reacting immune cells, and indeed in the same model completely prevent diabetes development throughout life. Clinical data regarding autologous transplantation for autoimmune diseases is variable. In such settings, a naïve immune system is transplanted and, depending on environmental factors, may not always result in rapid re-creation of the diseased state. Some patients show excellent and long-lived clinical remission of disease, whereas others enjoy initial symptomatic benefit with subsequent relapse.
Autologous transplantation reintroduces the host’s defective HSCs, and therefore may not result in long-term cure. As the molecular basis for various monogenic hematolymphoid diseases is determined, gene therapy may become a realistic strategy to correct autologous HSCs before transplantation. On transplantation, these few modified HSCs could reconstitute a complete, corrected hematolymphoid system that persists for life. This strategy would be instrumental in the treatment of immune diseases, in addition to genetic and acquired nonmalignant blood diseases, such as sickle cell anemia for which currently allogeneic HCT is occasionally performed. In addition, gene therapy for HSCs may play a pivotal role in generating HSCs that produce immune cells predisposed to attacking tumors. However, to date gene therapy is individual specific, and is limited by the current inability to achieve reliable and rapid gene transduction with vectors that do not by insertional mutagenesis induce diseases such LMO2-activated acute lymphocytic leukemia.
Furthermore, transplantation of HSCs generating mature cells resistant to infectious agents may prove an effective strategy to combat a magnitude of viral agents. Case reports of human immunodeficiency virus (HIV)-infected patients transplanted with HSCs from donors resistant to the disease resulted in at least preliminary cure of these patients. In these select scenarios, transplanted donor HSCs generated donor T cells bearing CCR5 defects, making them impenetrable to HIV. Long-term outcome of these studies is unknown and the feasibility of such treatments using currently available transplantation strategies is questionable. However, these studies illustrate a potential new therapeutic use of HCT if other hurdles such as supply of resistant-matched donor cells are overcome.
HCT has been repeatedly confirmed to be the singular curative therapy for this plethora of blood and immune diseases. To date, however, HCT has not been routinely applied in these manners to treat the hundreds of thousands of patients who suffer from these ailments, primarily because of concerns regarding the morbidity and mortality of allografting procedures. With elimination of GvHD by transplantation of purified HSCs that are debulked of reactive T cells, therapy of this nature may become a mainstream reality.
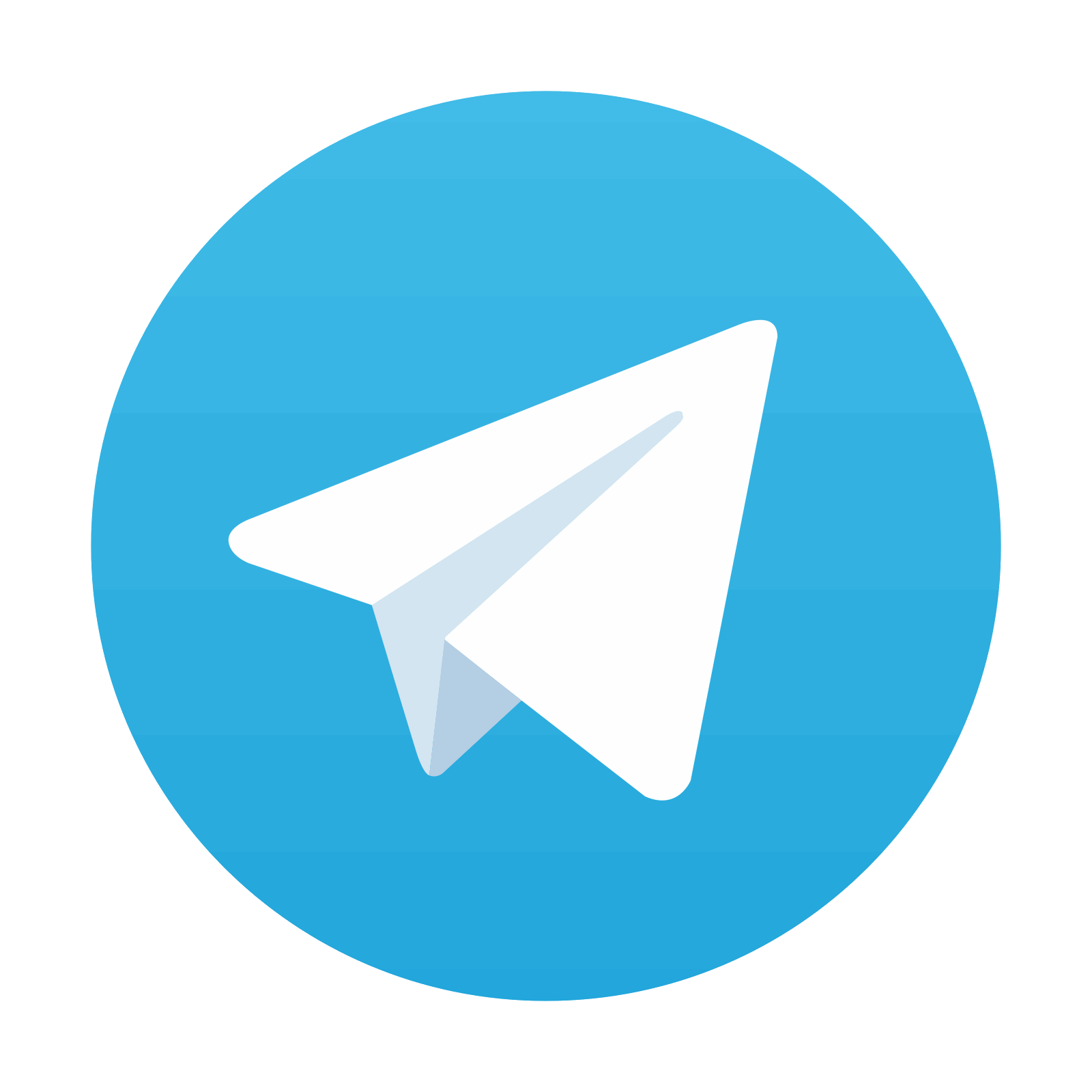
Stay updated, free articles. Join our Telegram channel

Full access? Get Clinical Tree
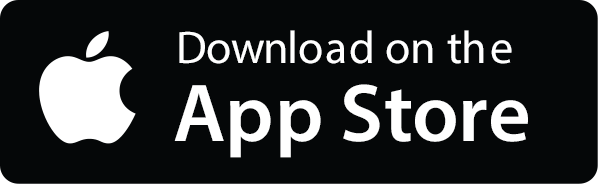
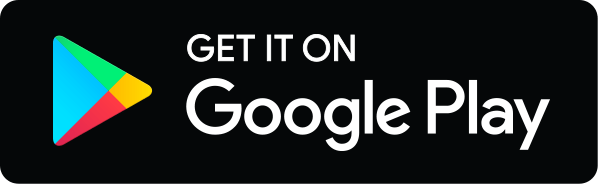