Because it is the best understood, the first models discussed concern monogenic (single-gene) mutations involving the leptin pathway (Schwartz et al., 2000). Spontaneous mutations leading to marked obesity had been described long before the underlying causes (e.g., defects in the leptin gene or the leptin receptor gene) had been discovered. Discoveries in this pathway led to the subsequent generation of a large number of engineered mutants. Another prominent animal model of obesity that resulted from a spontaneous mutation is the Otsuka Long Evans Tokushima Fatty (OLETF) rat, which lacks functional receptors for the satiating hormone CCK. Diet-induced models of obesity (DIO) are often used to study polygenic causes of obesity. DIO animals are believed to better mimic the state of common obesity in humans than most of the genetically modified models, and may be the best choice for testing prospective therapeutics. Finally, models of surgically or chemically induced and seasonal models of obesity are summarized. Surgically induced obesity has lost much of its importance since the introduction of genetically modified animals, which allow the more specific ablation of neurons in defined parts of the brain.
Most animal models of obesity are small rodents (rats or mice), but it should be mentioned that most mammals, when maintained in small enclosures with free food (as in many zoos in the past), develop obesity. The most commonly used animal models of obesity are probably the leptin-deficient ob/ob mouse, the leptin receptor deficient db/db mouse, its rat counterparts like the Zucker rat, the MC4 receptor-deficient animals and models of diet-induced obesity.
The choice of model for a particular experiment depends upon the goal of the study. For example, as described above, DIO animals may be the best choice for testing prospective therapeutics. Transgenic models or models with spontaneous mutations may be used in the evaluation of a prospective therapeutic to determine whether it engages a specific target or pathway in vivo. The transgenic models and models with spontaneous mutations may also be used to explore the role of specific molecular targets and pathways in the physiology of food intake and their potential role in obesity.
MONOGENIC MODELS: MONOGENIC MUTATIONS IN THE LEPTIN PATHWAY
Leptin and Its Receptor
Animals with a defect in the leptin-signaling pathway in the hypothalamus of the brain develop a morbidly obese phenotype. The models include animals that lack leptin production and/or that are insensitive to leptin due to leptin receptor mutations or extreme leptin resistance. Mutations are spontaneous (e.g., Lepob/Lepob mouse; Lepdb/Lepdb mouse) or genetically engineered. Animals with mutations that lie downstream of the leptin-sensing neurons in the hypothalamus are also included.
ob/ob mouse (Lepob/Lepob mouse, the ‘obese’ mouse)
A spontaneous mutation leading to the markedly obese phenotype in the Lepob/Lepob mouse has been recognized since the 1950s (Mayer et al., 1951; Coleman, 1978). However, it was not until the discovery in 1994 of the underlying mechanisms and characterization of the ob gene product, leptin, that intensive research on the genetics of obesity increased dramatically (Zhang et al., 1994). Today, the ob gene is one of the most studied genes in obesity research. A single-base spontaneous mutation of the ob gene prevents the secretion of bioactive leptin because leptin synthesis is terminated prematurely. Leptin is mainly synthesized in white adipocytes, and its secretion is directly proportional to the amount of stored triglyceride. Leptin deficiency has also been observed in rare cases of human obesity (O’Rahilly, 2009).
Phenotypically, the lack of leptin leads to marked, early-onset obesity characterized by hyperphagia, reduced energy expenditure, and hypothermia; further defects are hypercorticosteronemia, insulin resistance associated with hyperglycemia and hyperinsulinemia, hypothyroidism, and growth hormone deficiency leading to a decrease in linear growth. Lepob/Lepob mice are infertile. Obesity in Lepob/Lepob mice is one of the few forms of obesity that can be treated effectively by the administration of exogenous leptin. Leptin normalizes all known phenotypic defects in Lepob/Lepob mice including obesity, symptoms of the metabolic syndrome, and reproductive function (Coleman, 1978; Bray and York, 1979; Zhang et al., 1994; Campfield et al., 1995; Halaas et al., 1995; Pelleymounter et al., 1995; Friedman, 1998).
db/db mouse (the ‘diabetic’ mouse)
The leptin receptor-deficient “db/db” mouse, also called the Lepdb/Lepdb mouse, is phenotypically similar to the Lepob/Lepob mouse and was so named because there is more marked hyperglycemia on some background strains than occurs in the Lepob/Lepob mouse. Lepob/Lepob mice are also characterized by hyperphagia and reduced energy expenditure, leading to marked early-onset obesity; they are hypothermic, have decreased linear growth due to growth hormone deficiency, and are infertile (Coleman, 1978; Halaas et al., 1995; Chua et al., 1996). The major difference from the Lepob/Lepob mouse is the marked resistance to leptin due to the fact that the Lepdb/Lepdb mouse has a (spontaneously) mutated leptin receptor. These mice also suffer from morbid obesity, but their leptin levels are markedly elevated. Lepdb/Lepdb mice are insulin resistant and develop diabetes. They have often been used to study type II diabetes-like syndromes, but these animals do not develop the full phenotype of type II diabetes. Among other differences, they lack pancreatic amyloid deposition. Analogous to the ob gene mutation, the mutation of the leptin receptor gene is also found in some human families; however, the mutation is very rare.
s/s mouse
The s/s mouse is a more specific, genetically engineered animal model of leptin receptor deficiency (Bates et al., 2003, 2005). In contrast to the defect in the Lepdb/Lepdb mouse, which leads to generalized leptin receptor dysfunction, the s/s mouse carries a mutation that specifically disrupts the transcription factor STAT3, which is a key component of the signaling pathway of the long form of the leptin receptor and mediates leptin’s effects on energy homeostasis. The amino acid tyrosine at position Tyr 1138 is crucial for the activation of this pathway by leptin. The specific replacement of the gene encoding the leptin receptor in mice with an allele coding for a serine residue (Ser 1138 instead of Tyr 1138) disrupts the STAT3 signal. Homozygous (s/s) mice are hyperphagic and obese, but they are fertile, have normal body length, and are less hyperglycemic compared to the Lepdb/Lepdb mouse. STAT3 mediates leptin’s effects on body energy homeostasis via melanocortin signaling, whereas other signaling pathways are necessary for the control of fertility, body growth, and glucose homeostasis. Eventually, however, s/s mice develop severe insulin resistance similar to that in Lepdb/Lepdb mice, particularly in the liver.
Leptin receptor-deficient rats: Zucker rat, ZDF rat, Koletsky rat
Analogous to the Lepdb/Lepdb mouse, several rat models of leptin-resistant obesity have mutations in the leptin receptor. The obese Zucker (fa/fa or ‘fatty’ rat) and the Koletsky rat carry mutated forms of the extracellular domain of the leptin receptor. They develop a similar phenotype of hyperphagia and reduced energy expenditure, leading to morbid obesity (Bray, 1977; Bray and York, 1979). These rats have impaired glucose tolerance, a growth deficit possibly related to a lower activity of the GH/IGF-1 axis, and hypothyroidism. Fertility is reduced. Koletsky rats have a nonsense and null mutation that leads to undetectable levels of leptin-receptor mRNA expression (Chua et al., 1996; Takaya et al., 1996; Friedman, 1997; Wu-Peng et al., 1997; Crouse et al., 1998; da Silva et al., 1998). In contrast, the fa/fa mutation of Zucker fatty rats is associated with a processing defect of the leptin receptor; the receptor is generated but retained intracellularly, leading to reduced numbers of leptin receptors on the cell surface. This is associated with decreased leptin binding and signal transduction. Koletsky rats are more hypertensive and have a more severe phenotype of insulin resistance than Zucker fatty rats.
Zucker Diabetic Fatty (ZDF) rats were derived from a substrain of obese Zucker fatty rats that displayed early dysregulation of glucose metabolism. ZDF rats develop early diabetes when presented with a high-fat diet, and part of their propensity to develop early diabetes may be related to altered expression of the glucose transporter GLUT4 in skeletal muscle (Zierath et al., 1998).
Wistar Kyoto fatty rat (WDF rat)
A related rat model was created by crossing Zucker (fa/fa) rats with Wistar-Kyoto (WKY) rats. Similar to the Zucker (fa/fa) rat, the Wistar Kyoto fatty (WDF) rat develops obesity and co-morbidities like insulin resistance, hyperinsulinemia and hyperlipidemia. Male WDF rats develop early hyperglycemia and glucosuria. Insulin resistance is associated with reduced insulin binding in the brain and peripheral organs (liver) (Ikeda et al., 1981; Figlewicz et al., 1986).
Obesity Models with a Deficit Downstream of the Brain Leptin Receptor
POMC knockout mouse
Proopiomelanocortin- (POMC-) expressing neurons in the hypothalamic arcuate nucleus are direct targets of leptin. POMC is the precursor of several biologically active peptides including α-melanocyte-stimulating hormone (αMSH). In the brain, αMSH is a potent anorexigenic neuropeptide that reduces eating and increases energy expenditure by activating melanocortin (MC) 3 and 4 receptors in the paraventricular nucleus of the hypothalamus and elsewhere.
Transgenic mice lacking POMC (POMC-/-) and consequently all POMC-derived peptides overeat and develop marked obesity that is exaggerated on a high-fat diet (Yaswen et al., 1999; Challis et al., 2004). Heterozygous mutants develop an intermediate phenotype, implying that both copies of a functional POMC gene are necessary to maintain normal energy homeostasis. Although treatment with leptin is ineffective, the obesity in POMC-/- mice can be markedly reduced when these mice are treated with αMSH or other agonists of the MC4 receptor, such as MT II. POMC deficiency has also been reported in rare cases of human obesity (O’Rahilly, 2009).
POMC/AgRP knockout mice
Agouti-related protein (AgRP) is co-expressed with neuropeptide Y (NPY) in a different population of arcuate nucleus neurons than those that express POMC. AgRP increases eating by acting as an antagonist at the MC4 receptor. Recently, mice with a double knockout for POMC and AgRP were created (Corander et al., 2011). Phenotypically and in terms of their hyperphagia and development of obesity, they were similar to the homozygous POMC-/- knockout mouse. Because αMSH but not AgRP restored the defects in the control of eating and body weight, AgRP on its own seemed to be ineffective. AgRP is therefore considered as an antagonist, but not an inverse agonist, at the critical melanocortin receptors at physiological concentrations.
MC4R knockout models
αMSH and AgRP influence energy homeostasis via MC receptors. The MC4 receptor subtype in particular is involved in the control of food intake. Specific inactivation of the MC4 receptor by a targeted knockout produces hyperphagia and morbid obesity (Huszar et al., 1997). MC4-/- mice are also hyperinsulinemic, hyperglycemic, and hyperleptinemic. In contrast to many other obesity models, MC4-/- mice do not have elevated circulating corticosterone levels. MC4-/- mice do not respond to leptin, AgRP, or αMSH. Similar mutations of the MC4 receptor are often stated to be the most frequent genetic cause of obesity in humans (O’Rahilly, 2009).
An MC4 knockout rat has recently been described (Mul et al., 2011). While it has many characteristics in common with MC4 KO mice (such as increased body weight, food intake and body length, and lower spontaneous activity), there are some noteworthy differences. In particular, while the MC4-/- mouse has increased expression of NPY but not of POMC in parts of the hypothalamus, the adult MC4 knockout rat has unchanged NPY levels but increased POMC expression.
MC3R knockout mouse
Targeted inactivation of another MC receptor subtype, the MC3 receptor, also produces an obese phenotype (Butler et al., 2000). This syndrome is characterized by a comparably mild increase in total body weight but a marked, late increase in fat accumulation; i.e., MC3 knockout mice have relative adiposity. Fat oxidation in these mice is reduced, especially on a high-fat diet. The mice exhibit hyperleptinemia and relatively mild hyperinsulinemia. Physical activity in cages equipped with running wheels is reduced.
MC4/MC3 receptor knockout mouse
A double knockout of the MC3 and MC4 receptors results in mice that are heavier than the single knockouts (Chen et al., 2000). The MC agonist MTII decreases eating much less in MC3 or MC4 receptor knockout mice than in wild-type controls, but only the double knockouts are completely unresponsive to MTII. These findings suggest that at least in respect to eating, MC4- and MC3-receptor mediated actions are complementary.
Ectopic agouti expression in mice
The agouti gene was cloned in 1992 and was the first obesity gene to be characterized at the molecular level. In wild-type mice, the gene is transiently expressed in hair follicles, where it leads to the production of red or yellow pigments and inhibits the production of black or brown pigments in melanocytes by antagonizing MC1 receptors. Mice with a spontaneous ectopic agouti gene mutation are yellow (‘yellow mice’).
Several agouti mutations have been described (Michaud et al., 1994). The lethal yellow (Ay) mutation is one of five dominant agouti mutations leading to ectopic agouti expression. Homozygous expression of the spontaneous mutation is lethal, and mice typically die before implantation. Heterozygotes are viable and, in addition to having yellow hair color, they usually develop obesity within the first few months of life. Obesity results from the ectopic expression of the agouti gene product, especially in the hypothalamus, where, like AgRP, it functionally antagonizes α-MSH at MC3 and MC4 receptors. Lean body mass is slightly increased, and adipose tissue mass is increased due to fat cell hypertrophy. In association with the obesity, the mice are prone to developing type II diabetes and are hyperleptinemic. The mice are infertile. Engineered transgenic mice that ubiquitously express agouti have a similar phenotype, arguing for a causal effect of the ectopic agouti expression.
The viable agouti Avy/- mouse also has a mottled yellow pigmentation of the hair. Avy/- mice are obese and slightly larger than their respective controls. The mice are hyperinsulinemic, hyperleptinemic, and hyperglycemic, as well as insulin resistant and leptin resistant (Yen et al., 1994; Klebig et al., 1995). Interestingly, both homozygous (Avy/Avy) and heterozygous (Avy/A and Avy/a) mice vary considerably in appearance: i.e., in the extent of the yellow fur color. The degree of obesity is correlated with the extent of the yellow fur coloration. In addition to the strong influence by the agouti-locus genotype and the genetic background of the dam, epigenetics provides a likely explanation for these differences (Wolff et al., 1998; Morgan et al., 1999).
AgRP overexpression
Agrt (also known as Art or the Agrp gene) shares sequence homology with the agouti gene. Its gene product, agouti-related protein or AgRP, is considered the natural antagonist to α-MSH at MC3 and MC4 receptors in the hypothalamus. Similar to the mice with ectopic agouti expression, transgenic mice overexpressing AgRP are obese and develop hyperinsulinemia with late-onset hyperglycemia (Graham et al., 1997). This eventually results in hyperplastic pancreatic islets.
Carboxypeptidase E (CPE) mutation
CPE is an enzyme involved in the post-translational processing of many prohormones into active neuropeptides. For example, in the periphery, CPE helps convert pro-insulin to insulin, and in the central nervous system, CPE helps cleave the POMC molecule. Specific point mutations lead to the inactivation of CPE [Cpe(fat)] and a disruption of processing and secretion of POMC and its products, including α-MSH. The latter is most likely responsible for the development of obesity in animals with disrupted CPE due to a shifted balance between α-MSH and AgRP activity at MC3 and MC4 receptors. Homozygous mice develop late-onset marked obesity and are infertile (Naggert et al., 1995; Bures et al., 2001).
OTHER MONOGENIC MODELS
Otsuka Long Evans Tokushima Fatty Rat (OLETF)
Otsuka Long Evans Tokushima Fatty (OLETF) rats are a model of mild obesity (Kawano et al., 1992; Moran and Bi, 2006; Moran, 2008). The name is derived from a colony of Long Evans rats that was selectively bred at the Tokushima Research Institute of Otsuka Pharmaceutical in Japan. The underlying pathology of OLETF rats is that of a CCK-1 receptor knockout; hence, OLETF rats and their lean counterparts (LETO; Long-Evans Tokushima Otsuka) have been used to study physiological CCK functions. Because CCK plays an important role in satiation, and because this effect is mediated by CCK-1 receptors, OLETF rats are a valuable animal model to study dysregulated control of meals, eating, and obesity. The obesity phenotype is relatively mild.
The hyperphagia and ensuing obesity of OLETF rats relates to a significant increase in the size of meals. This increase results from the lack of the CCK feedback signal that primarily projects to the nucleus of the solitary tract (NTS) in the hindbrain, but which may also result from the increased expression of neuropeptide Y (NPY) in the dorsomedial hypothalamic nucleus (DMH). The latter may also explain some apparent species differences between the OLETF rat, which becomes obese on a normal diet, and the CCK1 receptor knockout mouse, which does not (Lo et al., 2010). Interestingly, overeating and an increase in meal size in OLETF rats can be observed in rat pups 2 days of age. In contrast, obesity models with defective hypothalamic signaling systems often develop overeating and obesity later in life. This difference in the onset of overeating may relate to the maturation of the hindbrain system of satiation, a process which normally occurs much earlier than that of the hypothalamus; hence, defects in the hindbrain systems, which mainly control meal size, become obvious at a much earlier age. Consistent with this idea, the overexpression of NPY in the DMH only occurs in the third week of life of the OLETF rats. Hence, at least at this young age, the lack of the incoming CCK signal seems to be sufficient to drive overeating in OLETF rats.
As a result of obesity, OLETF rats develop diabetes; they are hyperglycemic from about 4 to 5 months of age and develop polyuria and polydipsia. Further, due to the lack of functional CCK1 receptors in the exocrine pancreas, OLETF rats respond less to CCK-induced stimulation of pancreatic secretions. While these rats seem unable to compensate for their large meals, they can prevent obesity from occurring if they have access to a running wheel.
DIET-INDUCED MODELS; POLYGENIC MODELS
Diet-Induced Obese (DIO) and Diet-Resistant (DR) Rats
Outbred Sprague-Dawley rats have been used as a polygenic model of obesity that presumably shares many characteristics with the common form of human obesity. When exposed to a high-energy (HE) diet, many Sprague-Dawley rats become obese (DIO), whereas others have a body weight trajectory similar to that of control rats on a low-energy diet. The latter are therefore called diet-resistant (DR) (Levin et al., 1986, 1997; Levin and Dunn-Meynell, 2000, 2002).
DIO and DR rats have been bred selectively over several generations (Levin et al., 1997; Michel et al., 2004). This has led to a more distinct obese phenotype in DIO compared to DR rats on HE diets. Some strains of DIO rats are now becoming obese without the necessity of being exposed to a HE diet, an effect that is more pronounced in males than in females. Interestingly, the selectively bred DIO rats are less sensitive to the hypophagic action of leptin by 4 to 5 weeks of age, at a time when they are still lean and before the body weights of DIO and DR rats start to diverge. Lean DIO rats also have a reduced leptin-induced response in several hypothalamic nuclei as assessed by the expression of phosphorylated signal transducer and activator of transcription 3 (the pSTAT3 response). The reduced response to peripheral leptin is not due to a defect at the level of the blood-brain barrier transport system. The latter system only becomes defective once the DIO rats have developed overt obesity on a low-energy diet or when exposed to a HE diet. Collectively, the data indicate that the hypothalamic leptin signaling system is defective in selectively bred DIO rats before they become overtly obese.
Interestingly, selectively bred DIO rats can be protected from becoming obese by rearing them in large litters (Patterson et al., 2010), effectively limiting the pups’ nutrient supply during the suckling period, and consequently reducing circulating leptin levels and hypothalamic leptin resistance. This reduced body weight persists into adulthood and is associated with a higher sensitivity to leptin in the arcuate nucleus, and to the anorectic effect of leptin.
Cafeteria diet-induced obesity
Rats become obese when offered a varied and palatable diet which mimics the so-called Western diet of humans (cafeteria diet) (Rothwell and Stock, 1979; Rogers and Blundell, 1984; Perez et al., 1999). Cafeteria diet–induced obesity mainly results from hyperphagia that is partly compensated by increased energy expenditure, in particular diet-induced thermogenesis (DIT) due to sympathetic activation of brown fat. Overeating of cafeteria diets is due to increased average meal size as well as increased meal frequency. This contrasts with overeating of palatable diets with no choice of foods, which mainly influences meal size.
High-fat diet-induced obesity
Even though the contribution of high-fat (HF) diets to human adiposity is disputed (Willett and Leibel, 2002
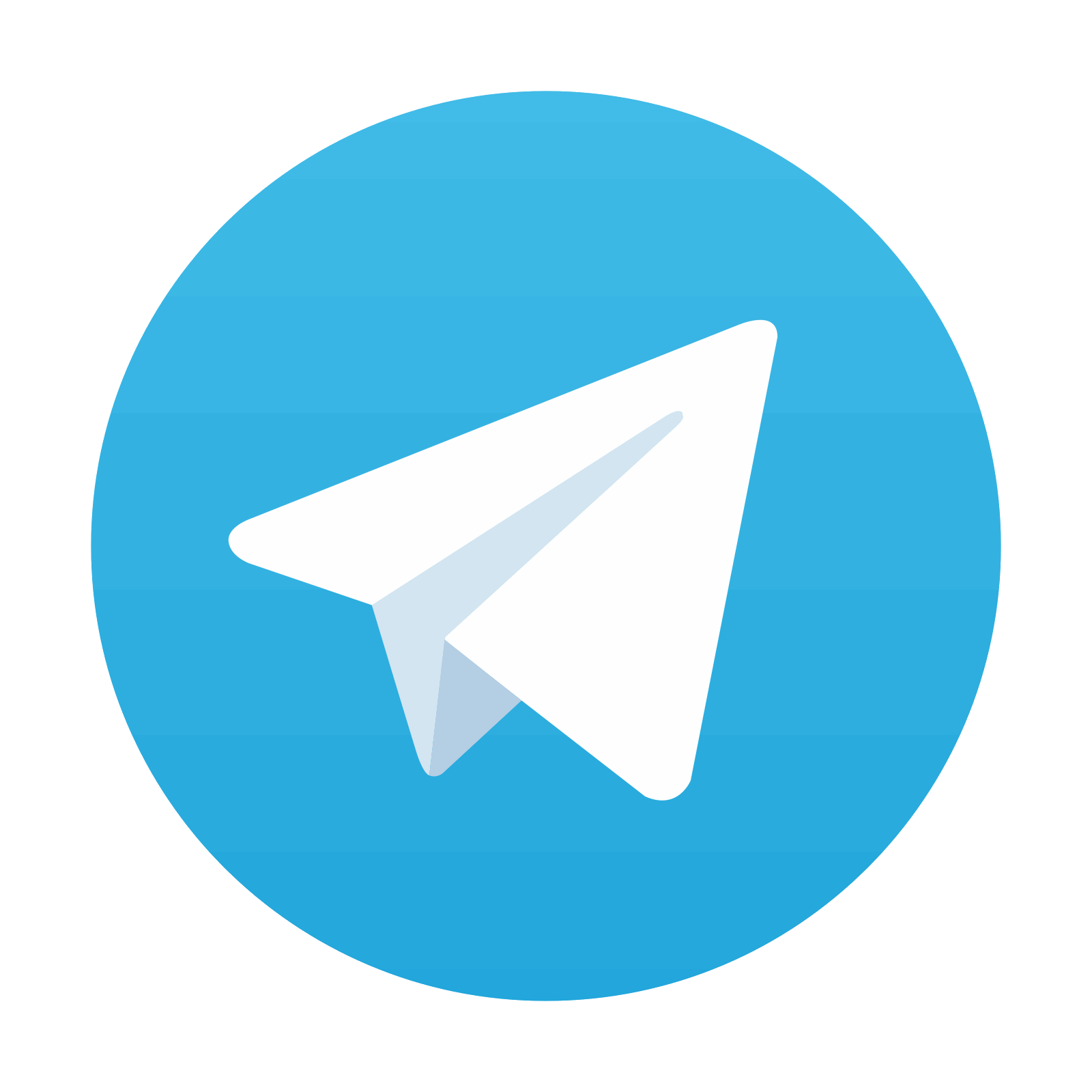
Stay updated, free articles. Join our Telegram channel

Full access? Get Clinical Tree
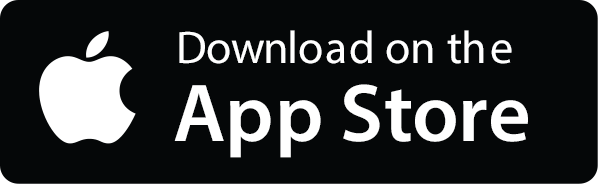
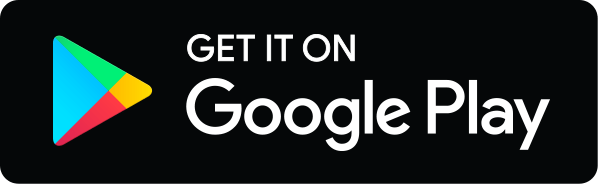