(1)
Medical Sciences Division Northern Ontario School of Medicine West Campus, Lakehead University, Thunder Bay, Ontario, Canada
Key Topics
Screening for prostate cancer (PrCa)
Molecular pathology of PrCa
Circulating cell-free DNA content as PrCa biomarker
Circulating PrCa epigenetic biomarkers
Circulating PrCa noncoding RNA biomarkers
Circulating PrCa protein and metabolomic biomarkers
Circulating PrCa cells
Key Points
PrCa is the most commonly diagnosed male cancer. Increasing screening efforts have led to early detection of curable diseases; however, this has been associated with the criticisms of overdiagnosis and overtreatment. Biomarkers that can forecast the biologic behavior of low-grade tumors are needed.
Because of the less than optimal accuracy of the PSA test, there have been great interests in uncovering circulating biomarkers for PrCa.
These research efforts have generated numerous discovery biomarkers needing validation. Some promising ones (e.g., IL-6 and TGFβ1 levels) are incorporated into the Kattan nomogram. Circulating PrCa cells demonstrate prognostic and predictive clinical utility.
12.1 Introduction
Globally, prostate cancer (PrCa) is the most commonly diagnosed non-cutaneous malignancy, and a major cause of cancer-related deaths in men. In 2012, the estimated incidence was 1.1 million with 307,000 case fatalities worldwide. The 2016 estimates are 180,890 new cases with 26,120 expected deaths for the US. The mortality rate falls significantly below the incidence rate, partly due to intense screening detection of mostly indolent tumors, especially in the more developed world. Consistent with this possibility, most cases of PrCa (~70 %) are diagnosed in the more developed parts of the world such as Australia, New Zealand, North America, and Europe. The geographic regions second to the aforementioned with increased PrCa diagnosis include the Caribbean, South America, and South Africa, with the lowest incidence rates in Asia.
Prostate cancer is screened for by the prostate-specific antigen (PSA) test and digital rectal examination (DRE), whereby an abnormal finding in either of these triggers a biopsy for evident histopathologic diagnosis. But because of biopsy sampling errors (because it randomly samples small volume of the gland), false negative outcomes are concerns to the urologic community. Similarly, clinical trials have shown that the finding and treatment of clinically insignificant tumors are even of a major concern not only from an economic perspective but also the physical and psychological harm it brings to the patient. This heightened evaluation of men over 50 leads to overdiagnosis and overtreatment.
Similar to breast and other cancers, PrCa is very heterogeneous, with unpredictable disease course. Some tumors may grow slowly (indolent cancers) and yet others can be very aggressive and extremely lethal. Due to the obvious limitations of PSA, and that DRE-detected tumors are not early cancers, the need for accurate early detection biomarkers is being actively sort after. Of even much importance are biomarkers that can accurately differentiate between indolent and aggressive cancers (the Holy Grail PrCa biomarker). Thus, companion diagnostic biomarkers that can be assayed noninvasively, preferably at the community level or at home (the lab-on a chip technology), will be an extremely helpful armamentarium.
Castrate-resistant PrCa (CRPC) poses a major challenge in PrCa management. Prostate cancers are addicted to androgen signaling for survival. Androgen deprivation is therefore a mainstay therapeutic strategy; however, the PrCa cell eventually becomes resistant to these treatments, which is a major issue with PrCa management. While advances are being made to develop alternative and more effective pharmacologic agents, docetaxel remains the first-line chemotherapeutic agent. While this treatment has major toxicity issues, only ~50 % of men will demonstrate some response. Noninvasive companion diagnostic and predictive biomarkers are needed, and these should be included in drug development protocols.
12.2 Screening Recommendations for PrCa
In general, there are no consensus guidelines or recommendations for PrCa screening. The reason is simply due to the fact that the PSA test is not accurate enough as a screening biomarker. Though it may have some benefits, many men with elevated PSA are subjected to invasive biopsy procedures, with possible detection of indolent cancers. Often many of these men are also subjected to unjustifiable treatments with their associated complications. The concept of overdiagnosis and overtreatment is well established in the PrCa field. Thus, screening recommendations vary considerably between various agencies and hospitals. The US Preventive Services Task Force, for instance, does not recommend PSA screening for PrCa. Similarly, because many PrCas are indolent, screening for men aged 79 or older is not recommended.
The American Cancer Society, however, has cautious recommendations aimed at making sure the individual and his healthcare team make an informed decision on screening. It is left open for men and their healthcare providers to decide on screening based on risk factors the person may have in relation to the potential benefits, prior to embarking on screening. This decision-making process takes into account the age of the individual, as well as family history of PrCa, and should start at:
Age 50 for the average-risk individual who has a life expectancy of at least 60 years
Age 45 for men with a first-degree relative diagnosed with PrCa at an age <65, as well as African-Americans
At age 40 for men at elevated risk because they have as least two first-degree relatives diagnosed with the disease at an earlier age
The screening program involves periodic PSA testing and DRE. The initial or prior screening results should inform subsequent screening intervals. Men with PSA of <2.5 ng/mL should be screened every couple of years, while those with ≥2.5 ng/mL need annual testing. The patients’ overall health status, PSA values, and preferences are to be considered in the screening process.
The Memorial Sloan Kettering Cancer Center (MSKCC) has recommended guidelines equally aimed at reducing overdiagnosis and yet able to detect significant disease early. They recommend screening begin at age 45. Men with PSA levels ≥3 ng/mL should consider having a biopsy, while those with levels between 1 and 3 ng/mL should have repeat testing every 2–4 years. With the lowest-risk category (PSA <1 ng/mL), decision on further testing should be based on stratified ages; for men aged 45–49, repeat testing should occur at by ages 51–55; those aged 50–59, repeat at age 60; and for those aged 60–70, there should be no further testing. Prior PSA levels and health status of men 71–75 should guide further testing, and for men ≥76, testing is not recommended.
12.3 Molecular Pathology of PrCa
With advancing age, the normal prostate glandular epithelium undergoes changes that may lead to PrCa. Inflammatory conditions may cause proliferative atrophic lesions (PIA). Similarly, prostatic intraepithelial neoplastic (PIN) changes may advance to high-grade lesions (HGPIN). Both PIA and HGPIN are precursor lesions of localized PrCa that may metastasize.
Prostate cancer demonstrates a unique molecular pathology, quite different from other solid tumors. Unlike many solid tumors, mutations are rare events, while epigenetic alterations play a major role in PrCa development and progression. Similarly, genomic rearrangements leading to fusion gene formation underlie PrCa development and progression. Genome-wide interrogation indicates PrCa cells harbor 1–2 mutations per megabase, and the frequency appears to increase with disease progression. These global genomic profiles enabled identification of a novel gene (SPOP) that is commonly mutated in PrCa, especially those lackingTMPRSS2-ERG fusions that characterize 27–79 % of PrCas and some precursor lesions.
12.3.1 Epigenetic Alterations in PrCa
The epigenome is altered in PrCa, and this is often an early event in disease progression. DNA methylation changes, histone modifications, and altered miRNA expression have all been demonstrated features of PrCa.
12.3.1.1 DNA Methylation in PrCa
Well established in the molecular progression model of PrCa is early gene promoter hypermethylation. Currently, there are over 50 genes silenced through promoter hypermethylation in PrCa. In many instances, these genes harbor infrequent mutations, LOH, deletions, or other genetic aberrations, except the epigenetic changes, which are often the primary mechanism of their silencing. Of interest, several of the hypermethylated genes in PrCa are age associated, being equally hypermethylated in association with the aging process. This offers further evidence for age being a risk factor of PrCa. Some of the commonly hypermethylated genes are GSTP1, APC, RASSF1A, RARβ2, CCND2, PTGS, ESR1/2, CDKN2A, EDNRB, HIC, MDR1, CAV1, CDH1, and BCL2. Less well studied is gene activation via promoter hypomethylation. However, global hypomethylation is associated with metastatic PrCa (mPrCa), and ~50 % of PrCas harbors hypomethylation, especially at DNA repeats such as LINE sequences. Several overexpressed genes due to promoter hypomethylation are also noted, and these include S100P, PLAU, WNT5A, CAGE, HPSE, CRIP1, and CYP1B1.
12.3.1.2 Histone Modifications in PrCa
Posttranslational modifications of histone proteins in association with chromatin remodeling underlie altered gene expression in PrCa. Noteworthy are altered expression of genes such as EZH2, HDAC1, and lysine-specific demethylase 1 (LSD1) involved in histone modification. EZH2, a histone methyltransferase involved in trimethylation of histone H3K27 (and also dimethylation of H3K9), is highly overexpressed in PrCa. Additionally, EZH2 overexpression is associated with silencing via promoter hypermethylation of PrCa-associated genes such as NKX3.1 and DAB2IP involved in EMT. HDAC1 upregulation is also a feature of PrCa, and this is more often associated with castration-resistant prostate cancer (CRPC).
12.3.1.3 MiRNA Alterations in PrCa
The new players in epigenetic regulation are miRNAs. Several miRNAs are deregulated in PrCa with yet to be fully deciphered functional importance. Some mechanisms of acquisition of androgen independence and hence CRPC emergence are mediated by miRNAs. Loss of miR-125b is associated with androgen independence. Similarly, reduced expression of miR-488 increases expression and hence transcriptional activity of androgen receptor (AR). Additionally, androgen independence is under the control of miR-221/222, and miR-616, all of which are deregulated in PrCa. MiR-331-3p, downregulated in PrCa, controls HER2 expression and AR signaling. Decreased miR-146a is associated with hormone-refractory PrCa. Additionally, several miRNAs are deregulated in PrCa, and these target important genes in PrCa cells. MiR34c targets and destroys BCL2 and E2F3 and hence is downregulated in PrCa, and miR-34a also downregulated in PrCa targets SIRT1 and BCL2. MiR-21, upregulated in PrCa, targets PTEN and PDCD4, while downregulation of miR-201 enhances EMT. Several hundreds of other miRNAs including let -7c, miR-15a, miR-16-1, miR-125, and miR-145 with reduced expression in PrCa target RAS, E2F3, BCL2, and MCL1. Thus oncomirs (upregulated) and tumor suppressormirs (downregulated) characterize prostate carcinogenesis.
12.3.2 Genetic Alterations in PrCa
Chromosomal abnormalities associated with aberrant oncogene and tumor suppressor gene expressions, as well as deregulated polycomb and developmental regulatory genes, modulate prostate carcinogenesis. Efforts at uncovering these genetic changes have led to the development of valid biomarkers for PrCa.
12.3.2.1 Chromosomal Aberrations in PrCa
Standard cytogenetics has not been that good for analysis of epithelial tumors because of the need for fresh samples, and also epithelial cancer cells grow poorly in vitro. Cytogenetic approaches have thus been very useful in analysis of hematologic malignancies. The evolution of comparative genomic hybridization (CGH) technology has enabled structural genomic aberrations to be scored in several epithelial cancers. The principle of CGH is simply a competition between normal and tumor DNA, each labeled with a distinct fluorochrome for hybridization on normal DNA template. This normal DNA template could be metaphase chromosomes of a healthy person (cCGH) or known genomic fragments immobilized on a microarray surface (aCGH). Fluorescence ratios measured after hybridization enable detection of chromosomal loses and gains (genomic imbalances).
A large number of PrCas (up to 90 %) demonstrate some aspects of DNA copy number imbalances. Importantly, most cancers with copy number abnormalities are advanced pathologic or clinical stage disease. Over half of organ-confined PrCas do not harbor any such lesions, but as many as 89 % of metastatic cancers have copy number changes. Thus progressive acquisition of genomic imbalances propels PrCa development.
Chromosomal losses at 8p (in particular) and 6q, 10q, 13q, 16q, and 18q are very common. Less commonly involved are chromosomal gains at 7q and Xq. But 8q gains are common. Chromosome 8q gain and 13q loss are markers of metastatic extracapsular disease, independent of Gleason score. 8q gain is an independent prognostic predictor of poor disease-specific survival. Array CGH offers better resolution because it detects 2.7–3.4-fold changes. This method has been used to show that losses at 8p23 and gains at 11q13 are associated with advanced metastatic disease. 8p loss is present in ~30 % of organ-confined and ~50 % of metastatic cancers. A very large region spanning ~12 Mbp (8p21.2–8p22) has been implicated. This region contains over 50 genes, but the prostate- and testis-specific androgen regulated homeobox gene, NKX3.1 at 8p21, has been well studied. The loss of this region occurs very early in PrCa, and its effects on prostate carcinogenesis appear to involve the PTEN/AKT pathway. Haploinsufficiency of NKX3.1 is insufficient to trigger cancer (not a typical TSG). Inactivation causes PIN lesions, and overexpression reduces PrCa growth. Other genes of interest in this region are the TNFR family members including TNFRSF10, TNFRSF10a, and TNFRSF10b. These genes may act synergistically in PrCa development.
12.3.2.2 Oncogenes and Tumor Suppressor Genes in PrCa
There are several genes that are overexpressed in PrCa, but the two well-established oncogenes are MYC and the TMPRSS2-ETS. The MYC locus on chromosome 8q24 is often amplified in PrCa, and ~30 % of PrCas harbor MYC amplifications leading to overexpression. MYC is capable of transforming prostatic epithelial cells. Additionally, MYC levels are higher in metastatic PrCa (mPrCa) than localized PrCa (lPrCa), and the elevated levels in advanced stage disease are associated with worse prognosis.
While uncommon in solid tumors, fusion genes appear to play an important role in PrCa pathology. Gene fusion between the 5′ untranslated regions of androgen-regulated transmembrane protease serine 2 (TMPRSS) to the 3′ exon of erythroblast transformation-specific (ETS) transcription factor family members is common in PrCa. TMPRSS and ERG (a member of the ETS transcription factor family of genes) map to chromosome 21q22, and the two genes are separated by less than 3 megabases. In a large percentage of PrCas (30–80 %), and some PIN lesions (up to 21 %), intergenic deletion of segments between these two genes leads to the TMPRSS2-ERG fusion rearrangements, of which the commonest is TMPRSS2-ETS fusion. The remaining fusion genes in PrCa are accounted for by other rearrangements of these genes. These fusion genes have several roles in PrCa including conferring androgen independence and are often associated with worse prognosis. Their noninvasive diagnostic and prognostic use in PrCa is highly pursued using primarily urinary and prostatic fluid samples. Other fusion genes demonstrated in PrCa samples include RAF1-ESRP1, ESRP1-RAF1, C15orf21-MYC, and SLC45A3-BRAF. Their roles in PrCa are being elucidated.
Similarly, alterations in TSGs are few in PrCa, but two well established are PTEN and RB. PTEN is a major negative regulator of the PI3K/AKT pathway. Consequently, PTEN loss through LOH, methylation, and mutations is observed in a vast majority (~70 %) of PrCas. Loss of a single allele is an early event in PrCa, and this may play a role in PrCa initiation through PI3K/AKT signal inhibition of NKX3.1 expression. Homozygous loss of PTEN is a feature of mPrCa. PTEN can act in concert with other altered genes such as elevated expression of HER2/3, BMI1, and loss of TP53 to promote prostate carcinogenesis. The RB tumor suppressor is also lost in a vast majority (~70 %) of CRPC. This leads to E2F1 translocation into the nucleus and the induction of transcription of multiple genes involved in cell cycle progression as well as the AR.
12.3.2.3 EZH2 and BMI1 Alterations in PrCa
Polycomb repressor complexes 1 and 2 (PRC1 and 2) are involved in gene silencing and are upregulated in many cancers. PRC1 enzymatically modifies histones and chromatin structure leading to gene silencing. BMI1 is a ring domain-containing enzyme that participates in PRC1 functions. PRC2, however, represses gene expression through histone methylation on lysine 79 of histone H3 (H3K79me2/3), and this is mediated through its component, EZH2. Both BMI1 and EZH2 are deregulated in PrCa. EZH2 for instance is under the control of MYC, TMPRSS2-ERG, and miRNAs and is also upregulated through gene amplification. PTEN loss of function and activated PI3K/AKT signaling can phosphorylate BMI1, and this interaction is involved in PrCa progression to invasive cancers. BMI1 activity is also enhanced by EZH2.
12.3.3 Developmental Gene Alterations in PrCa
Prostate glandular formation and cellular diversification are regulated by NKX3.1, AR, and FOXA1. Abnormal expression or reactivation of these genes can cause prostatic glandular hyperplasia leading to the development of neoplastic lesions and PrCa.
NKX3.1 is a homeobox developmental gene located on chromosome 8p21, a chromosomal region with LOH in ~50 % of lPrCa and up to 80 % of mPrCa. It is a transcriptional repressor expressed early in prostate glandular development and differentiation and is required for all stages of its development. Loss of NKX3.1 carries a risk for PrCa development; however, it fails to display typical tumor suppressor functions, because some aggressive PrCas maintain normal expression, and the loss of NKX3.1 is insufficient to initiate cancer formation. There appears to be interplay between NKX3.1, MYC, and PTEN in PrCa progression. NKX3.1 represses MYC target genes. Thus MYC overexpression and loss of NKX3.1 are associated with the development of PIN. Loss of NKX3.1 also appears to coincide with loss of PTEN, suggesting possible synergistic interactions.
The AR reactivation is a major cause of the emergence of CRPC, following androgen deprivation therapy (ADT). Various mechanisms, including mutations, amplification, splice variants, posttranslational modifications, and synthesis of androgen by cancer cells, are involved in AR activation. In men with lPrCa, treatment with ADT causes gene amplification and overexpression leading to CRPC. Gain of function mutations is also detected quite often in CRPC but not in early stage untreated disease. The most common mutation (T877A) resides in the ligand-binding domain and serves to increase androgen sensitivity. Up to 25 % of CRPC harbors AR mutations. Posttranscriptional splicing and/or gene rearrangement generates constitutively active AR splice variants. The most common splice variant (AR3/AV-7) is a 35-kb intragenic tandem duplication of the third exon. Another variant is the Arv567es that results from deletions of exons 5, 6, and 7. These alternative spliced forms are overexpressed in CRPC and possibly induce alternative gene expression signature from those by the wild-type AR. Additionally, posttranslational modifications (PTM) occur in the AR that enhances its functions. These changes are more often associated with CRPC compared to treatment naïve tumors. The most common PTM is phosphorylation of different tyrosine, threonine, and serine residues on the AR. For example, pSer210 can activate the AR in conditions of low androgen levels, and this modification is associated with progression to CRPC.
FOXA1 enhances AR binding to chromatin to induce target gene expression. Expectedly, FOXA1 is overexpressed in CRPC, and this is associated with poor prognosis. FOXA1-mediated control of AR interaction with chromatin leads to expression of defined gene sets in lPrCa and CRPC. Mutations in the DNA-binding domain of FOXA1 are uncovered in PrCa, but their functional role awaits elucidation.
An intriguing finding in the development of AR is the presence and overexpression of all the necessary ingredients required for de novo androgen synthesis by CRPC cells, which suggests that these cells can synthesize and respond in an autocrine fashion to androgens, even in conditions of absence or low circulating androgens, as in ADT.
12.4 Circulating PrCa Biomarkers
The need to complement or replace the PSA test has led to the discovery of several circulating biomarkers for PrCa. Extensively investigated are alterations in ccfDNA, epigenome, noncoding RNA, and proteins. Established as prognostic biomarkers are circulating PrCa cells.
12.4.1 Circulating Cell-Free Nucleic Acid Content as PrCa Biomarkers
The levels of ccfDNA have been quantified in blood from PrCa patients using mainly the PCR technique; however, a few studies have used fluorometric, spectrometric, and dipstick (Invitrogen) approaches. In spite of the inherent technical variables of biomarker development, the available data suggest a potential role for ccfDNA content in PrCa diagnosis and prognosis, especially in men with high-risk indices such as rising PSA and abnormal DRE (Table 12.1).
Table 12.1
Diagnostic and prognostic potential of ccfDNA content in PrCa
Utility | Diagnostic performance and prognostic utility |
---|---|
Diagnosis | Sensitivity: 58–88 % Specificity: 64–94 % AUROCC: 0.708–0.881 |
Prognosis | Levels are much higher in metastatic PrCa men than those with localized disease Levels correlate with Gleason score and pT stage Levels are predictive of PSA recurrence and cancer-specific survival Levels predict surgical margin status and extra-prostatic spread |
The pioneering work by Jung and colleagues failed to reveal differences in ccfDNA content between patients with localized PrCa (lPrCa) and controls, although levels were increased in BPH and metastatic PrCa (mPrCa) [1]. However, there was a significant difference in the levels between lPrCa and mPrCa patients, and this was associated with disease prognosis. Subsequent studies by other investigators revealed clear differences in the circulating levels of DNA in cancer patients and controls, achieving a range of sensitivities, specificities, and AUROCC of between 58–88 %, 64–94 %, and 0.708–0.881, respectively. The available data indicate ccfDNA levels could be threefold higher in PrCa patients than controls. The work by Ellinger and colleagues in 2008 is noteworthy [2]. Using samples from 168 patients with PrCa, 42 with BPH, and 11 healthy controls, a sensitivity of 88 %, specificity of 64 %, and AUROCC of 0.824 were achieved, proving the potential diagnostic utility of ccfDNA in high-risk men. Papadopoulou et al. had observed elevated ccfDNA with diagnostic potential as well [3]. The cost-effective dipstick method apparently achieved comparable results to the other expensive and labor-intensive methods. The smaller samples investigated by Altimari at al. (64 cancer patients and 45 men with BPH) had a higher performance (sensitivity of 80 %, specificity of 82 %, and diagnostic AUROCC of 0.881) [4]. The multi-biomarker model approach by Chun et al. [5] may be best for enhancing the use of ccfDNA in PrCa detection. Incorporating ccfDNA in a diagnostic model with total PSA and free/total PSA improved PrCa detection accuracy by 5.6 %. While many of these works indicate promise, Boddy et al. failed to reproduce them [6]. Despite this, the verdict is in favor of the diagnostic potential of ccfDNA, if validated and probably incorporated into an algorithm with other useful PrCa biomarkers.
The prognostic potential of ccfDNA levels in men with PrCa has also been shown. Plasma DNA content is in general higher in patients with mPrCa than those with lPrCa. Additionally, these levels are demonstrated to correlate with pathologic stage and significantly predict PSA recurrence after surgery, as well as PrCa-specific survival in patients with metastatic disease.
12.4.1.1 Circulating Mitochondrial DNA Content as PrCa Biomarker
In men with PrCa, the role of ccf-mtDNA has been addressed. MtDNA content was increased threefold in men with mPrCa compared to controls [7]. Noted was the lack of correlation between the content of mtDNA and nuclear DNA. Furthermore, this study demonstrated an association between high ccf-mtDNA content and PrCa-specific survival. The work by Ellinger et al. found that while mtDNA content did not differ between men with lPrCa and BPH, it was an independent predictor of PSA recurrence after surgery [8].
12.4.1.2 DNA Integrity Index as PrCa Biomarker
The clinical importance of DNA integrity index (DII), a measure of necrotic vs. apoptotic DNA in circulation, has been examined in men with PrCa. The measurement of ten different DNA of fragment sizes between 200 and 10 kb in plasma from PrCa patients and healthy controls with DII defined as ratio of long to short fragments revealed higher index in patients than controls. DII achieved a diagnostic sensitivity and specificity of 70 % and 68 %, respectively, with the AUROCC of 0.788. Ellinger et al. amplified <200 bp of PTGS2 (apoptotic fragments) and >250 of REPRIMO (non-apoptotic fragments) and defined apoptotic index (AI) as the ratio of PTGS2 to REPRIMO DNA fragments [9]. Ironically, there were more short DNA fragments (PTGS2) in cancer patients than in men with BPH (suggesting more apoptosis in cancer cells than BPH, but the authors refer to this as being of noncancerous source), achieving a sensitivity and specificity of 88 % and 64 %, respectively, as a diagnostic. The AI was also significantly higher in cancer patients than BPH and healthy men, achieving a sensitivity of 70 % at and enhanced specificity of 82 %. Short ccfDNA fragments could also predict PSA recurrence after surgery. Feng et al. obtained plasma samples from 96 cancer patients and 122 men with BPH for measurement of ccfDNA levels and DII analysis [10]. DII was defined as the ratio of ALU247bp to ALU115bp fragments. Both of these indices (ccfDNA and DII) could differentiate men with PrCa from controls, which were men with BPH and elevated PSA (≥ 4 ng/ml) but without cancer. Mean plasma ccfDNA content was 19.74 ± 4.43 in PrCa patients compared to 7.36 ± 1.58 in men with BPH (p < 0.0001), and mean DII was 0.34 ± 0.05 in cancer patients compared with 0.19 ± 0.03 in the BPH cohort (p < 0.0001). The diagnostic performance based on AUROCC was 0.864 for ccfDNA levels and 0.910 for DII. In addition to ccfDNA content, DII also demonstrates clinical potential in PrCa patients.
12.4.2 Circulating PrCa Epigenetic Biomarkers
Prostate cancer is characterized by several gene promoter hypermethylation. However, the most commonly studied somatic gene alteration in PrCa is GSTP1 promoter hypermethylation that occurs in up to 90 % of PrCas. Similarly, although there are numerous methylated genes in PrCa, GSTP1 is most extensively studied in body fluids. Available studies have detected GSTP1 methylation at various frequencies in circulation of PrCa patients. Multiple body fluid samples (serum, plasma, urine, nucleated blood cells, ejaculates) from PrCa and BPH patients were analyzed for GSTP1 promoter methylation in csb-DNA and ccfDNA templates. Methylation was detected in 90 % of tumors, 72 % of plasma/serum samples, 76 % urine samples, and 50 % of ejaculates. Methylation as a marker of CTCs were detected by MSP in 30 % of PrCa patient samples [11]. In another cohort, tissue and matched preoperative plasma and urine samples from early PrCa and BPH patients were tested for GSTP1 methylation. Methylation was detectable in 91.3 % of PrCa and 29 % of BPH tissue samples and was detected in up to 53.6 % of plasma and urine samples [12]. GSTP1 promoter hypermethylation in PrCa and BPH patient plasma samples was explored in another series, and methylation was in 30.6 % of PrCa and matched tissue samples. The majority (92.6 %) of BPH samples were negative for GSTP1 methylation [13].
12.4.2.1 Circulating PrCa Diagnostic Epigenetic Biomarkers
Multiple studies of gene methylation in circulation using multivariate analytical approaches offer evidence of their clinical potential in PrCa. In an attempt to uncover diagnostic circulating epigenetic biomarkers for PrCa, a multigene CpG island hypermethylation in sera from PrCa patients was conducted. Sera from 226 consecutive patients comprised of 168 PrCa, 42 BPH, 11 healthy controls, and 5 incidental PrCas were subjected to GSTP1, PTGS2/Cox2, REPRIMO/RPRM, and TIG1 methylation analysis. GSTP1 and TIG1 hypermethylation significantly differentiated PrCa patients from BPH and healthy controls. GSTP1 methylation in sera, in combination with any of the other genes, was very specific for PrCa (92 %), but sensitivity was low (42–47 %). This assay could help identify men with PrCa despite negative biopsy because of the high specificity [2]. A cohort of samples from a previous study of GSTP1 methylation as a measure of tumor-derived ccfDNA [14] were examined for apoptotic index, defined as a ratio of the levels of PTGS2 (<200 bp apoptotic fragments) to those of REPRIMO (>250 bp non-apoptotic fragments). The apoptotic index was significantly higher in PrCa than BPH patients and healthy controls. Only a small percentage (1.92 %) of ccfDNA was positive for GSTP1 methylation. PTGS2 DNA concentration performed at a sensitivity of 88 % and specificity of 64 % in PrCa detection. The apoptotic index achieved diagnostic sensitivity of 70 %, and specificity of 82 %, and correlated with histologic tumor grade. Kaplan–Meier analysis showed a significant correlation between PTGS2 fragments or apoptotic index and PSA recurrence after surgery [9].
Analysis of multiple biomarkers for PrCa detection was conducted by targeting methylation of GSTP1, RARβ2, and RASSF1A and allelic imbalance (AI) at six polymorphic loci in serum samples from men with stage I–IV PrCa and healthy controls. Forty-seven percent of PrCa patients had one or more AI. Methylation was detected in 28 % of serum samples. The use of both markers increased PrCa detection to 63 %. Importantly, 63 % of PrCa patients with normal PSA were detected by this assay, and the sensitivity was 89 % [15]. Another study attempting to prove the value of biomarker panel analysis in body fluids (serum, urine) for PrCa screening targeted circulating levels of PSA, AMACR, and MMP-2, as well as methylation of RASSF1A and GSTP1. Serum and urine from 113 men with lower urinary tract symptoms were obtained for analysis. With an AUROCC of 0.706 indicated that serum measurement of MMP-2 is superior to both PSA and AMACR. The methylation status of both genes was not much better than biomarker combination, which increased the overall AUROCC to 0.788 with a sensitivity of 57.1 % and specificity of 96.6 % [16]. RARβ2 methylation in serum of PrCa and BPH patients as a novel biomarker of PrCa revealed a high detection rate of 92.9 % in samples from PrCa patients and in 10.7 % BPH patient sera, indicative of needed further exploration of this biomarker [17].
Meta-analysis provides objective information for evidence-based medicine. Thus, a meta-analysis of GSTP1 methylation in sera, plasma, whole blood, urine, ejaculate, prostatic secretions has been conducted. This work included 22 studies that met selection criteria. The pooled plasma, serum, and urine data yielded a sensitivity of 52 %, but the specificity was as high as 89 %. There was high specificity with regard to sample type, methods, and primer sequences and locations. While the sensitivity is similar to that of PSA, the much higher specificity than PSA suggests it can be used as a complementary assay to PSA to help reduce the false-positive rates of PSA and importantly in patients with biopsy sampling errors [18].
12.4.2.2 Circulating PrCa Epigenetic Biomarkers and Clinicopathologic Variables
Analyses of circulating GSTP1 methylation have also been correlated with various clinicopathologic features of PrCa patients. Preoperative sera from men with PrCa treated with radical prostatectomy and those with negative biopsies were analyzed for GSTP1 methylation. Additional set of patients comprised of lPrCa patients treated with prostatectomy, those with PSA recurrence within 2-year median follow-up, and those free of disease after 3-year median follow-up were further tested. GSTP1 methylation was in 12 % of lPrCa, 28 % of metastatic PrCa patients, as well as in 15 % of those with recurrences, but in none of those free of disease. Multivariate analysis indicated that GSTP1 methylation was a significant predictor of PSA recurrence with a HR of 4.4 [19]. In another study, serum samples from patients with hormone-refractory PrCa (HRPC), early stage disease, and healthy controls were subjected to methylation analysis of GSTP1, AR, and SFN methylation statuses. Methylation frequencies in sera from HRPC patients were 32.2 % for GSTP1, 40.3 % for AR, and 86.6 % for SFN. People with early stage disease as well as healthy controls also showed variable levels of methylation in sera. The methylation of GSTP1 and SFN significantly differentiated HRPC patients from healthy controls, and GSTP1 hypermethylation in sera from HRPC patients significantly correlated with metastasis and cancer differentiation status [20].
Earlier studies have shown that GSTP1 promoter hypermethylation in preoperative serum samples from patients with lPrCa is predictive of early PSA failure after surgery. Thus, sera from 192 men with lPrCa, 18 with metastatic HRPC, and 35 with biopsy negative for PrCa were analyzed targeting CpG island hypermethylation of eight genes (MDR1, EDNRB, CD44, NEP, PTGS2, RASSF1A, RARB, and ESR). Of all the genes, only MDR1 was positive in 38.2 % of lPrCa patients and in 16.1 % of those with recurrent disease after surgery. The remaining seven genes were negative in sera from patients with lPrCa. All the genes except CD44, PTGS2, and ESR were methylated at different frequencies in sera form patients with mPrCa, with the most frequently methylated gene being MDR1 (83.3 %). Thus, a panel of well-selected genes in serum could define metastatic HRPC [14].
12.4.3 Circulating PrCa Genetic Biomarkers
The chromosomal alterations in PrCa are examined in circulation as well. LOH in plasma as a tool for PrCa screening has been explored using a panel of 15 polymorphic markers of known TSGs. DNA concentration was higher in cancer than BPH patients. LOH was more frequent in samples from PrCa (34 %) than in BPH (22 %) patients. In this cohort, LOH was highest (22 % each) at 3p24 (THRB locus) and 8p21 (D8S360) in PrCa samples, and these differed from BPH loci with frequency of 6 % at each of D8S286, D8S360, D9S1748, and D11S898 [21]. Schwarzenbach’s group further used 15 markers for LOH analysis of tissue, plasma, and bone marrow aspirates from PrCa patients. LOH was as high as 72 % in tissue samples but 56 % and 44 % in bone marrow and plasma samples, respectively. Different markers were positive in different samples (evidence of tumor heterogeneity). For example, the highest blood markers were D8S360/D10S1765, and the highest bone marrow markers were THRB/D8S137. Twenty-two percent of patients with no clinical evidence of metastasis had tumor cells in bone marrow, of which 16 % had LOH detectable in bone marrow plasma. This is the first observation of the presence of tumor DNA in blood and bone marrow plasma of PrCa patients with implications for early detection of micrometastasis [22]. Another study by this group involved the use of 13 polymorphic markers on samples from a large patient population to establish the frequencies of AI in PrCa patients in relation to clinical and pathologic variables. Allelic imbalance was detected at a frequency of 11 % in plasma and 34 % in tissue samples. LOH at D7S522 in plasma was associated with increased prostate volume, and LOH at THRB with PSA and percent-free PSA [23]. With 12 polymorphic markers used to examine plasma from patients with lPrCa and mPrCa, the frequency of LOH was 10 % and 12 % in localized and metastatic cancer samples, respectively. However, fractionation of plasma DNA enabled increased detection of LOH in low molecular weight fractions (23 %) compared to high molecular weight fractions (7 %). Most frequent site of LOH was at marker D11S898, and marker combinations D6S1631/D8S286/D9S171 and D8S286/D9S171 were associated with increasing Gleason scores [24]. In an attempt to develop a multi-biomarker serum assay for PrCa early detection, six polymorphic markers on six chromosomes, in addition to promoter methylation of RASSF1, RARβ2, and GSTP1, were examined. Allelic imbalance and methylation were detected in 47 % and 28 %, respectively. Of noteworthy, when the two classes of biomarkers (LOH and methylation) were combined, the detection of PrCa in men with apparently normal PSA was increased to 63 %. This assay achieved a sensitivity of 89 % for PrCa detection [15]. Microsatellite alterations that are frequent in PrCa can be detected and measured in circulation of cancer patients, and this has potential clinical implications worthy of exploration.
12.4.4 Circulating PrCa Coding RNA Biomarkers
The diagnostic utility of circulating transcripts, mostly targeting hTERT mRNA, has been assessed by a number of studies. Plasma from men with elevated PSA and healthy controls were assayed for hTERT mRNA. Median hTERT mRNA values were significantly much higher in cancer than control samples. Importantly, patients with prostatitis had lower levels than the PrCa cohort. At a defined cutoff, hTERT mRNA achieved a sensitivity of 81 % and specificity of 60 % for PrCa detection. Its utility in conjunction with PSA to improve PrCa detection has been suggested [25]. Plasma hTERT mRNA as a biomarker for differentiating localized from locally advanced PrCas, as well as its prognostic utility, was evaluated using univariate and multivariate analysis in comparison with conventional tumor markers. Plasma hTERT mRNA and serum PSA levels were significantly elevated in samples from locally advanced PrCa patients than men with localized disease. Compared to PSA, plasma hTERT mRNA was less sensitive (83 % vs. 100 %) but highly specific and accurate with a higher positive likelihood ratio for differentiating localized disease from locally advanced disease. Multivariate analysis revealed hTERT mRNA and age (but not PSA) could predict advanced disease. For prognostic utility, plasma hTERT mRNA and serum PSA levels could predict biochemical recurrence in univariate analysis. Multivariate analysis identified Gleason score and PSA as significant predictors of biochemical recurrence. Although hTERT mRNA levels showed a positive prognostic trend, it failed to reach significance [26]. A follow-up study by this group examined both the diagnostic and predictive use of plasma hTERT mRNA in PrCas. The study design recapitulates the previous one. Plasma from patients with elevated PSA (n = 105) and healthy controls (n = 68) was assayed. Plasma hTERT mRNA was equally sensitive (85 % vs. 83 %) but more specific (90 % vs. 47 %) than PSA. Plasma hTERT transcript levels were significantly associated with poor prognosis and were an independent predictor of PrCa. Univariate analysis showed plasma hTERT mRNA (again and not PSA) could predict biochemical recurrence. Multivariate test identified hTERT and tumor stage as significant determinants of biochemical recurrence [26].
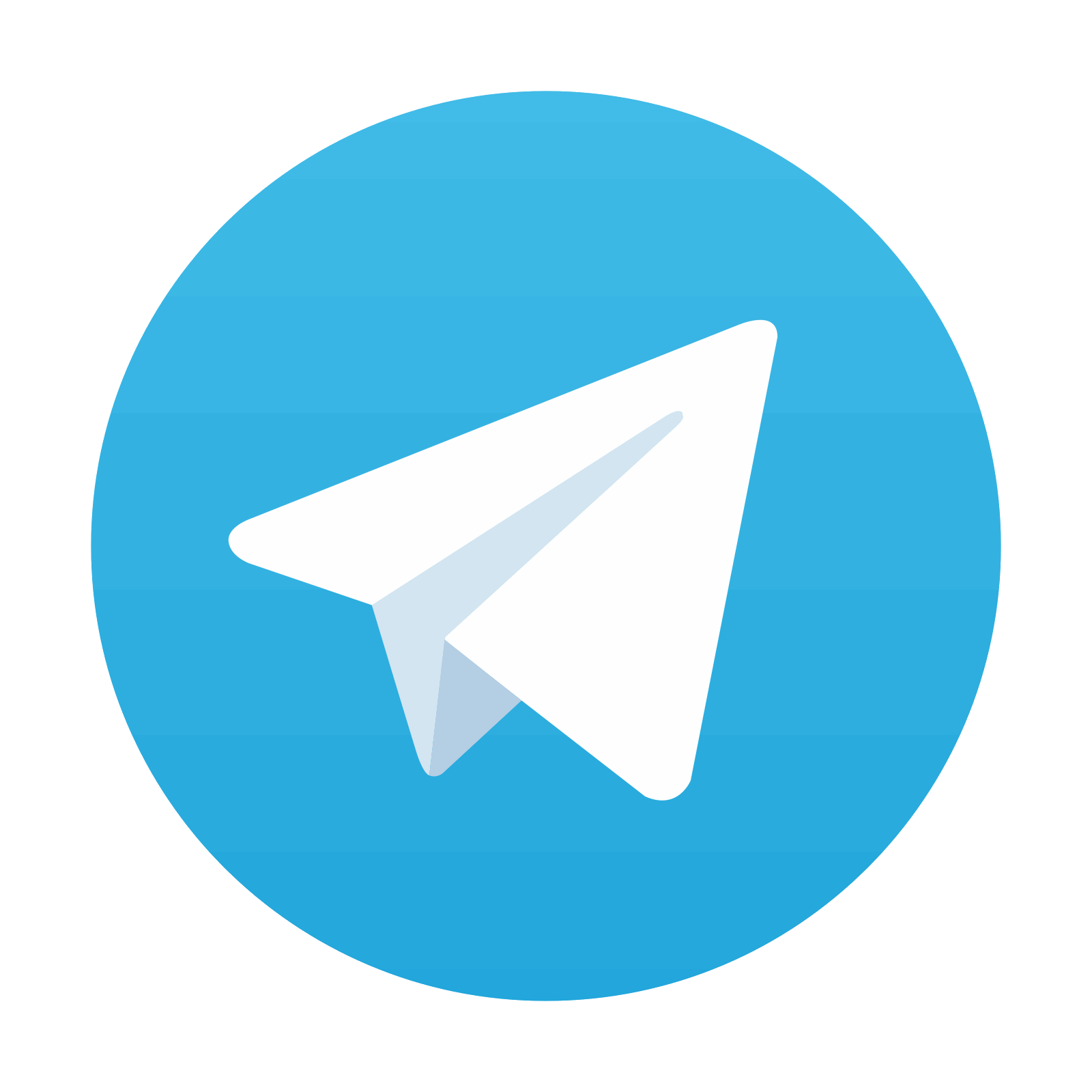
Stay updated, free articles. Join our Telegram channel

Full access? Get Clinical Tree
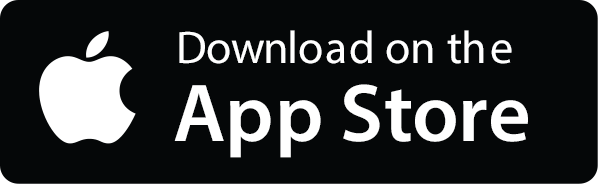
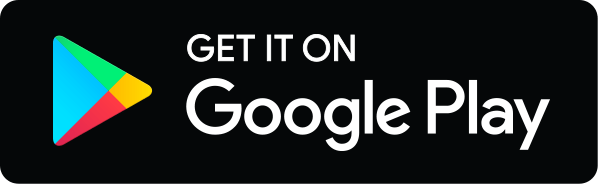