INTRODUCTION
SUMMARY
Blood neutrophil levels are maintained in a normal steady state by hematopoiesis in the marrow, the distribution of neutrophils between the marginated pool in the microvasculature and the freely circulating pool in the blood, and the rate of egress from blood to tissues. Marrow production of neutrophils is regulated by three principal glycoprotein hormones, or cytokines: interleukin-3, granulocyte-monocyte colony-stimulating factor, and granulocyte colony-stimulating factor (G-CSF), but only the genetic elimination of G-CSF has a measurable effect on blood neutrophil levels. The latter two cytokines are available as recombinant pharmaceutical products that can be administered therapeutically to ameliorate certain causes of neutropenia. Neutrophil interaction with endothelium is mediated by selectins, glycoproteins with sugar-binding sites that support shear-dependent rolling on endothelium, and by integrins on the neutrophil binding to ligands on the endothelial cells, permitting firm attachment to endothelium and emigration into tissues. Neutrophils have a short life span in blood, with a disappearance half-time of approximately 7 hours. The process can be accelerated when inflammation is present and highlights the need for a sustained rate of production to maintain a normal blood neutrophil count. The pathogenesis of neutropenia is more complex to analyze kinetically than anemia or thrombocytopenia because at least four compartments are involved: marrow storage pool, circulating pool, marginated pool, and tissue pool. The latter is particularly difficult to assay. Measurements can be further complicated in the nonsteady state, when dramatic increases in turnover rates and distribution among the four principal pools are in disequilibrium, as occurs during acute inflammatory states.
Acronyms and Abbreviations:
β2-Integrin, a member of family of receptors that mediate attachment between a cell and the tissues surrounding it; C5a, chemotactic fragment of complement component C5; CD, one of the cluster of differentiation antigens; CSF, colony-stimulating factor; DF32P, diisopropyl fluorophosphate; G-CSF, granulocyte colony-stimulating factor; GM-CSF, granulocyte-monocyte colony-stimulating factor; IL, interleukin; L-selectin (and other selectins), a member of selectin family of proteins, which are leukocyte cell-adhesion molecules; Mr, relative molecular mass; NTR, neutrophil turnover rate; T1/2, half-time; TBNP, total blood neutrophil pool; TNF-α, tumor necrosis factor-α.
DEFINITION AND HISTORY
Neutrophils are produced in the marrow, where they arise from progenitor and precursor cells by a process of cellular proliferation and maturation. They differentiate from the pluripotential stem cell1,2 through a series of progressively more committed progenitor or colony-forming units, including the granulocyte-monocyte colony-forming unit and the granulocyte colony-forming unit, which give rise to neutrophils.3,4 The early progenitor cells cannot be recognized under the microscope but can be identified retrospectively by the type of colony formed in culture of marrow cells (Chap. 18). The earliest morphologically recognizable neutrophil precursor is the myeloblast. From there, the formal sequence of precursor development is myeloblast → promyelocyte → myelocyte → metamyelocyte → band neutrophil → segmented neutrophil (Chap. 60). The term granulocyte often is loosely used to refer to neutrophils but strictly speaking includes eosinophils and basophils. Eosinophilic (Chap. 62) and basophilic granulocytes (Chap. 63) develop from progenitors in a manner analogous to the neutrophils, although commitment to neutrophilic, eosinophilic, or basophilic development probably is established at an early progenitor stage, and are dependent on the cytokines interleukin (IL)-5 and stem cell factor, respectively.
The normal human neutrophil production rate is 0.85 to 1.6 × 109 cells/kg per day. Mature neutrophils are stored in the marrow before they are released into the blood. In the absence of an inflammatory focus, they leave the circulation randomly, with a half-disappearance time of approximately 7 hours. The cells then enter the tissues and probably function for 1 or 2 days before their death or loss into the gastrointestinal tract through mucosal surfaces.
The marrow has an impressive capacity to produce neutrophils, yet it is carefully regulated both at steady state and in times of heightened demand. This chapter outlines current concepts of neutrophil production, distribution, and survival. For detailed data and methods, the reader is referred to original articles and reviews on neutropoiesis and neutrophil kinetics.5,6,7,8,9,10,11,12,13,14,15,16,17
REGULATION OF NEUTROPHILIC GRANULOPOIESIS
Although the primary cellular manifestation of commitment is the expression of receptors for lineage-specific hematopoietic growth factors, the “decision” for a stem cell to self-renew is, at least partially, a stochastic event.1,18 On the other hand, stromal elements, collectively referred to as the hematopoietic microenvironment, release short-range signals that regulate the process of commitment from multipotential stem cell pools. Although many details of hematopoietic stem cell regulation (Chap. 18) remain to be elucidated, much is known regarding the interaction of hematopoietic cytokines with their receptors and actions on the committed granulocyte progenitor cells and their mature progeny.19,20,21,22,23,24
The humoral regulators involved in granulopoiesis have been defined by in vitro culture systems.20,21 Originally identified by their ability to stimulate colony formation from marrow progenitor cells, the hemopoietic cytokines were initially termed colony-stimulating factors (CSFs) based on this assay system.25 With regard to neutrophil production, at least four human CSFs have been defined. Granulocyte-monocyte colony-stimulating factor (GM-CSF) is a 22,000 relative molecular mass (Mr) glycoprotein that stimulates the production of neutrophils, monocytes, and eosinophils. Granulocyte colony-stimulating factor (G-CSF) has a Mr of 20,000 and stimulates exclusively the production of neutrophils. IL-3, or multi-CSF, also has a Mr of 20,000 and acts relatively early in hematopoiesis, affecting pluripotential stem cells. Finally, stem cell factor (also known as c-kit ligand or steel factor), with a Mr of 28,000, acts in combination with IL-3 and/or GM-CSF to stimulate the proliferation of the early hematopoietic progenitor cells, basophils and mast cells.
In addition to their effects on neutrophil precursors, G-CSF and GM-CSF act directly on the neutrophil, enhancing its function. These cytokines regulate the production, survival, and functional activity of neutrophils.21,22,26,27 In a murine model of severe bacterial infection, endothelial cells translate pathogen signals into G-CSF–driven marrow neutrophil production.28 The mature neutrophil lacks IL-3 receptors and thus is not affected by IL-3. In fact, the genetic elimination of IL-3 obliterates delayed type hypersensitivity. However, IL-3 receptors are present on mature eosinophils and monocytes. IL-3 is produced by activated T lymphocytes and thus is expected to have a physiologic role in circumstances of cell-mediated immunity. GM-CSF also is produced by activated lymphocytes. However, like G-CSF, it also is elaborated by mononuclear phagocytes and endothelial and mesenchymal cells when these cell types are stimulated by certain cytokines, including IL-1 and tumor necrosis factor, or bacterial products, such as endotoxin.29,30,31 Stem cell factor is secreted by a variety of cells, including marrow stromal cells,32,33 and affects the development of several kinds of tissues.32,34
The activities of exogenously administered biosynthetic (recombinant) human G-CSF and GM-CSF in humans are well documented.22,27,35,36,37 G-CSF administration rapidly induces neutrophilia, whereas GM-CSF causes an increase in neutrophils, eosinophils, and monocytes. GM-CSF cannot be detected easily in normal plasma; thus, its role as a day-to-day, long-range modulator of neutrophil production is uncertain. Mice in which the GM-CSF gene is “knocked out” have generally normal hematopoiesis, but show macrophage abnormalities, pulmonary alveolar proteinosis, and decreased resistance to microbial challenge.38,39,40,41 However, G-CSF appears to be a critical regulator of neutrophil development, as giving an animal an antibody to G-CSF leads to profound neutropenia.42 The G-CSF knockout mouse shows severe neutropenia.43 Neutropenia that results from a production disturbance, such as exposure to cytotoxic drugs, is associated with high circulating serum concentrations of G-CSF.44
Methods used to study granulocyte kinetics can be categorized as follows: (1) neutrophil depletion or destruction to determine the size and rate of mobilization of reserves and the level of compensatory neutrophil production; (2) use of radioactive tracers to study neutrophil distribution, production rates, and survival times; (3) mitotic indices of marrow granulocytic cells to assess proliferative activity and cell cycle times; and (4) induced inflammatory lesions to study cell movement into the tissues. Of these categories, the most popular has been the use of radioactive tracers.
Neutrophil production and neutrophil kinetics usually are analyzed by describing neutrophil movement through a number of interconnected compartments. These compartments can be arranged into three major groups: the marrow, the blood, and the tissue (Fig. 61–1). The complexities of analyzing these compartments are covered in several recent reviews.45,46,47,48
Figure 61–1.
Scheme of maturation of neutrophil precursor cells. The myeloblast is the first recognizable precursor of neutrophils. Myeloblasts undergo division and maturation into promyelocytes and thereafter into neutrophilic myelocytes after which stage mitotic capability is lost. The major compartments of precursor proliferation and distribution are indicated across the top of the figure: marrow, blood, and tissues. The marrow precursor compartment is made up of the proliferating compartment (myeloblasts through myelocytes) and the maturation and storage compartment (metamyelocytes to mature polymorphonuclear neutrophils [PMN]). Under normal conditions, cells do not return from the tissue compartment to the blood or marrow.
Marrow neutrophils can be divided into the mitotic, or proliferative, compartment and the maturation storage compartment. Myeloblasts, promyelocytes, and myelocytes are capable of replication and constitute the mitotic compartment. Earlier progenitor cells are few in number, not morphologically identifiable, and usually neglected in kinetic studies. Metamyelocytes, bands, and mature neutrophils, none of which replicate, constitute the maturation storage compartment.
The average number of cell divisions from the myeloblast to the myelocyte stage in the proliferative compartment has been estimated between four and five.49 Data obtained using radioactive diisopropyl fluorophosphate (DF32P) suggest the existence of three divisions at the myelocyte stage, but the number of cell divisions at each step may not be constant. The major increase in neutrophil number probably occurs at the myelocyte level, because the myelocyte pool is at least four times the size of the promyelocyte pool. Because of the difficulties in measuring human intramarrow neutrophil kinetics, a precise model of the dynamics of the mitotic compartment is not available.
Table 61–1 lists the estimated sizes of the marrow neutrophil compartments and the transit times and cell-cycle stages of the cells in the various compartments. Precise studies have measured a postmitotic pool of (5.59 ± 0.9) × 109 cells/kg and a mitotic pool (promyelocytes and myelocytes) of (2.11 ± 0.36) × 109 cells/kg. These studies led to a calculated normal marrow neutrophil production of 0.85 × 109 cells/kg per day. Radioautographic studies with [3H]thymidine support the concept of an orderly progression from metamyelocytes to mature neutrophils within the maturation storage compartment. These studies also suggest a “first in, first out” pattern for cells leaving this compartment and entering the blood. Several labeling techniques indicate the myelocyte-to-blood transit time is 5 to 7 days.12,50 Previous studies with DF32P reported a range from 8 to 14 days.9,49 During infections, however, the myelocyte-to-blood transit time may be as short as 48 hours.51
Fraction in Mitosis (Mitotic Index) | Fraction in DNA Synthesis (S Phase) | Transit Time Range (h) | Total Cells (× 09/kg) | |
---|---|---|---|---|
Mitotic compartment | ||||
Myeloblast | 0.025 | 0.85 | 23 | 0.14 |
Promyelocyte | 0.015 | 0.65 | 26–78 | 0.51 |
Myelocyte | 0.011 | 0.33 | 17–126 | 1.95 |
Maturation storage compartment | ||||
Metamyelocyte | 8–108 | 2.7 | ||
Band | 12–96 | 3.6 | ||
Polymorphonuclear neutrophil | 0–120 | 2.5 |
Whether the production of neutrophils in the mitotic compartment exactly equals the neutrophil turnover rate (NTR) is not known with certainty. Studies in dogs suggest some immature neutrophils die in the marrow (“ineffective granulopoiesis”).52 Ineffective granulopoiesis has not been shown in normal humans,14,53 although ineffective granulopoiesis occurs in some pathologic states, including the myelodysplastic syndromes,54 myelofibrosis, and some of the idiopathic neutropenic disorders. At present, however, no convenient means of quantitating ineffective granulopoiesis is available.
On completion of maturation, the neutrophils are stored in the marrow and are referred to as the mature neutrophil reserve. The reserve contains many more cells than are normally circulating in the blood. Table 61–2 lists comparative data on the characteristics of the maturation storage compartment. Under stress, maturation time may be shortened, divisions may be skipped, and release into the blood may occur prematurely.
Neutrophils leave the marrow storage compartment and enter the blood without significant reentry into the marrow. The total blood neutrophil pool (TBNP) consists of all the neutrophils in the vascular spaces. Some of these neutrophils are free in the circulation (the circulating pool), while others roll along the endothelium of small vessels or are temporarily sequestered in the alveolar capillaries of the lung (the marginated pool).55,56 Cells in the two pools are freely exchangeable. When neutrophils labeled with DF32
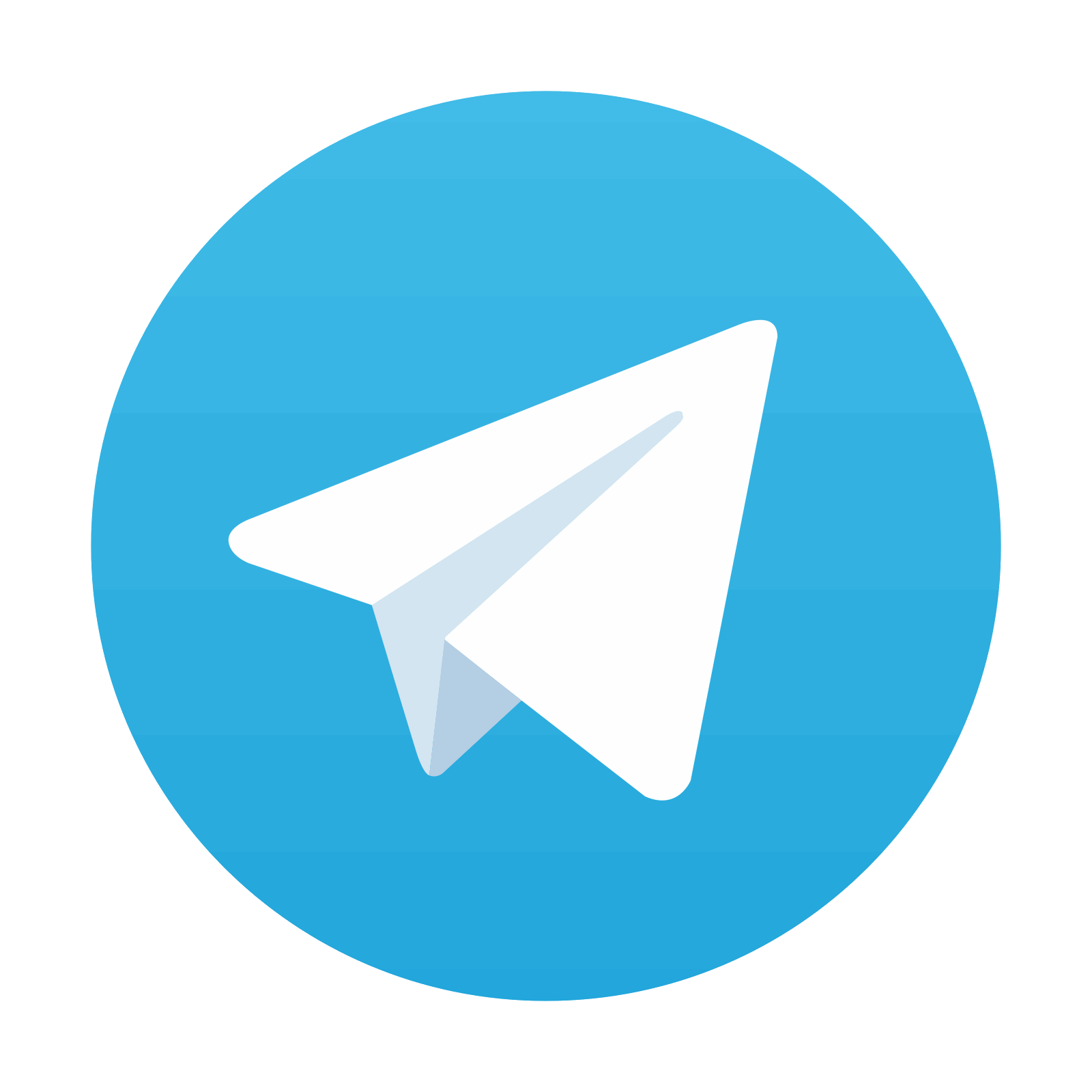
Stay updated, free articles. Join our Telegram channel

Full access? Get Clinical Tree
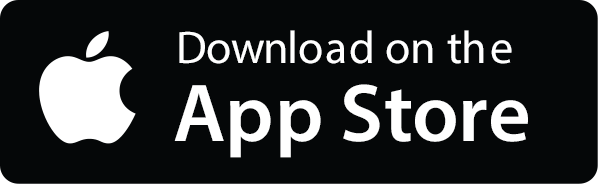
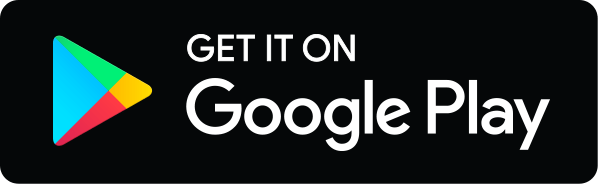
