INTRODUCTION
SUMMARY
Monocytes and macrophages play an important role in human biology, both as a component of the hematopoietic system and within the stroma and tissue microenvironment where they contribute trophic and clearance functions. They constitute a widely dispersed cellular system throughout the body, interacting with host cells and foreign invaders through their versatile biosynthetic and secretory responses, to maintain physiologic homeostasis. They are specialized migratory or sessile phagocytes, present within the circulation and extravascular tissue compartment, contributing to diverse pathologic processes directly and through their production of bioactive products. Because of their extensive heterogeneity and plasticity, the centrality of monocytes and their progeny has not always been recognized by hematologists. The origin, life span, and functions of the monocyte are the focus of this chapter, including their relevance to health and disease in humans, based on current understanding of their properties. The relationship of monocytes and macrophages to dendritic cells, and monocyte-derived cells with a specialized immunologic role in T-lymphocyte activation, are described. Together, macrophages and dendritic cells are major antigen-presenting cells, contributing to host defense, innate and acquired immunity, and inflammation, as well as noninfectious disease processes, both within and outside the lymphohematopoietic organs.
METHODS OF MONOCYTE AND MACROPHAGE STUDY
There has been a resurgence of interest in the in situ analysis of macrophages.1 Genetic/ribonucleic acid interference manipulation, more recently with macrophage-specific/restricted promoters, has been used to knock down macrophage genes or messenger RNA, and to mark cells with fluorescent labels such as green fluorescent protein. Of particular value in tracing their origins and distribution has been the use of fractalkine receptor-transgenics,2 and myeloid-specific lysozyme-Cre for targeted ablation.3 Random chemical mutagenesis has been spectacularly successful in validating known, and discovering novel, gene targets that affect macrophage functions.4,5 A wider range of experimental models (Drosophila, zebra fish) have facilitated interspecies comparisons of macrophage migration and phagocytosis in vivo.6,7 The analysis of microRNA expression8 and functions is still in its infancy and is likely to generate important insights into monocyte/macrophage gene expression in health and disease. Combined with improved imaging methods (fluorescent, nuclear magnetic resonance imaging-based, 2-photon microscopy), new insights have been obtained regarding the dynamic behavior of macrophages and dendritic cells (DCs) in vivo.9 There has been progress in provoking embryonic and induced pluripotent stem cell differentiation into macrophages and DCs in vitro, opening the possibility of introducing mutations into human genes, to complement the naturally occurring material derived from human inborn errors and resultant genetic diseases.10
Acronyms and Abbreviations:
CR, complement receptor; DC, dendritic cell; DC-SIGN, dendritic cell–specific intercellular adhesion molecule-3–grabbing nonintegrin; EMR, epidermal growth factor module-containing mucin-like hormone receptor; FACS, fluorescence-activated cell sorting; FcR, Fc receptor; GM-CSF, granulocyte-macrophage colony-stimulating factor; IFN-γ, interferon-γ; IL, interleukin; LPS, lipopolysaccharide; M-CSF, macrophage colony-stimulating factor; MARCO, macrophage receptor with a collagenous structure; MR, mannose receptor; PRR, pattern recognition receptor; Sn, sialoadhesin; SR-A, scavenger receptor A; TGF, transforming growth factor; TLR, toll-like receptor; TNF-α, tumor necrosis factor-α.
Although individual-labeled cells can be followed in accessible tissues or ex vivo, the resolution, isolation, and characterization of important embedded macrophage populations are limiting. Methods of isolation from solid organs, for example, brain and even liver and gut, are prone to artifact, and macrophages are profoundly affected by removal from their natural tissue environment. Many of the genetic manipulations introduced by transgenesis are leaky and not uniform, not surprising in the light of macrophage heterogeneity. Although the fate of recently recruited cells from blood into tissues can be tracked more easily, the slowly turning over resident populations are less easily accessed, resulting in bias. Finally, there are intrinsic difficulties with human experimentation in vivo. Induced skin blisters, for example, make it possible to collect fluid and cells from sites of inflammation.11 However, the low frequency of monocytes compared with neutrophils limits the use of ex vivo indium-labeled cells for transfer studies in vivo.
PRODUCTION
Macrophages and related amoeboid phagocytic cells, ancient in the evolution of multicellular organisms, are the main leukocytes responsible for innate immunity and tissue remodeling, as documented by Metchnikoff in his pioneering studies on invertebrates,12 and confirmed by contemporary studies on Drosophila melanogaster.7 In mammals, much of our knowledge of macrophage ontogeny derives from studies in the mouse. After origins from an aortic mesonephric site, the best understood phases of macrophage development occur during midfetal development, in the yolk sac, followed by fetal liver, spleen, and marrow, before and after birth.13 The association of macrophages with definitive erythropoiesis is a striking feature of fetal liver hematopoiesis from approximately day 12 of mouse development; macrophages then, for the first time, become intimately associated with nucleated erythroblasts, reaching a peak of hematopoietic cluster formation at day 14. The role of stromal macrophages in hematopoiesis within the adult is illustrated and discussed further in this chapter.
The association of macrophages with erythroblasts is mediated by surface adhesion molecules,14 including a poorly characterized divalent cation-dependent receptor and the sialic acid-binding molecule sialoadhesin (Siglec1).15 The potential trophic functions of stromal macrophages in marrow erythropoietic islands is poorly understood, as is the considerable role of macrophages in iron and heme metabolism. Macrophages interact with cells in numerous ways; however, during erythropoiesis a special phagocytic process allows for the removal of pyknotic erythroid nuclei during the final stages of erythropoiesis. The mechanism of recognition of membrane-bound erythroid nuclei is not clear, nor its relationship to the uptake of apoptotic cells elsewhere during development. The production of granulocytes from progenitors also involves macrophage–myeloblast clusters and similar adhesion receptors. Once fetal liver hematopoiesis declines before and after birth, the macrophages in the liver adopt the features of resident Kupffer cells. The stromal macrophages associate with developing blood cells within islands of clustered cells, a feature of hematopoiesis throughout life.16 During fetal life, monocytes and macrophages are distributed through the developing vasculature, providing amoeboid, phagocytic cells implicated in tissue remodeling, for example, sculpting of digits,17 and growth of the central nervous system.18 Blood monocytes seed resident tissue macrophage populations throughout the organism, and these cells proliferate more readily in the fetus than in later life; the adhesion molecules, chemotactic signals, and receptors involved during this constitutive phase of distribution are poorly defined, but it is independent of the β2-integrin CD11b/CD18, which plays a role in myelomonocytic cell recruitment induced by inflammation in the adult.19 The appearance of macrophages during development has been correlated with fibrous scar formation after injury.17 In sum, macrophages play a major role during development, both in hematopoiesis and in extravascular tissues, and much remains to be learned regarding their properties in the fetus.
Figure 68–1 gives an overview of differentiation of monocytic cells in the adult.20 The origins of monocytes from multipotential (progenitors colony-forming units, spleen [CFU-S]) and committed hematopoietic precursors (colony-forming units, culture [CFU-C]) and the role of lineage-restricted growth factors such as monocyte/macrophage colony-stimulating factor (M-CSF; also termed CSF-1) and granulocyte-macrophage (GM)-CSF have been studied extensively, but new details are still emerging. Both transcription factor c-Myb and receptor FLT3, M-CSF–dependent myeloid lineages (monocyte and dendritic) occur. These cell types may have different responses to tissue damage and infection.20 Monocytes share precursors with other hematopoietic cells and are closely related to granulocytes. Monocytic precursors are the source of adult tissue macrophages, as well as of myeloid DCs and osteoclasts. Their relationship to B lymphocytes and to plasmacytoid DCs is still unclear, as plasmacytoid DCs express a range of myeloid as well as lymphoid markers. There is a considerable body of knowledge about the specific growth factors and their receptors, and growing knowledge of the nature and role of transcription factors involved in monocyte/macrophage differentiation.21 Genetic and cellular abnormalities in growth and differentiation pathways underlie myeloid leukemogenesis, though rarely giving rise to monocytic leukemia.
Figure 68–1.
Differentiation of the macrophage/dendritic cell (DC) progenitor and origin of macrophage and DC subsets. CDP, common dendritic cell precursor; CMP, common myeloid progenitor; GMP, granulocyte/macrophage progenitor; HSC, hematopoietic stem cell; HSPCs, hematopoietic stem and progenitor cells; MDP, macrophage/DC progenitor; pDC, plasmacytoid dendritic cell. For further details see Ref. 20. (Used with permission of S. Seif, GraphisMedica, 2014.)
The classic studies of Lewis and Lewis22 in 1926, Maximow23 in 1932, and Ebert and Florey24 in 1939, showed that monocytes transform into macrophages and multinucleated giant cells in vitro. Macrophages can be produced from monocytes or hematopoietic progenitor cells culture in cytokines, such as GM-CSF or M-CSF.
The alterations of ultrastructure during transformation into macrophages, epithelioid cells, and giant cells have been described using purified populations of monocytes and in vitro culture techniques.25 As the monocyte matures into the macrophage, the cell enlarges in size, and the lysosomal content and the amount of hydrolytic enzymes within the lysosomes (e.g., phosphatases, esterases, β-glucuronidase, lysozyme, arylsulfatase) increase. At the time, the size and number of mitochondria increase, their energy metabolism increases concomitantly. Production of lactate also increases. The Golgi complex, which packages lysosomes, increases in size and vesicle complexity (Chap. 67). Several stimuli induce formation of multinucleated giant cells from monocytes.26
M-CSF and GM-CSF are the major growth factors implicated in monocyte and macrophage differentiation. Other cytokines, such as interleukin (IL)-3 and IL-4, result in minimal monocyte proliferative expansion, and their genetic elimination has no effect on the lineage. M-CSF promotes survival as well as growth and differentiation of macrophages, exclusively, acting through a specific receptor (CSF-1R), encoded by the protooncogene c-FMS, which has been extensively used as a lineage marker for fluorescence-activated cell sorting (FACS) analysis (CD115) and transgenesis.27,28 The role of M-CSF has been reviewed29 and its role in macrophage and osteoclast development is illustrated in Fig. 68–2. The naturally occurring mouse mutant, op/op, gives rise to M-CSF deficiency and osteopetrosis, with marked or partial deficiency in monocyte and selected tissue macrophage populations; DC numbers are unaffected.30 Unlike PU.1 deficiency, the op/op mouse is viable, though its reproductive ability is impaired, because M-CSF also plays an important role in the reproductive system. Uterine epithelium is a rich source of M-CSF, inducing monocyte-macrophage recruitment, growth and differentiation, and upregulating scavenger receptor (SR) expression, cell adhesion, and endocytosis of modified low-density lipoproteins and other polyanionic ligands. M-CSF is produced in a soluble and membrane-bound forms, is present in plasma, and has been implicated in atherosclerosis and tumor-dependent recruitment of monocytes and macrophages. The size of the growth burst induced by M-CSF depends on the stage of differentiation of the target cell, decreasing markedly as the precursors mature into monocytes and macrophages. Adhesion and inflammatory stimuli enhance the response to growth factors and can result in macrophage proliferation at peripheral sites, for example, in granulomata.
Figure 68–2.
Regulation of macrophage and osteoclast development by macrophage colony-stimulating factor (M-CSF). Circulating M-CSF, produced by endothelial cells in blood vessels, together with locally produced M-CSF regulates the survival, proliferation, and differentiation of mononuclear phagocytes and osteoclasts. The cytokine synergizes with other hematopoietic growth factors (HGFs) to generate mononuclear progenitor cells from multipotent progenitors, and with receptor activator of nuclear factor-κB ligand (RANKL) to generate osteoclasts from mononuclear phagocytes. Brown arrows indicate cell differentiation steps; blue arrows indicate cytokine regulation. (Used with permission of S. Seif, GraphisMedica, 2014.)
GM-CSF has a broader myeloid target profile. It is produced by many cells, including macrophages themselves, especially after inflammatory stimuli such as lipopolysaccharide (LPS), and it enhances production of monocytes and macrophages with a different morphology to that induced by M-CSF. GM-CSF is required for myeloid DC differentiation in vitro and has been widely used, alone and in combination with cytokines such as IL-4 or transforming growth factor (TGF)-β, to produce DC from mouse marrow or from human monocytes in cell culture.31,32 Its targeted deletion in mice or genetic loss of function mutants of its specific receptor chain in humans results in pulmonary alveolar proteinosis, associated with defective alveolar macrophage metabolism of pulmonary surfactant.33
Once the cells have acquired the characteristics of mature monocytes/macrophages, they display considerable heterogeneity in morphology and phenotypic plasticity. In general, their proliferative potential is limited, and their life span can vary from less than 1 day to many months, depending on their microenvironment, infections, and other stimuli. Although terminally differentiated, macrophages remain extremely active in messenger RNA and protein synthesis, with complex, often characteristic profiles of gene expression, depending on innate and acquired immune stimuli and cellular interactions. Tissue macrophages are relatively resistant to apoptosis, compared with neutrophils, but this feature changes during infection. Their active membrane turnover and endocytosis make them susceptible to toxic agents, making them targets for clearance by surviving macrophages. Sublethal injury and infection can also induce autophagy, increasingly recognized as an important component of inflammatory and infectious diseases.
The remarkable ability of macrophages to undergo homotypic cell–cell fusion results in giant cell formation. This is a feature of osteoclast differentiation, depending on M-CSF and the tumor necrosis factor (TNF) family member receptor activator of nuclear factor-κB ligand (RANKL), which act on monocytic precursors to yield catabolic cells able to excavate and remodel living bone. Local adhesion and ruffling of their plasma membrane are associated with focal, polarized release of H+ and hydrolytic enzymes by monocyte-derived osteoclasts. The attempted uptake of non- or poorly degradable foreign materials induces “foreign-body giant cells,” with distinct properties; macrophage-derived giant cells are also characteristic of granulomatous diseases such as tuberculosis (Langerhans giant cells; Fig. 68–3) and parasitic infections (e.g., schistosomiasis). Mycobacterial and ill-defined host lipids are able to induce giant cell formation in vitro. The mechanism of fusion involves cellular differentiation to induce a fusogenic phenotype and surface glycoprotein interactions with a selected substratum; T-helper type 2 (Th2) cytokines, such as IL-4 and IL-13, act through a common receptor chain and signaling pathway to enhance macrophage fusion.34 DNA synthesis, a feature of high-turnover granulomata associated with infection, can result in abortive cell division and cell death. These macrophage-derived giant cells are distinct from syncytia induced by fusogenic virus infection, especially paramyxoviruses and retroviruses such as the human immunodeficiency virus.
Monocytes are defined as the population of differentiated cells present in the circulation, with classical morphologic features (Chap. 67), and include the less-well defined precursors able to give rise to myeloid DCs and osteoclasts. Because of their ready availability from human blood and the sensitive methods now available to analyze their phenotype ex vivo (FACS, microarray, immunochemistry, and cytochemistry), human monocytes have been more amenable to study, whereas in the mouse, analysis of precursor–product relationship and tissue distribution have provided new insights into the fate and heterogeneity of the circulating population. The number of monocytes in the circulation depends on constitutive, steady-state production and delivery from marrow, possibly from marginated pools in spleen, as well as adhesion and diapedesis in response to unknown stimuli and enhanced recruitment in response to peripheral stimuli such as infection and inflammation. M-CSF and glucocorticoids affect their level and phenotype, as do metabolic stimuli; Chap. 70 describes clinical conditions that give rise to monocytosis. The biochemical properties and functions of monocytes are described in Chap. 67. They are relatively radioresistant once entering the circulation, where they persist for 12 to 48 hours as motile cells, with an ability to engulf particles and to adhere transiently or more stably to arterial as well as microvascular endothelium, thus modulating their phagocytic ability. Depending on interactions with the vessel wall and local differentiation, monocytes are able to crawl along and patrol the intravascular surface utilizing CD11a, a β2-integrin–dependent property.20 Mature macrophages lining the endothelium can also detach and recirculate, for example, filled with lipid stores as foam cells in atherosclerosis, and circulate heavily laden with erythroid breakdown products in malaria.
The presence of significant numbers of immune cells and molecules in adipose tissue suggest vibrant interactions between the immune and metabolic systems. In obesity, the inflammatory infiltrate and activation state of macrophages in adipose tissue may contribute to insulin resistance. The cellular localization and inflammatory potentials of macrophages,35 as well as the ratio of macrophages to adipocytes,36 differ in obese and lean mice. In lean mice, macrophages in the adipose tissue have the alternate or M2 phenotype (ARG1+CD206+CD301+), are uniformly distributed, and serve a protective function as they are less inflammatory and promote insulin sensitivity by producing IL-10; however, in obese mice, macrophages distribute around necrotic adipocytes, and induce inflammation and insulin resistance.35,37 CC-chemokine receptor 2 (CCR2) and its ligand (CCL2) are critical for macrophage recruitment to adipose tissue.38 Metabolic disease can be viewed as maladaptive consequence of inflammation-induced insulin resistance, which may beneficially conserve energy resources for the immune system combatting infection for brief periods.39,40,41,42,43
The precursors of myeloid DC and osteoclasts may represent a subpopulation of monocytes, whose further differentiation depends on cytokines and local factors in the vessel wall, marrow, and other tissues. Ex vivo substantial numbers of monocytes give rise to myeloid DC after treatment with GM-CSF and IL-4.32 Monocytes that differentiate into macrophages do not recirculate for the most part, but persist for varying times as “resident” tissue cells that turn over locally, especially in lymph nodes. It is not known if the constitutive exit from blood is a stochastic process or specific to particular tissues.
Phenotypic heterogeneity of monocyte populations has become a topic of intense interest, thanks to the availability of surface antigens/receptors such as CD14, CD16 (human), and Ly6C (mouse), and analysis of chemokine/receptor expression, especially fractalkine receptor (CX3CR) and CCR2.34 Figure 68–4 illustrates the subsets and tissue progeny established by the use of genetically manipulated mice and Table 68–1 compares expression of markers to characterize monocyte subsets in mouse and human blood. The relationship of monocyte precursor subsets that give rise to inflammatory tissue macrophages and DCs is better defined than is that of those that give rise to resident cells, which turn over more slowly. Current studies aim to elucidate the subset origin of other recruited populations, for example, in atherosclerosis, normal CNS, and tumors, and in response to metabolic, traumatic, or degenerative injury. Conceptually, it is still not clear how stable these apparently distinct subsets are or whether they represent part of a continuous phenotypic spectrum, arising by modulation of subpopulations rather than irreversible, true differentiation. Separation and microarray analysis of freshly isolated monocytes will yield further information regarding this question, providing novel markers and diagnostic signatures. Removal from an in vivo environment, as well as in vitro artifacts, can profoundly alter the phenotype and function of monocytes in such studies. Imaging and in situ analysis may enable single-cell direct studies of their fate.
Antigen | Human CD14hi CD16– | Human CD14+ CD16+ | Mouse CCR2+ CX3CR1low | Mouse CCR2– CX3CR1hi |
---|---|---|---|---|
CHEMOKINE RECEPTORS | ||||
CCR1 | + | – | ND | ND |
CCR2 | + | – | + | – |
CCR4 | + | – | ND | ND |
CCR5 | – | + | ND | ND |
CCR7 | + | – | ND | ND |
CXCR1 | + | – | ND | ND |
CXCR2 | + | – | ND | ND |
CXCR4 | + | ++ | ND | ND |
CX3CR1 | + | ++ | + | ++ |
OTHER RECEPTORS | ||||
CD4 | + | + | ND | ND |
CD11a | ND | ND | + | ++ |
CD11b | ++ | ++ | ++ | ++ |
CD11c | ++ | +++ | – | + |
CD14 | +++ | + | ND | ND |
CD31 | +++ | +++ | ++ | + |
CD32 | +++ | + | ND | ND |
CD33 | +++ | + | ND | ND |
CD43 | ND | ND | – | + |
CD49b | ND | ND | + | – |
CD62L | ++ | – | + | – |
CD86 | + | ++ | ND | ND |
CD115 | ++ | ++ | ++ | ++ |
CD116 | ++ | ++ | ++ | ++ |
F4/80 | ND | ND | + | + |
Ly6C | ND | ND | + | – |
7/4 | ND | ND | + | – |
MHC class II | + | ++ | – | – |
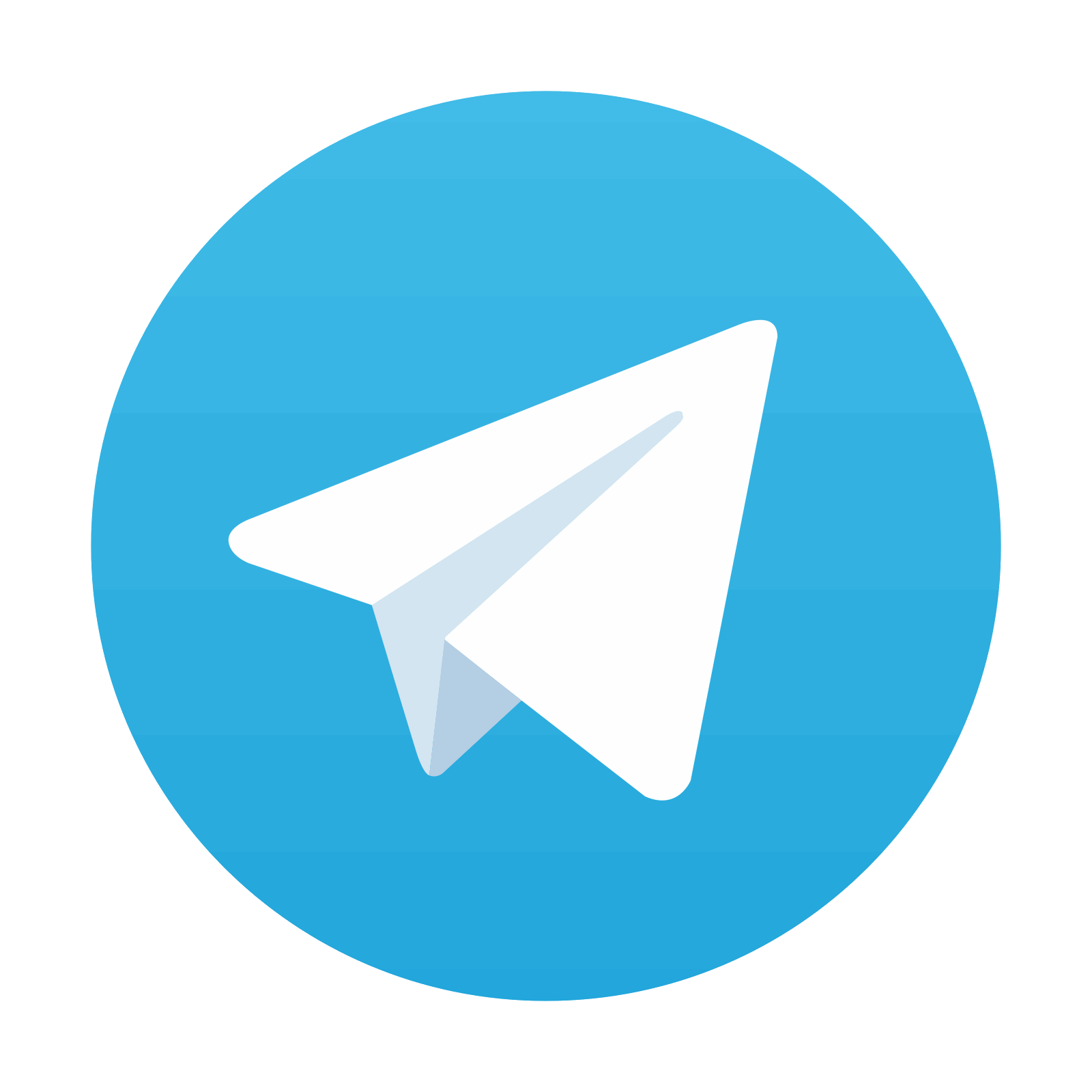
Stay updated, free articles. Join our Telegram channel

Full access? Get Clinical Tree
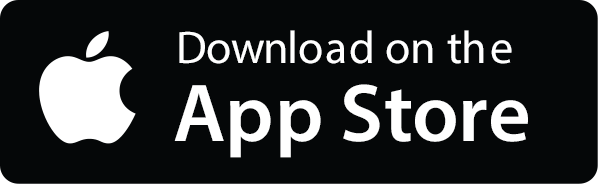
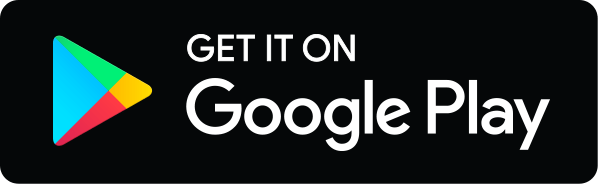