Key points
- •
The clinical application of radiation oncology balances the dual goals of improved tumor control with reduced treatment-related morbidity.
- •
Over time, technological and biological advances have offered many opportunities to further reduce normal tissue complications and improve tumor control with radiotherapy.
- •
More recent and emerging technologies promise further improvements in the therapeutic ratio.
- •
Rational application and critical appraisal of these new technologies will remain essential responsibilities for the field of radiation oncology.
Introduction
The discipline of radiation oncology involves the therapeutic application of ionizing radiation in the management of benign and malignant diseases. In fact, x-rays were used to treat a patient with ulcerated breast cancer less than 2 months after their discovery in November 1895. Therapeutic radiology, like its diagnostic counterpart, has undergone considerable evolution since then. Still, the practice of radiation oncology has at its core several fundamental concepts that have guided, and will continue to guide, management decisions.
Tumors and their neighboring organs will remain in proximity to one another. These tumors and their normal tissue counterparts will have varying responsiveness to ionizing radiation. Therefore, an ideal radiation treatment will balance optimal tumor control with acceptable acute side effects and late toxicities. Over time, new technological or biological advances will promise improved differentiation of these two populations, with better clinical outcomes. Some of these advances will actually work, shifting paradigms and reinforcing the ongoing need for rigorous testing before widespread adoption and changes to accepted standards of care.
With each new patient encounter, the radiation oncologist considers these concepts and determines the most appropriate treatment approach. A simplified algorithm is shown in Figure 4-1 , showing the general thought processes and decision points involved. The global decisions regarding treatment and goals of therapy are usually reached within a multidisciplinary context with other medical providers and informed consent of the patients and their support networks. Once the decision has been made to incorporate radiation therapy into the treatment plan, the more specific questions regarding timing, dose, treatment volume, and technology are considered. The solid and dashed arrows between the various boxes represent the interrelated nature of these questions and how they may each in turn influence the decision-making process.
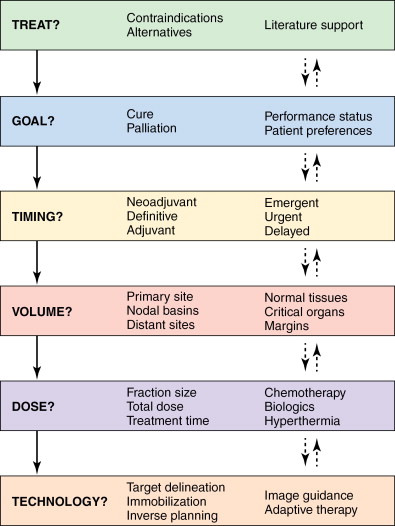
This chapter will briefly describe these concepts, along with specific clinical examples with various malignancies to illustrate the interplay between the decision points. We will focus on the therapeutic ratio, selection and definition of radiation target volumes, side effects and toxicity considerations, dose fractionation, and the impact of technological advances on these topics.
Therapeutic ratio
The overriding goal in patient care is to optimize treatment efficacy while minimizing toxicity. This concept is represented by the therapeutic ratio ( Figure 4-2 ). Depending on the tumor type and the surrounding normal tissues, a given amount of radiation dose will yield an expected range of both tumor control probability (TCP) and normal tissue complication probability (NTCP). The vertical white lines illustrate how an increase in dose (dashed line to solid line) leads to both higher tumor control and normal tissue complications. An example would be the dose escalation experience in prostate cancer. Multiple randomized trials have shown an improvement in prostate-specific antigen disease-free survival with higher doses of radiation at the cost of increased rectal and urinary complications.
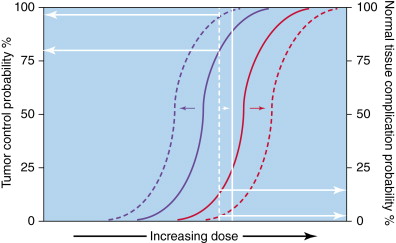
Ideally, the two curves can be further separated, with the same radiation dose providing extremely high TCP and low NTCP. Strategies that preferentially radiosensitize tumor cells and shift the curve left (dashed blue curve) may allow for improved TCP with the same physical dose. Alternatively, techniques that preferentially protect normal tissues (dashed red curve) may reduce treatment morbidity without compromising efficacy.
In clinical practice, agents that sensitize tumors often affect normal tissues as well, leading to improved outcomes at the cost of increased toxicity. Examples of this include the use of concurrent chemotherapy with radiation compared to radiation alone in the management of lung, head and neck, cervical, esophageal, and gastrointestinal cancers. At the same time, radioprotection agents have had less clinical impact than expected. For example, the compound amifostine is U.S. Food and Drug Administration (FDA)-approved for mitigation of xerostomia in head and neck cancer patients treated with radiation alone. In practice, very few centers now utilize this agent because of its cumbersome administration, its own toxicity profile, the superiority demonstrated of concurrent chemoradiation in head and neck cancer, and the subsequent development of new radiation technologies that better spare the parotid glands.
One example where technological advances may have improved the therapeutic ratio is the use of four-dimensional computed tomography simulation (4DCT) and intensity-modulated radiotherapy (IMRT) planning in locally advanced lung cancers. Simulation with 4DCT allows for capture of respiratory motion to avoid missing the tumor, whereas IMRT planning has the capacity to deliver more conformal dose distributions with reduction in high-dose normal tissue irradiation. In a retrospective series, the use of 4DCT/IMRT compared with conventional computed tomography (CT) and planning resulted in improvements in overall survival with decreased rates of radiation-induced pneumonitis.
Target volumes
When radiation oncologists discuss treatment targets and volumes ( Figure 4-3 ), they utilize definitions and acronyms developed by the International Commission of Radiation Units and Measurements (ICRU). The ICRU 50 report published in 1993 introduced the concepts of gross tumor volume (GTV), clinical target volume (CTV), and planning target volume (PTV). The GTV represents grossly visible disease, either clinically or radiographically. The CTV represents areas of potential microscopic extension of disease from the GTV. These microscopic deposits may be in proximity to the primary tumor and/or dispersed throughout the relevant draining regional lymph node basins. The PTV includes sufficient margin around the CTV to ensure adequate coverage and accounts for various uncertainties such as patient/organ motion and daily setup errors.
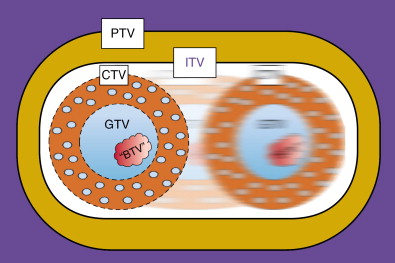
Technologic advances such as 4DCT, which captures organ motion, and immobilization devices/onboard imaging modalities that can reduce setup errors, have helped characterize and eliminate some of these uncertainties. A modern linear accelerator treatment machine with onboard imaging and respiratory motion tracking capabilities is shown in Figure 4-4 . The subsequent ICRU 62 report considered these advances with the addition of internal target volume (ITV) and setup margin (SM). Now, PTV = CTV + ITV + SM. Further advances in diagnostic radiology with functional imaging modalities are providing potential biologic target volumes (BTVs) to further improve the therapeutic ratio.
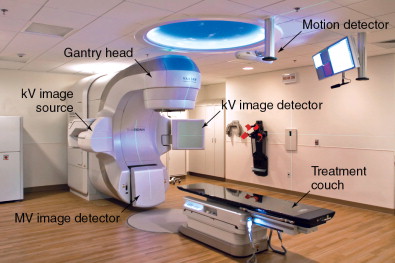
These target volume definitions help to determine how much technology and complexity is required to safely and effectively deliver the radiation treatments to optimize the therapeutic ratio. Depending on the clinical scenario, treatment plans may vary from relatively straightforward beam arrangements to highly complex delivery methods with rigid immobilization and onboard imaging modalities. For example, Figure 4-5 shows representative treatment fields used to treat a patient with locally advanced rectal cancer. Typically, the plan involves a posterior–anterior (PA) beam and two opposed lateral beams for coverage. Tumor volumes and normal tissues are shown contoured on a slice-by-slice basis and projected onto the digital reconstructed radiographs. The primary tumor and nodal GTVs are shown in red. The iliac blood vessels have been contoured in turquoise as surrogates for sites of potential microscopic disease in the pelvic lymph nodes. Small bowel (magenta) and femoral heads (tan and blue) are also outlined. Panel A (PA) and panel B (left lateral beam) demonstrate the additional margins on the GTV superiorly, inferiorly, and laterally to account for microscopic disease in the mesorectum and pelvic nodes (CTV) and additional margin for setup uncertainties (PTV). Organ motion considerations are often moot given the size of the treatment fields. Still, patients undergo fluoroscopic evaluation with rectal contrast before treatment to ensure the fields adequately cover the intended volumes.
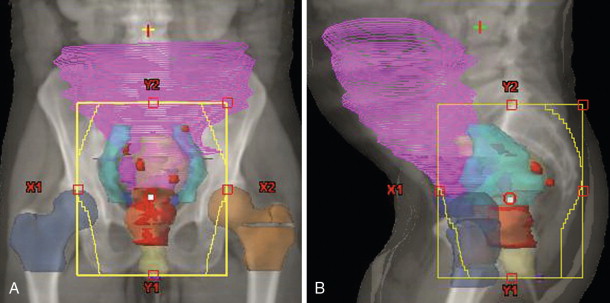
How do these target volumes fit into an algorithmic approach for rectal cancer as described in Figure 4-1 ? When the decision is made to treat a patient with locally advanced nonmetastatic rectal cancer, the goal is cure and generally involves therapy with surgery, radiation, and/or chemotherapy. Radiation therapy is often used in a neoadjuvant fashion, delivered preoperatively to the target volumes as outlined in Figure 4-5 , prior to surgical resection. The extensive literature on this subject has described multiple fractionation regimens, including 5 Gray (Gy) × 5 daily treatments to a total dose of 25 Gy with radiation alone. A more typical strategy employed at Duke involves 1.8 Gy daily fractions to a total dose of 50.4 Gy with concurrent chemotherapy. These volumes are large, with up to 2-cm margins in all directions to account for any organ motion and setup uncertainties.
The radiation doses are dictated by the tolerance of the small intestines and by the fact that radiation therapy plays an adjuvant role to reduce locoregional recurrences following the primary treatment modality, which is surgical resection. Microleaf collimators help to shield the small bowel and femoral heads. Of note, the patient was also treated in the prone position with a belly board designed to further minimize the amount of small bowel irradiated by the lateral fields. Oftentimes, simple maneuvers or changes in patient position during simulation and immobilization can facilitate treatments without the need for advanced planning and delivery techniques.
Side effects and toxicity
Although some clinical scenarios may not benefit from the utilization of advanced technological breakthroughs, the field of radiation oncology remains dedicated to the rational application of these emerging technologies for any potential gains in the therapeutic ratio. One such example may be found in the evolution of adjuvant radiation therapy in the management of breast cancer patients. Breast conservation with lumpectomy followed by whole breast radiotherapy remains the current standard, with radiation reducing the risk of recurrence by two-thirds compared to surgery alone.
Given the excellent survival and rates of cure, especially in early-stage breast cancer patients, there is growing awareness and emphasis on the potential late side effects of radiotherapy that may reduce the therapeutic ratio. In particular, patients with left-sided breast cancers often had anterior portions of the heart within the tangential radiation treatment portals. This excess heart irradiation likely contributed to the increased risk of late cardiac morbidity and mortality seen in breast cancer survivors. Subsequent techniques that tried to better shield the heart may have compromised target coverage and resulted in more recurrences under the heart blocks.
With the advent of respiratory motion tracking, many left-sided breast patients are now simulated using breath-hold techniques ( Figure 4-6 ). Panel A shows the merged CT images from a patient during both free-breathing and breath-hold at end-inspiration. Panel B shows the medial border of a typical tangential field for treatment of the whole breast. Portions of the heart can be seen within the treatment volume. In panel C, the heart has physically moved away from the chest wall, allowing the same tangent field to cover the breast while minimizing heart irradiation.
