Principles of Insulin Therapy
Alice Y. Y. Cheng
Bernard Zinman
HISTORICAL BACKGROUND
The isolation of insulin from dog pancreas and demonstration of its biologic effectiveness by Banting, Best, Collip, and MacLeod in 1921 at the University of Toronto represents one of the greatest medical discoveries of modern medicine (1). This antidiabetic substance, initially called isletin by Banting and Best and later named insulin by MacLeod, was purified sufficiently by Collip, the biochemist member of the team, so that it could be injected into humans (2). The first injection was given to a patient with diabetes, Leonard Thompson, on January 11, 1922, at the Toronto General Hospital (3). Improvements in insulin extraction and purification followed, facilitating the widespread use of insulin for patients with diabetes.
In 1936, Hagedorn discovered that the addition of fish protamine kept insulin in suspension so that it was absorbed slowly from subcutaneous sites, thus prolonging the effect of insulin (4). Scott and Fisher (5) discovered that zinc could further extend the action of protamine insulin, leading to the development of protamine zinc insulin. In 1946, NPH insulin (neutral protamine Hagedorn), a more stable form of protamine insulin, was introduced and remains in use today (6).
For the first 60 years of the insulin era, insulin was available only in bovine or porcine preparations. In the 1980s, human insulin was introduced (7), making animal insulin essentially obsolete. In the 1990s, insulin analogues with pharmacokinetics that were more appropriate for bolus (premeal) therapy were introduced and facilitated the improvement of subcutaneous insulin regimens (8). Over the last 80 years, great strides have been made to improve the treatment of diabetes with improved insulin formulations, increased ease of self-monitoring of blood glucose, and a better understanding of physiologic insulin requirements. Unfortunately, although we are much closer to the goal of physiologic insulin replacement, this goal remains elusive owing to the inherent limitations of administering insulin at a nonphysiologic site (subcutaneous tissue) (9).
TYPES OF INSULIN
The laboratory production of human insulin in the early 1980s has gradually resulted in the replacement of animal insulins as a viable therapeutic choice for patients with diabetes. Human insulins and newer insulin analogues produced by recombinant
DNA technology are becoming the main insulins used in the current treatment of diabetes in most countries. Insulins for clinical use can be characterized according to their pharmacokinetic profiles. They are available in rapid-acting, short-acting, intermediate-acting, and long-acting preparations (10). Table 39.1 shows the onset, peak, and duration of action after subcutaneous injections of the insulins used commonly in therapy. The different time-action profiles make it feasible to pursue the goal of simulating physiologic insulin secretion, as shown in Figure 39.1; however, this goal remains difficult to achieve with the current formulations. Insulin replacement should be thought of in terms of mealtime (bolus) and basal insulins. The mealtime insulins are the rapid-acting analogues or short-acting regular human insulin. These insulins have been used to attempt to simulate the high levels of insulin seen in individuals without diabetes after ingestion of a meal. The basal insulins are the intermediate- and long-acting human insulins and analogue. They simulate the basal level of insulin occurring between meals, through the night, and with fasting. Insulin is commercially available in concentrations of 100 or 500 units/mL, designated U-100 or U-500. The U-500 concentration, which is available only in short-acting formulations, is used only in rare cases of insulin resistance when the patient requires extremely large doses of insulin.
DNA technology are becoming the main insulins used in the current treatment of diabetes in most countries. Insulins for clinical use can be characterized according to their pharmacokinetic profiles. They are available in rapid-acting, short-acting, intermediate-acting, and long-acting preparations (10). Table 39.1 shows the onset, peak, and duration of action after subcutaneous injections of the insulins used commonly in therapy. The different time-action profiles make it feasible to pursue the goal of simulating physiologic insulin secretion, as shown in Figure 39.1; however, this goal remains difficult to achieve with the current formulations. Insulin replacement should be thought of in terms of mealtime (bolus) and basal insulins. The mealtime insulins are the rapid-acting analogues or short-acting regular human insulin. These insulins have been used to attempt to simulate the high levels of insulin seen in individuals without diabetes after ingestion of a meal. The basal insulins are the intermediate- and long-acting human insulins and analogue. They simulate the basal level of insulin occurring between meals, through the night, and with fasting. Insulin is commercially available in concentrations of 100 or 500 units/mL, designated U-100 or U-500. The U-500 concentration, which is available only in short-acting formulations, is used only in rare cases of insulin resistance when the patient requires extremely large doses of insulin.
TABLE 39.1. Approximate Pharmacokinetic Characteristics of Human Insulin and Insulin Analogues Following Subcutaneous Injection | |||||||||||||||||||||||||||||||||||||||||||||||||||||||||||||||||||||||||||||||||||||||||||||||
---|---|---|---|---|---|---|---|---|---|---|---|---|---|---|---|---|---|---|---|---|---|---|---|---|---|---|---|---|---|---|---|---|---|---|---|---|---|---|---|---|---|---|---|---|---|---|---|---|---|---|---|---|---|---|---|---|---|---|---|---|---|---|---|---|---|---|---|---|---|---|---|---|---|---|---|---|---|---|---|---|---|---|---|---|---|---|---|---|---|---|---|---|---|---|---|
|
![]() Figure 39.1. Normal insulin secretion in relation to meals and the overnight fasting state. (Redrawn from Owens DR, Zinman B, Bolli GB: Insulins today and beyond. Lancet 2001; 358:739.) |
Mealtime Insulins
RAPID-ACTING INSULIN ANALOGUES: LISPRO AND ASPART
The time-action profile of regular human insulin is unable to adequately mimic physiologic insulin secretion. Insulin in solution self-associates and forms larger aggregates called hexamers. These large aggregates need to dissociate after subcutaneous injection before diffusion of insulin into the circulation is possible (11). Therefore, analogues of human insulin have been developed that can dissociate rapidly from hexamers to monomers or that remain less associated in solution, thus allowing faster absorption and onset of action (10,11). The first rapidly acting insulin analogue approved for human administration was insulin lispro, in which the terminal proline and lysine residues of the B chain of insulin are inverted, resulting in decreased self-association properties of the insulin (8,12). Lispro insulin is absorbed much more rapidly than regular insulin. Lispro begins acting within 15 minutes, reaches peak biologic effects in 60 to 90 minutes, and continues to act for 4 to 5 hours. In comparison to regular insulin, lispro peaks more rapidly, achieves higher blood insulin concentrations more rapidly, and has been shown to lower postprandial glucose levels and decrease rates of hypoglycemia (13,14,15,16,17). The impact of lispro compared with regular insulin on levels of glycosylated hemoglobin (HbA1c) has been variable (18,19,20,21,22,23). However, lispro provides more flexibility for patients at mealtimes because it does not need to be administered 30 minutes before a meal and the dose can readily be adjusted for changes in the carbohydrate content of the meal. Randomized controlled prospective trials have demonstrated that lispro is one of the preferred insulins for insulin pump therapy with continuous subcutaneous insulin infusion (CSII) and results in lower HbA1c levels and less hypoglycemia (24,25,26). Lispro must be used with caution in patients with gastroparesis because of its rapid onset of
action and the potential for early postprandial hypoglycemia. Patients with gastroparesis can delay the meal insulin injection until after they have consumed the meal to reduce the risk of hypoglycemia.
action and the potential for early postprandial hypoglycemia. Patients with gastroparesis can delay the meal insulin injection until after they have consumed the meal to reduce the risk of hypoglycemia.
Insulin aspart is another very-rapid-acting insulin analogue. Similar to lispro, it is absorbed very rapidly after subcutaneous injection, resulting in higher peak concentrations compared with those achieved with regular insulin (27). Trials comparing insulin aspart and regular insulin in patients with type 1 diabetes show significantly reduced HbA1c levels with no difference in the frequency of hypoglycemia (28,29,30). In type 2 diabetes, insulin aspart is comparable to regular insulin, with no significant difference in HbA1c levels or frequency of hypoglycemia (31). Both insulin lispro and insulin aspart appear to be associated with a lower incidence of nocturnal hypoglycemia than regular human insulin. For these reasons, lispro and aspart are the insulins of choice for use before meals in most treatment regimens and particularly in multiple daily injection therapy (13,14,15,16,17,19,20,21,23,28,29,30). The safety and efficacy of the use of aspart in CSII have also been demonstrated (31a).
SHORT-ACTING HUMAN INSULIN: REGULAR
As mentioned earlier, subcutaneously injected regular insulin, because of its relatively slow rate of absorption, is unable to mimic physiologic insulin secretion following a meal. Regular insulin has an onset of action 15 to 60 minutes after injection, a peak effect 2 to 4 hours after injection, and a duration of action ranging from 5 to 8 hours (11). Given the “short-acting” nature of regular insulin, it is used primarily as a mealtime insulin. Therefore, regular insulin should be administered approximately 30 to 45 minutes before a meal in order to match the kinetics of insulin absorption with the peak of carbohydrate absorption after the meal (11).
Basal Insulins
INTERMEDIATE-ACTING HUMAN INSULINS: NPH AND LENTE
Both NPH and lente insulins are modified into a suspension form to delay their absorption from subcutaneous sites, thereby prolonging their action. NPH insulin, first developed in 1946, has a longer duration of action than regular insulin (12). Its onset of action is within 2.5 to 3 hours of injection, with a peak action 5 to 7 hours after injection and a duration of action between 13 and 16 hours (12). Lente insulin, the other intermediate-acting insulin, was first introduced in the 1950s. Its onset of action is similar to that of NPH at 2.5 hours, its peak action is 7 to 12 hours after injection, and its duration of action is up to 18 hours (12). Given the more prolonged action of NPH and lente insulins, they are not ideal for controlling postprandial serum glucose levels. When given at appropriate times, these insulins are reasonably effective in lowering fasting plasma glucose and predinner plasma glucose levels (11). These insulins are used as the basal insulins and are necessary for adequate glycemic control in type 1 and type 2 diabetes. Currently, NPH insulin is the only intermediate-acting insulin available for the pen delivery system.
LONG-ACTING HUMAN INSULIN: ULTRALENTE
The role of long-acting insulin is to achieve basal insulin coverage with a relatively small or no pharmacologic/biologic peak action. Its onset of action is 4 hours after injection, its peak action is at 8 to 10 hours, and its duration of action is up to 20 hours (12). In clinical practice, its biologic action appears to be similar to that of intermediate-acting insulin. NPH and ultralente provide similar glycemic control when used as the basal insulin in a multiple daily injection regimen with lispro as the mealtime insulin (32). Most patients (75%) require only one injection of basal insulin per day given at bedtime (32). However, NPH seems to provide a slightly better daily glycemic profile than ultralente in those requiring two injections of basal insulin per day (32).
LONG-ACTING INSULIN ANALOGUE: GLARGINE
Glargine is a long-acting insulin analogue that was developed in an attempt to provide a more constant level of insulin than that achieved with the human intermediate- and long-acting insulin preparations (10,33,34,35,36,37,38). Glargine has a higher isoelectric point than human insulin and precipitates in the neutral environment of subcutaneous tissue, giving it its prolonged duration of action (10,33,34,35,36). Glargine is absorbed more slowly than the human long-acting insulin preparations, with no pronounced insulin peaks, decreasing the risk of nocturnal hypoglycemia (33,34,36,37,38). Insulin glargine has a greater affinity than human insulin for insulin-like growth factor 1 (IGF-1) receptors, and it has been suggested that this may lead to increased mitogenic potential in cell lines rich with IGF-1 receptors (39). However, the clinical significance of this hypothesis is still unknown (39).
Detemir is another long-acting insulin analogue that was developed using a different approach—binding to albumin. An aliphatic fatty acid has been acylated to the B29 amino acid, and the B30 amino acid has been removed (39a). This results in reversible binding between albumin and the fatty acid acylated to the insulin. After injection, 98% of the insulin is bound to albumin. The gradual release of the bound fraction from albumin allows for the sustained, prolonged action of detemir (39b). The time-action profile of insulin detemir is characterized by a peak activity at 6 to 8 hours after injection and prolonged 24- hour duration of action (39b). Detemir has been approved for release in Europe and has received preliminary approval from the FDA in the United States. Studies to date have shown less hypoglycemia and less variation in blood glucose levels with detemir as basal insulin in intensive regimens compared with NPH (39c,39d,39e).
Premixed Insulin
Premixed preparations of short- and intermediate-acting insulins are available in a wide range of ratios (90/10 to 50/50) (11). The most commonly used premixed insulin is 70/30, which contains 30% short-acting and 70% intermediate-acting insulin. A premixed insulin of 75% NPL (neutral protamine lispro) and 25% lispro, Humalog Mix 75/25, also is available (40). Humalog Mix 75/25 provides a relatively rapid peak in insulin activity, similar to lispro alone, and the NPL provides basal coverage similar to that of NPH (40). This allows improved postprandial glycemic control compared with that offered by 70/30 insulin (41,42). A premixed insulin of 30% aspart and 70% protaminated insulin aspart (NovoLog Mix 70/30) is also available. Like Humalog Mix 75/25, NovoLog Mix 70/30 provides improved postprandial glucose control and less hypoglycemia than 70/30 insulin (42a,b).
Use of premixed insulin avoids the potential problems of self-mixing and reduces the number of steps before injection, thereby reducing the number of possible errors. The premixed insulins are preferred by elderly patients and those with visual or fine-motor impairment (43). However, premixed insulins do not permit easy adjustment of mealtime and basal insulin doses and are inappropriate for patients with type 1 diabetes. They are valuable and frequently used in the treatment of type 2 diabetes.
GOALS OF THERAPY
Type 1 Diabetes
Insulin deficiency is the hallmark of type 1 diabetes. Insulin replacement is essential for life in people with type 1 diabetes to avoid a progressive catabolic state and ketosis. When used properly, insulin can eliminate clinical symptoms of hyperglycemia, prevent diabetic ketoacidosis, restore lean body mass and exercise capacity, decrease the incidence of certain infections, and improve the patient’s sense of well-being. In addition, the use of insulin in intensive treatment regimens, with the goal of achieving near-normal plasma glucose concentrations, delays the onset and slows the progression of microvascular complications in patients with type 1 diabetes (44,45). Table 39.2 itemizes some of the principal goals of insulin therapy. The Diabetes Control and Complications Trial (DCCT) was a multicenter, randomized controlled trial comparing multiple daily injection therapy or continuous subcutaneous insulin infusion (intensive therapy) to therapy with one to two injections per day (conventional therapy) in 1,441 patients with type 1 diabetes, followed for a mean of 6.5 years (44). The intensive-therapy group, who achieved significantly lower HbA1c levels than patients in the conventional-therapy groups, had a relative risk reduction of 76% for developing retinopathy and a 54% relative risk reduction for progression of retinopathy (44). The intensive-therapy groups had a 39% relative risk reduction for developing microalbuminuria and a 54% relative risk reduction for progressing to albuminuria (44). The intensive-therapy group also had a 64% relative risk reduction for developing neuropathy or experiencing progression of diagnosed neuropathy (46). Thus, intensive insulin therapy, which resulted in a lower HbA1c level, was associated with a statistically and clinically significant reduction in microvascular complications in the intensive-therapy group. A follow-up study of the DCCT group, the Epidemiology of Diabetes Intervention and Complications (EDIC) study, showed that the risk reduction in microvascular complications from intensive therapy persists for at least 4 years despite increasing hyperglycemia (47). However, the benefit related to macrovascular complications is less clear (48). The DCCT showed a nonstatistically significant 41% relative risk reduction for macrovascular complications with intensive therapy; however, the study was not powered to detect a difference in macrovascular events, and the number of these events in the trial was low (49). There was also a relative risk reduction of 40% for developing elevated low-density lipoprotein (LDL) cholesterol levels in the intensive-therapy group (49). A recent meta-analysis of intensive therapy in type 1 diabetes showed that intensive therapy decreased the total number of macrovascular events but showed no significant effect on the number of patients affected or on mortality due to macrovascular disease (48). The need for insulin in type 1 diabetes for survival is apparent. However, the appropriate use of insulin with the goal of achieving near-normal serum glucose concentrations has benefits beyond those of mere survival and symptom relief.
TABLE 39.2. Goals of Insulin Therapy | |
---|---|
|
The degree of glucose lowering to be sought depends on many factors and should be individualized for each patient. In the DCCT, the mean HbA1c level achieved by the intensive-therapy group was approximately 7.2% compared with a mean of 9.0% in the conventional-therapy group (44). Therefore, the most recent clinical practice recommendations from the American Diabetes Association state that the primary treatment goal in type 1 diabetes should be blood glucose control approximating the median value achieved in the intensive-therapy group (50). Table 39.3 outlines the glycemic goals from the American Diabetes Association. In fact, any improvement in blood glucose control will slow the development and progression of microvascular complications. However, one must consider the risks of hypoglycemia when aiming for a low HbA1c level. The intensive-treatment group in the DCCT had a threefold greater risk of severe hypoglycemia compared with the conventional-therapy group, but the rate of hypoglycemia decreased with time (44). Therefore, very tight control should not be attempted in patients unwilling or unable to participate actively in their glucose management. Patients with hypoglycemia unawareness or those susceptible to permanent injury from hypoglycemia, such as children or the elderly, can be managed with intensive therapy with multiple daily injections (MDI) or CSII but should have higher glycemic targets to avoid hypoglycemia (51,52,53). Appropriate diabetes education and self-monitoring of blood glucose are invaluable components of reducing hypoglycemia (51,53,54). Clinical judgment and common sense are required to determine the target premeal, postprandial, and
bedtime glucose levels for individual patients without placing them at undue risk for hypoglycemia (50,51,53,54).
bedtime glucose levels for individual patients without placing them at undue risk for hypoglycemia (50,51,53,54).
TABLE 39.3. Target Glycemic Control for Nonpregnant Adults with Diabetes | ||||||||||||||||||||||||||||||||||||||||||||||||
---|---|---|---|---|---|---|---|---|---|---|---|---|---|---|---|---|---|---|---|---|---|---|---|---|---|---|---|---|---|---|---|---|---|---|---|---|---|---|---|---|---|---|---|---|---|---|---|---|
|
Type 2 Diabetes
The initial treatment for type 2 diabetes generally does not include insulin. Diet, exercise, weight loss, and oral hypoglycemic agents are initially adequate therapies for achieving glycemic control. However, insulin is indicated for patients who are unable to achieve good glycemic control with a combination of oral agents, diet, and exercise (55). The goals of insulin therapy in type 2 diabetes are similar to those for type 1 diabetes. The elimination of clinical symptoms of hyperglycemia is an important goal. In addition, maintaining good glycemic control reduces the risk of microvascular and macrovascular complications. The United Kingdom Prospective Diabetes Study (UKPDS) was a multicenter, randomized, controlled study designed to establish whether intensive blood glucose control reduced the risk of macrovascular and microvascular complications in patients with type 2 diabetes (56) and to compare the relative effectiveness and safety of different pharmacologic approaches to therapy. The UKPDS studied 5,102 patients with type 2 diabetes and followed them for an average of 10 years (56). There was a 12% reduction in diabetes-related endpoints and a 25% risk reduction in microvascular endpoints for the intensive-therapy group (56). There was no significant difference within the intensive-therapy group (i.e., no difference between insulin and sulfonylureas). A smaller Japanese study also showed significant reductions in microvascular complications with improved HbA1c levels (57). Although the original analysis of the UKPDS data showed a nonstatistically significant 16% risk reduction (p = 0.052) in macrovascular complications with intensive therapy (56), a recent analysis showed a significant relationship between macrovascular complications and hyperglycemia, as measured by the updated mean HbA1c (58,59). For every 1% reduction in updated mean HbA1c, there was a 14% risk reduction for myocardial infarction (p < 0.0001), a 12% risk reduction for stroke (p = 0.035), and a 43% risk reduction for amputation or death from peripheral vascular disease (p < 0.0001) (58).
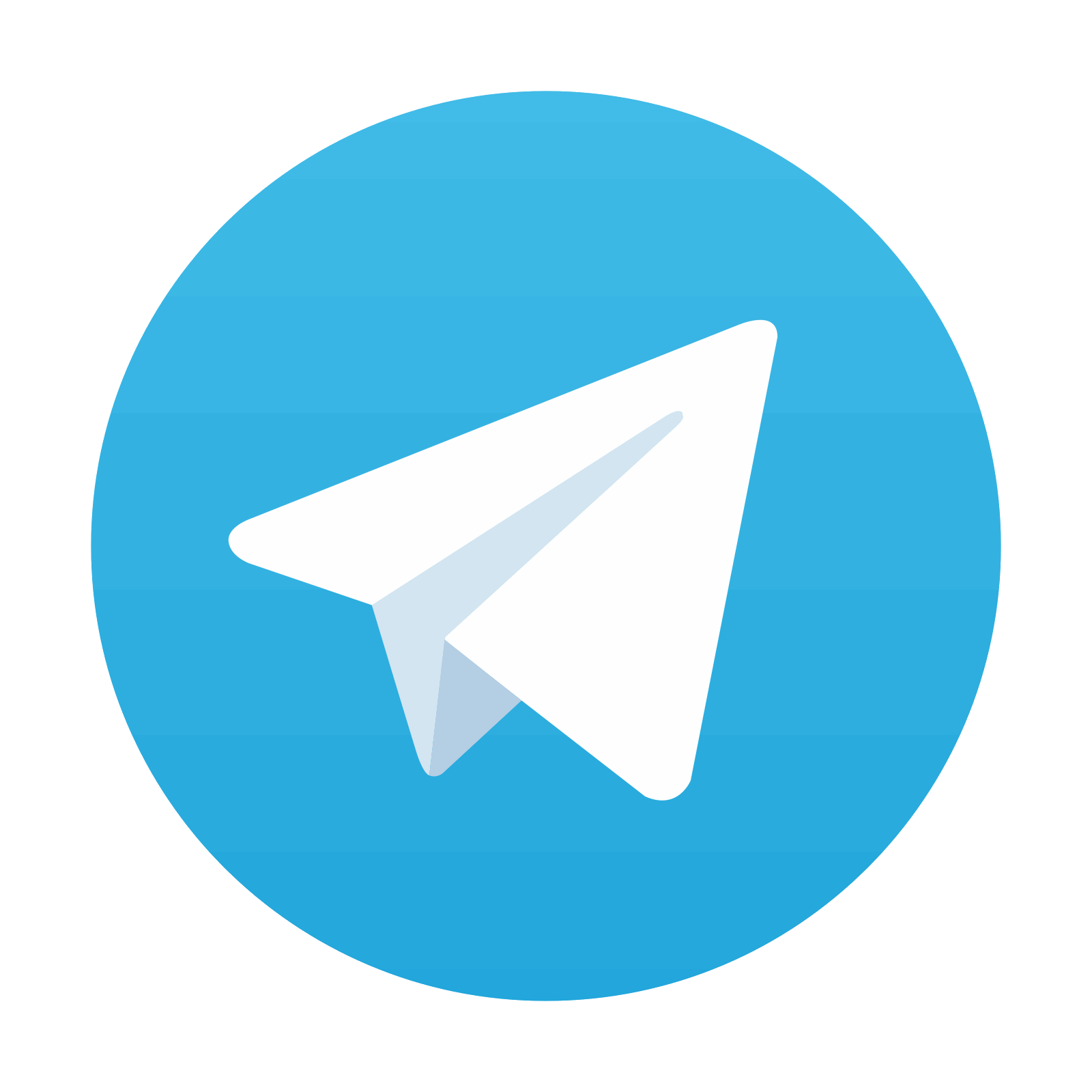
Stay updated, free articles. Join our Telegram channel

Full access? Get Clinical Tree
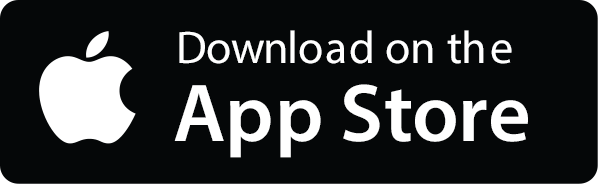
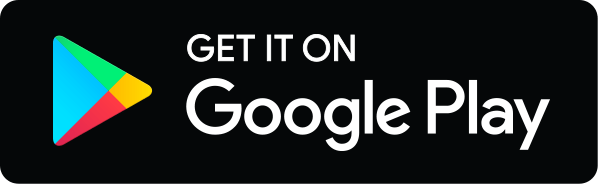