INTRODUCTION
SUMMARY
Antithrombotic drugs are among the most commonly used drugs in medicine and are generally separated into anticoagulants, fibrinolytic agents, and platelet inhibitors based on their primary mechanism of action. For many decades, warfarin, which acts by inhibiting vitamin K action, was the only oral anticoagulant available. Vitamin K antagonists have a prolonged effect, unpredictable pharmacokinetics, and require monitoring, but warfarin was widely used for prevention and treatment. The introduction of novel targeted oral anticoagulants has changed the landscape of anticoagulation. Rivaroxaban, apixaban, and edoxaban are novel oral inhibitors of factor Xa, whereas dabigatran is an orally available inhibitor of thrombin. Unfractionated heparin and the low-molecular-weight heparins are the most commonly used rapidly acting parenteral anticoagulants; they inhibit activated serine proteases through antithrombin. One synthetic agent in this class, fondaparinux, is specific for inhibition of factor Xa, and is effective for prevention and treatment of venous thromboembolism. Several parenteral direct thrombin inhibitors have excellent anticoagulant action and offer an alternative to heparins. A number of fibrinolytic agents are available, all of which convert plasminogen to plasmin to accelerate clot lysis. Differences among them include their degree of fibrin specificity, half-life, and antigenicity. Antiplatelet agents play an important role in prevention and treatment of arterial thrombosis. Aspirin is a cyclooxgenase-1 inhibitor that is effective and widely used in the prevention of stroke and myocardial infarction. Drugs that modulate cyclic adenosine monophosphate levels include dipyridamole, pentoxifylline, and cilostazol, and are primarily used in treatment of peripheral vascular disease. Adenosine diphosphate receptor blockers such as ticlopidine, clopidogrel, and prasugrel are effective in treatment of coronary and peripheral arterial disease. Examples of inhibitors of fibrinogen interaction with αIIbβ3 are abciximab, tirofiban, and eptifibatide. These drugs are highly effective in treatment of patients with acute coronary syndromes.
Acronyms and Abbreviations:
ACT, activated clotting time; ADP, adenosine diphosphate; aPTT, activated partial thromboplastin time; cAMP, cyclic adenosine monophosphate; COX, cyclooxygenase; CYP, cytochrome P450; DVT, deep vein thrombosis; FFP, fresh-frozen plasma; Gla, γ-carboxyglutamic acid; HIT, heparin-induced thrombocytopenia; INR, international normalized ratio; ISI, international sensitivity index; LMWH, low-molecular-weight heparin; MI, myocardial infarction; NSAID, nonsteroidal antiinflammatory drug; PE, pulmonary embolism; PG, prostaglandin; PGI2, prostacyclin; PRP, platelet-rich plasma; PT, prothrombin time; TNK, tenecteplase; t-PA, tissue-type plasminogen activator; VTE, venous thromboembolism.
Antithrombotic agents are highly effective and are among the most commonly used drugs in medicine because thrombotic diseases are the leading cause of mortality and morbidity in Western countries. Antithrombotic agents are characterized separately as anticoagulants, antiplatelet agents, or fibrinolytic drugs, depending on their primary mechanism, although there is overlap in their activities and clinical indications (Table 25–1). Their greatest use is in prevention of thrombosis in patients at high risk, but they also have important applications for treating acute thrombosis. For many agents, the risk-to-benefit ratio is narrow, with the result that bleeding complications occur, and bleeding is the most common adverse effect. Consequently, the clinician should carefully weigh the risks and benefits for each patient when selecting treatment. Generally, these drugs do not cause bleeding by themselves; instead, they exacerbate preexisting bleeding or predispose to bleeding from pathologic lesions that may be found in the gastrointestinal or genitourinary tracts or central nervous system. A careful review of comorbid conditions that may increase bleeding risk is important when deciding on therapy.
Anticoagulant therapy acts to decrease fibrin formation by inhibiting the formation and action of thrombin, and its most common uses are in preventing systemic embolization in patients with atrial fibrillation and for secondary prevention of venous thromboembolism. Anticoagulant therapy is sometimes monitored using coagulation testing because of marked biologic variation in effect with some agents. Antiplatelet agents act to inhibit platelet function, and their primary uses are in preventing thrombotic complications of cerebrovascular and coronary artery disease. They also have a role in treatment of acute myocardial infarction and some effect in preventing venous thrombosis. Fibrinolytic agents accelerate lysis of thrombi by increasing conversion of plasminogen to plasmin, and are primarily used in the acute management of myocardial infarction, in clearing occluded catheters and also in selected patients with stroke or venous thromboembolism. Fibrinolytic therapy is associated with a higher risk of bleeding complications than treatment with either anticoagulants or antiplatelet agents. Treatment of acute thrombosis often involves combinations of agents with multiple actions for maximum effect.
Antithrombotic therapy has changed considerably with the introduction of several novel target-specific oral anticoagulants. These drugs offer more predictable pharmacokinetics and fewer drug interactions than warfarin, thus eliminating the need for frequent monitoring. These agents have been studied in numerous phase III randomized clinical trials that have compared these agents to standard anticoagulation therapies. In most cases, the new anticoagulants are equivalent to conventional anticoagulation in reducing thrombotic events, while in many cases they are associated with lower rates of major bleeding complications.
Anticoagulants—decrease fibrin formation by inhibiting thrombin or thrombin formation Agents Oral—warfarin and other vitamin K antagonists, dabigatran (direct thrombin inhibitor) and oral direct Xa inhibitors (rivaroxaban, apixaban, edoxaban) Parenteral—heparin, low-molecular-weight heparins, fondaparinux, direct thrombin inhibitors (argatroban, desirudin, bivalirudin) Antiplatelet agents—inhibit platelet function Agents Aspirin, clopidogrel, prasugrel, dipyridamole, abciximab, eptifibatide, tirofiban, vorapaxar Fibrinolytic agents—plasminogen activators and accelerate clot lysis Agents Streptokinase, urokinase, alteplase, reteplase, tenecteplase |
VITAMIN K ANTAGONISTS
The development of vitamin K antagonists as oral anticoagulants began in the 1920s with investigation of a hemorrhagic disease in cattle, the cause of which was eventually traced to ingestion of moldy hay leading to hypoprothrombinemia.1 A coumarin that inhibited vitamin K was purified and eventually introduced into clinical practice in the 1940s. Several coumarin derivatives with differing pharmacologic properties are now available as anticoagulants worldwide, and are collectively referred to as vitamin K antagonists, but warfarin is nearly universally used in North America. These agents are widely used to prevent or treat common thrombotic diseases and represent the most commonly used oral anticoagulant currently available.2
The coumarins are competitive inhibitors of vitamin K. They inhibit γ-carboxylation reactions required for synthesis of several coagulation proteins, including factors II, VII, IX, and X, as well as proteins C and S, which are involved in inhibitory regulation of hemostasis. The synthesis of these proteins requires a posttranslational modification of several glutamic acid residues, converting them to γ-carboxylated glutamic acid (Gla), which is required for proper membrane interaction and biologic activity (Chap. 115).3,4 The carboxylation reaction requires reduced vitamin K, which is converted to vitamin K epoxide in the reaction. Vitamin K epoxide subsequently undergoes reduction by an enzyme that is inhibited by warfarin.5,6,7 Therefore, treatment with warfarin causes reduced γ-carboxylation, leading to synthesis of molecules with impaired activity.8,9,10
Warfarin preparations consist of a racemic mixture of S and R enantiomers in approximately equal proportion in an oral formulation with high bioavailability. Warfarin is water soluble and rapidly absorbed after oral administration, reaching a peak concentration after 60 to 90 minutes. An intravenous preparation is also available for patients who cannot take oral medications or who have malabsorption. It is tightly bound to plasma proteins with a half-life of 35 to 45 hours, with only the free, nonbound form having biologic activity. Warfarin is metabolized through the cytochrome P450 (CYP) system, the activity of which is influenced by environmental factors and also by genetic polymorphisms that alter the structure of common enzymes. Other vitamin K antagonists have similar activities but exhibit differences in absorption and elimination.
Because warfarin is a vitamin K antagonist, its action is influenced by the vitamin K content of the diet. Naturally occurring vitamin K is found in a variety of vegetables, and changes in diet can affect the vitamin K availability and warfarin effect.11 This may be seen particularly in patients receiving warfarin who are on strict weight-reduction diets or in those with little oral intake because of illness. Also, diarrhea can affect vitamin K availability, as can administration of broad-spectrum antibiotics leading to marked warfarin sensitivity in hospitalized patients. Ingestion of vitamin K in dietary supplements or vitamins also affects sensitivity to warfarin. Liver disease can increase sensitivity to warfarin because of impaired synthesis of coagulation factors, and hyper- or hypometabolic states may also alter sensitivity. Hereditary resistance to warfarin has been described and related to specific mutations in vitamin K epoxide reductase.12 Many drug interactions can influence the pharmacodynamics of warfarin by altering synthesis or clearance of vitamin K–dependent coagulation factors or interfering with warfarin metabolism, and patients should be advised to consult their physician or pharmacist about effects on anticoagulation when changing drug therapy or starting new medications (Table 25–2).11 Other commonly used drugs affecting hemostasis, such as aspirin, nonsteroidal antiinflammatory agents, heparins, and other anticoagulants can add to the antihemostatic effects of warfarin and can lead to bleeding.
POTENTIATE EFFECT | |
---|---|
α-Methyldopa | Indomethacin |
Acetaminophen | Isoniazid |
Acetohexamide | Mefenamic acid |
Allopurinol | Methimazole |
Androgenic and anabolic steroids | Methotrexate |
Antibiotics that disrupt intestinal flora (tetracyclines, streptomycin, erythromycin, kanamycin, nalidixic acid, neomycin) | Methylphenidate |
Nalidixic acid | |
Nortriptyline | |
Oxyphenbutazone | |
Cephaloridine | p-Aminosalicylic acid |
Chloral hydrate | Paromomycin |
Chloramphenicol | Phenylbutazone |
Chlorpromazine | Phenyramidol |
Chlorpropamide | Phenytoin |
Cimetidine | Propylthiouracil |
Clofibrate | Quinidine |
Diazoxide | Salicylate |
Disulfiram | Sulfinpyrazone |
Ethacrynic acid | Sulfonamides |
Glucagon | Thyroid hormone |
Guanethidine | Tolbutamide |
DEPRESS EFFECT | |
Antipyrine | Glutethimide |
Azathioprine | Griseofulvin |
Barbiturates | Haloperidol |
Carbamazepine | Phenobarbital |
Digitalis | Prednisone |
Ethanol | Rifampin |
Ethchlorvynol | Vitamin K |
The anticoagulant effect of warfarin is the result of decreased levels of vitamin K–dependent coagulation factors, and their concentration represents a balance of synthesis and metabolism. Warfarin administration impairs synthesis, and levels of vitamin K–dependent factors fall in relation to their metabolism. This is short for factor VII, with a half-life of approximately 5 hours, but longer for factors X and IX (t1/2 = 24 hours) and longest for factor II (prothrombin) with a half-life of approximately 72 hours. The desired anticoagulant effect results from a balanced reduction of all factors and requires several days to achieve. Imbalances in reduction of coagulation factors may occur during initiation of therapy as factor VII level falls rapidly, whereas others, especially factor II, decline more slowly. The initial rapid fall in factor VII level may lead to an early elevation in the prothrombin time (PT) expressed as international normalized ratio (INR) without reflecting the desired anticoagulant effect. Because protein C is a natural inhibitor of coagulation with a short half-life (approximately 8 hours), its level may fall rapidly, theoretically inducing a procoagulant state during initiation of therapy.
As a result of the delayed anticoagulant effect of warfarin, therapy is initiated with a rapidly acting agent, such as heparin or low-molecular-weight heparin (LMWH), if immediate anticoagulation is needed. For example, patients with venous thromboembolism are typically given heparin or an LMWH for rapid effect, and warfarin is also administered within the first 24 hours. After a period of 5 or more days, the necessary anticoagulant effect of warfarin is achieved, and the parenteral anticoagulant can be stopped. Anticoagulation is initiated with a dose close to the expected daily maintenance requirement, which is usually between 5 and 10 mg.13,14,15 There is, however, great variability in the doses required, and smaller amounts should be used for frail, elderly, or poorly nourished patients, or those with an increased bleeding risk. In patients with a low level of protein C or protein S as a result of an inherited deficiency, initiation of warfarin therapy without concomitant heparin or other immediately acting anticoagulant can lead to very low levels of these natural anticoagulants with ensuing thrombosis such as skin necrosis.
The anticoagulant effect of the vitamin K antagonists is monitored using the PT, which is sensitive to decreases in vitamin K–dependent factors and is progressively lengthened as the vitamin K–dependent factors reach lower levels. A critical component of the PT is the thromboplastin reagent that is used. Variability in thromboplastin composition leads to variation in results. The widespread introduction of the INR has improved standardization of results.16 Manufacturers determine the potency of thromboplastins by measuring the international sensitivity index (ISI), and this is used as a correction factor for the responsiveness of the thromboplastin in the PT. The INR represents the ratio of the patient PT to control PT corrected by the ISI. By this method, INR values obtained in different laboratories can be reliably compared for therapeutic monitoring.
During initiation of therapy, the INR is checked every 2 to 3 days for 1 to 2 weeks until a stable therapeutic effect is achieved. The target INR for most indications is 2.5 with a desirable therapeutic range from 2 to 3. A higher INR is recommended for patients with mechanical heart valve replacement and for those who failed anticoagulant therapy despite well-documented INR values in the 2 to 3 range. During chronic therapy, the INR should be monitored regularly, depending on stability of the response, and minor dose adjustments are frequently needed. A recent randomized trial in patients on chronic warfarin therapy with a stable dose for 6 months demonstrated that INR monitoring every 12 weeks was noninferior to monitoring every 4 weeks with regards to time in therapeutic range (74.1 vs. 71.6 percent).17 Monitoring can also be performed using portable instruments that are suitable for home use, enabling selected patients to learn to modify their warfarin doses in response to their INR value.18 A nonblinded randomized study compared home monitoring to INR monitoring in a specialized coagulation clinic in a cohort of patients on chronic warfarin and capable of conducting home monitoring.19 In this trial, rates of the primary composite end point (thrombosis, major bleeding or death) were equivalent, but home monitoring was associated with significant improvement in patient satisfaction, quality of life and time in therapeutic range. Specialized clinics devoted to monitoring warfarin typically achieve better results in maintaining patients within the therapeutic range, resulting in fewer bleeding complications.20,21 Problems with keeping patients within the therapeutic range often result from failure of compliance, changes in diet, medication or alcohol intake, or intercurrent illnesses.
Warfarin sensitivity is affected by polymorphisms in CYP and vitamin K epoxide reductase complex (VKORC), and pharmacogenomics may become important in dosing. The clearance of warfarin is the result of hepatic metabolism and CYP2C9 is the most important enzyme mediating its clearance.22 A number of polymorphisms have been identified, but the most important are CYP2C9*2 and CYP2C9*3, which are found in approximately 11 percent and 7 percent of patients and result in reductions of enzymatic activity of approximately 30 percent and 80 percent, respectively.23,24,25 This reduced metabolic clearance leads to increased drug levels and an increased anticoagulant effect. VKORC1 converts oxidized vitamin K to the active reduced form as required for posttranslational carboxylation. VKORC1 is the target of warfarin, which functions as a competitive inhibitor. Numerous coding polymorphisms have been identified that can affect the response to warfarin.26,27 Common haplotypes can be separated into low-dose (A) and high-dose (B) groups with different sensitivities to warfarin.
Evidence is clear that polymorphisms in either CYP2C9 or VKORC1 affect warfarin sensitivity. Retrospective studies have shown that patients with at least one variant allele have an increased risk of INRs over the desired range and the variant groups also require more time to achieve stable dosing compared to patient with wild type allele.28,29 Additional prospective observational studies indicate that CYP2C9 and VKORC1 genotype affect warfarin sensitivity.30,31,32 Several prospective studies have incorporated genotyping for CYP2C9 and VKORC1 into algorithms that typically include clinical variables such as age, gender, and drug interactions in an attempt to use genetic information to improve warfarin dosing. The results of these trials are conflicting possibly as a consequence of different trial design and patient populations. Incorporation of CYP2C9 and VKORC1 polymorphisms into a dosing algorithm in one randomized study more accurately predicted warfarin dose and reduced dose modifications, but did not improve time in therapeutic range.33 Another randomized study compared dosing based on genotype and clinical variables to clinical variable alone in almost 1000 patients starting warfarin. This showed no difference in time in therapeutic range, bleeding, or thromboembolism.34 Genotype-based dosing resulted in significantly decreased time in the therapeutic range for black patients in this study suggesting such an approach is detrimental for this subgroup. A third study conducted in Europe reported a significant improvement in time in therapeutic range (67.4 vs. 60.3 percent), episodes of excessive anticoagulation (INR >4), and median time to reach a therapeutic INR (21 vs. 29 days) with genotype-based dosing.35 A similarly designed study by the same European group in patients starting acenocoumarol or phenprocoumon showed no improvement in these same measures with genotype based dosing.36 Further research is needed before genetic testing becomes part of standard care for patients receiving warfarin.
The most serious and common complication of warfarin is bleeding, and its risk is related primarily to patient characteristics, the degree of the anticoagulation, and the duration of therapy. The rate of major bleeding with 3 to 6 months of warfarin treatment in recent clinical trials of patients with venous thromboembolism was 1.2 to 2.2 percent.37,38,39 Several risk models have been developed to predict bleeding risk in patients on warfarin. The HAS-BLED score was derived and validated in a prospective cohort of more than 6000 patients on warfarin for atrial fibrillation (Table 25–3).40 Variables in this model include hypertension, abnormal renal or liver function, stroke, prior bleeding complications, labile INR, age older than 65 years, and drug or alcohol abuse. The intensity of anticoagulation as reflected by the INR is the most important predictor of bleeding risk, which is low in the therapeutic range but increases as the INR prolongs further. The cumulative risk of bleeding increases with a longer duration of treatment, whereas the absolute risk is greatest early, possibly caused by pathologic lesions present at the time therapy is started.
Variable | Points |
Hypertension (uncontrolled, systolic >160 torr) | 1 point |
Abnormal renal or liver function | 1 point each, max 2 points |
Stroke (prior history) | 1 point |
Bleeding history of predisposition | 1 point |
Labile INR (<60% time in therapeutic range) | 1 point |
Elderly (>65 years old) | 1 point |
Drugs/alcohol use (nonsteroidal antiinflammatory drugs, aspirin, antiplatelet agents) | 1 point for each, max 2 points |
A rare complication of warfarin therapy is skin necrosis, which if it occurs usually presents early in the course of anticoagulation.41 Thrombosis in dermal and subdermal venules is the underlying cause, and this may be caused by disproportionately rapid reduction in proteins C and S. Skin necrosis typically begins with burning and tingling at the affected site, which usually involves a region with a large amount of subcutaneous tissue, such as the breast, buttock, or thigh. Painful hemorrhagic full-thickness skin infarction may develop, and frequently will require skin grafting. Other complications from warfarin are rare. Occasional patients report alopecia; hypersensitivity reactions are rare and are almost uniformly caused by the dye used in the pill rather than by the warfarin itself.
Warfarin use in patients with heparin-induced thrombocytopenia (HIT) may be complicated by limb gangrene caused by occlusive venous thrombosis; this effect is likely a result of a combination of inadequate parenteral anticoagulant effect and reduced levels of protein C, in concert with relatively preserved levels of factors II and X that are seen early after the initiation of warfarin administration.42 This complication highlights the need for parenteral anticoagulants to be continued in patients with HIT until the coagulopathy has largely resolved, as indicated by a return of the platelet count to normal or near normal levels.
Oral anticoagulation should be avoided in pregnancy because warfarin crosses the placenta, and exposure during organogenesis in the first trimester can lead to fetal embryopathy with significant cranial bone malformations.43 Anticoagulation during pregnancy increases bleeding complications, especially later in pregnancy. Warfarin may be considered during the second trimester, but heparin or an LMWH is a preferable alternative in most situations. Vitamin K antagonists are safe during lactation.44
Anticoagulation must be reversed for episodes of bleeding, surgery, trauma, or overdosage. Appropriate interventions for patients with excessively prolonged INRs without bleeding include holding warfarin doses, administering low doses of vitamin K (0.5 to 1.0 mg), and increasing the frequency of monitoring (Table 25–4).45 Serious bleeding and major warfarin overdosage requires factor replacement and larger vitamin K doses that may need to be given intravenously. Four-factor concentrates have been developed and tested for reversal of warfarin. A large, open-label, prospective phase IIIB noninferiority study in nonsurgical patients on warfarin requiring reversal for major bleeding compared fresh-frozen plasma (FFP) to four-factor prothrombin complex concentrate (PCC) (factors II, VII, IX, X, protein C, and protein S).46 Four-factor PCC was as effective in achieving hemostasis as plasma (72.4 percent with four-factor PCC vs. 65.4 percent with plasma; absolute difference, 7.1 percent [95 percent confidence interval, −5.8 to 19.9]) and superior to FFP with regards to INR correction within 0.5 hours of infusion (62.2 percent receiving four-factor PCC vs. 9.6 percent receiving FFP; absolute difference, 52.6 percent [95 percent confidence interval, 39.4 to 65.9]). The rate of adverse events, such as death and thromboembolism, was similar between groups.
Indication | Action |
---|---|
INR <6 | Lower the dose, consider withholding 1 or more doses |
Recheck in 3–7 days | |
INR 6–10 | Lower the dose and withhold 1–3 doses |
Consider administering vitamin K, 1–2 mg orally | |
Recheck INR in 24–48 hours | |
INR >10 | Withhold doses until INR in desired range and cause of elevation ascertained |
Give vitamin K, 2–4 mg orally | |
Recheck INR in 24 hours | |
Serious bleeding and major overdose | Administer four-factor prothrombin complex concentrate if available for rapid reversal. If four-factor prothrombin complex concentrate not available administer fresh-frozen plasma. Also give 5–10 mg vitamin K intravenously |
Anticoagulated patients who need invasive procedures represent management problems, and decisions about periprocedural anticoagulation should be based on balancing the risk of thromboembolism with that of bleeding from the procedure. Evidence-based guidelines for bridging therapy are available,45 and the topic has been reviewed.47 The goal is to reduce the intensity of anticoagulation during and immediately after surgery, while avoiding thromboembolism caused by the underlying disease. Generally, the risk of recurrence is greatest in the period shortly after an episode of acute thrombosis and declines progressively over time. If possible, elective surgery and other invasive procedures associated with a high bleeding risk should be postponed during the first several months following acute thrombosis. The bleeding risk is usually highest during surgery and decreases rapidly to baseline after approximately 7 to 10 days. Most surgery can be done with a minimal bleeding risk in patients receiving warfarin and an INR of 1.5 or less. A recent cohort study of 1496 patients on chronic anticoagulation undergoing periprocedural bridging therapy showed that bleeding occurred in 5.1 percent of patients, and risk factors for bleeding included mitral mechanical valve, active cancer, prior bleeding history, and reinitiation of anticoagulation within 24 hours of procedure.48 A randomized study of anticoagulated patients undergoing defibrillator placement showed that bridging therapy was associated with a significant increase in device-pocket hematoma (16 percent vs. 3.5 percent) without a reducing thromboembolic complications.49 Patients at moderate or high risk of thrombotic recurrence should receive heparin or LMWH “bridging therapy” when their INR becomes subtherapeutic. Postprocedural bridging therapy should be undertaken only in patients in whom the risks of this therapy (principally bleeding) are less than the perceived benefits (a reduced risk of thromboembolism).
HEPARIN
Heparin (and the related LMWHs) is the most widely used, rapidly acting, parenteral anticoagulant. Heparin is a heterogeneous mixture of sulfated glycosaminoglycan molecules with varying chain lengths (molecular mass [Mr] 5000 to 30,000 daltons). Heparin has no direct anticoagulant effect, but serves to activate plasma antithrombin (AT), a serine protease inhibitor. Only about one-third of heparin molecules contain the necessary unique pentasaccharide sequence required to interact with AT and thus display anticoagulant activity.50 AT inhibits thrombin, factor Xa, and other coagulation serine proteases in a reaction that is slow by itself, but is accelerated approximately 1000-fold in the presence of heparin.51 To inhibit thrombin, heparin binds to both the active enzyme and AT, forming a ternary complex. The inhibition of factor Xa, however, occurs through binding to heparin–AT complex without the requirement for heparin binding directly also to factor Xa. The requirement for a ternary heparin–AT–thrombin complex requires heparin molecules with 19 or more saccharide units, whereas smaller heparin molecules are effective in promoting factor Xa inactivation. Thrombin and factor Xa are relatively protected from inhibition by the heparin–AT complex when they are surface immobilized within thrombi or on cells.52
Heparin is not absorbed after oral ingestion, so must be given either subcutaneously or intravenously. The pharmacokinetics of unfractionated heparin is complex, a result of extensive protein binding, with a dose-dependent half-life in the range of 1 to 2.5 hours.53 A common protocol uses an initial intravenous bolus of 5000 units or 75 U/kg, followed by a maintenance infusion of 1250 to 1660 U/h or 18 U/kg per hour. The anticoagulant effect is immediate, but laboratory monitoring is needed because of the variability in response among patients. Monitoring is most convenient with the activated partial thromboplastin time (aPTT), which is sensitive to plasma heparin concentrations of 0.1 U/mL or higher. Because different reagents and measuring systems have differing sensitivities to heparin, it is recommended that the therapeutic range be established for each laboratory by calibrating the aPTT to a plasma heparin concentration of 0.2 to 0.4 units by protamine sulfate titration, or 0.3 to 0.7 U/mL using an anti–factor Xa assay.54 The usual aPTT range for heparin therapy is between 1.5 and 2.5 times the mean of the normal range. Clinically useful nomograms are available for adjusting the heparin dose using either fixed- or weight-based dosing.55 Alternatively, monitoring can be performed using anti-Xa levels, which is a useful approach when the aPTT is unreliable, as in patients with baseline prolongation of the aPTT as a consequence of expressing a lupus anticoagulant. Rapid achievement of a therapeutic level, as assessed by the aPTT or anti-Xa level, is important in ensuring an adequate anticoagulant effect.
Some patients fail to display an adequately prolonged aPTT following treatment with unfractionated heparin, despite apparently adequate or even supratherapeutic doses of the drug. This phenomenon is termed heparin resistance and is usually caused by an acute-phase response that results in high levels of procoagulant proteins, including factor VIII. The antithrombotic effect of heparin correlates best with plasma heparin levels, which may be adequate in these circumstances despite a subtherapeutic aPTT.56 For patients who require heparin doses of greater than 35,000 U/day to increase the aPTT into the therapeutic range, consideration should be given to using heparin levels determined by an anti-Xa assay. Substitution of LMWH for unfractionated heparin is another consideration. Although AT deficiency may cause heparin resistance, most AT-deficient patients can be adequately anticoagulated with heparin in usual doses. No monitoring is recommended when low doses of heparin are used for prophylaxis of venous thromboembolic disease, although minimal prolongation of the aPTT may occur. Care is required in very light weight and frail elderly who may be anticoagulated with usual “prophylactic” doses of unfractionated heparin; aPTT monitoring might be considered in such patients.
A large study demonstrated that patients with acute venous thromboembolism can be safely treated with fixed, weight-adjusted heparin doses without aPTT monitoring.57 In this study, 708 patients were allocated randomly to receive either unfractionated heparin with a subcutaneous bolus dose of 333 U/kg followed by a twice-daily dose of 250 U/kg, or LMWH over 3 months of follow up. Recurrent venous thromboembolism occurred in 13 patients who were allocated to unfractionated heparin and in 12 who were allocated to LMWH. Major bleeding occurred in four and five patients, respectively. This study calls into question the need for routine aPTT monitoring of therapeutic dose, weight-adjusted unfractionated heparin and warrants validation.
Heparin has a short half-life, and its anticoagulant effect disappears several hours after discontinuation of an intravenous infusion. Therefore, stopping the infusion and local measures are usually adequate to control bleeding. However, in major or life-threatening bleeding, the anticoagulant effect can be neutralized with protamine sulfate, which is a basic polypeptide that binds tightly to the acidic heparin molecule. The usual dose of protamine required is 1 mg to neutralize 100 units of heparin. The dose to be administered is based on the amount of heparin remaining in the circulation. Protamine is routinely used to neutralize heparin after cardiopulmonary bypass using standard formulas and activated clotting time monitoring.
The most frequent complication of heparin administration is bleeding, which is related to the dose and intensity of treatment, as well as to patient characteristics. HIT is an immune-mediated platelet consumption caused by an antibody directed against a complex of heparin and platelet factor 4 (Chap. 118). Despite thrombocytopenia, HIT is more commonly associated with thrombotic complications than bleeding, and it occurs in approximately 3 percent of patients, depending on the type of heparin, the dose and route of administration, and the indication for heparin anticoagulation (e.g., more common in “surgical patients” than in “medical patients”). The “4T’ score is a clinical prediction tool with good negative predictive value.58 Platelet counts should be monitored during treatment and heparin discontinued if thrombocytopenia occurs, and an alternative anticoagulant that does not interact with the heparin-platelet factor 4 complexes should be administered. Vitamin K antagonists should be given only after the platelet count has risen to greater than 150,000/μL. Long-term heparin therapy can also cause osteoporosis, and radiographic evidence of bone loss occurs in approximately 15 percent of women who receive prolonged treatment during pregnancy, with symptomatic vertebrae fractures in approximately 2 percent. The bone loss may resolve after heparin is discontinued.
Limitations of unfractionated heparin led to studies correlating structural and functional relationships of heparin, and this eventually resulted in the development of LMWHs, several of which are available. LMWH preparations are produced by treating heparin chemically or enzymatically to decrease the size of the polysaccharide chains, yielding products with restricted molecular weight distributions with a mean of approximately 4000 to 5000 daltons.59 Like heparin, LMWHs exert antithrombotic effects through interaction with AT. In the presence of LMWH, AT inactivates factor Xa in the same way as unfractionated heparin, but it is less able to inactivate thrombin because the shorter polysaccharide length does not allow formation of the necessary ternary complex. Consequently, LMWHs have a greater proportion of anti–factor Xa than AT activity.
LMWHs also have different pharmacokinetic properties than unfractionated heparin.54 Following subcutaneous administration, LMWHs are nearly completely absorbed, a clear benefit over unfractionated heparin, which exhibits variable and dose-dependent absorption. LMWHs also exhibit less binding to plasma proteins and cells than unfractionated heparin, resulting in more predictable blood levels and anticoagulant effects. LMWHs have a longer plasma half-life than unfractionated heparins, allowing once- or twice-daily subcutaneous administration.
LMWHs have significant renal clearance, and high levels can accumulate in patients with renal insufficiency. Care must be taken in dosing LMWHs in patients with reduced renal function as bleeding risks are increased,60 and monitoring with anti–factor Xa levels may be needed. Similarly, monitoring may be necessary to achieve appropriate levels in very obese patients, although weight-based dosing probably achieves better anticoagulation. Protamine sulfate does not completely reverse the anticoagulant effect of LMWH but is partially effective and can be useful in patients with serious hemorrhage.61 Several LMWH preparations are available and approved for both prophylaxis and treatment of venous and arterial thrombotic diseases. Each preparation differs slightly and is pharmacologically unique, although the agents are likely similarly effective for the treatment and prevention of venous thrombosis.
Similar to unfractionated heparin, the most common adverse effect is bleeding, which occurs at approximately the same frequency and severity when used in similar patient groups for the same indication. HIT is much less common than with unfractionated heparin,62 occurring only in 0.3 to 0.45 percent of patients. However, cross-reactivity of the antibody occurs, and LMWH is not an acceptable choice for continued anticoagulation in patients with HIT induced by treatment with unfractionated heparin. Animal studies suggest that osteoporosis may be less common with LMWH, and this is reported by several small clinical trials.63
CHOICE OF HEPARIN OR LOW-MOLECULAR-WEIGHT HEPARIN
The factors governing the choice of heparin or LMWH concern effectiveness, safety, convenience, and cost. For treatment of venous thromboembolic disease, the safety and efficacy of heparin and LMWH are comparable. A meta-analysis of 14 studies comparing unfractionated heparin to LMWH in 4754 patients showed that LMWH-treated patients had less recurrent venous thromboembolic complications (4.3 percent vs. 5.6 percent, odds ratio [OR] 0.76, 95 percent confidence interval 0.57 to 1.01) and fewer major bleeding events (1.3 percent vs. 2.1 percent, OR 0.6, 95 percent confidence interval 0.39 to 0.93).64 LMWH also offers better convenience because subcutaneous administration permits outpatient treatment. However, LMWHs may be difficult to use in patients with renal insufficiency because of decreased clearance, and intravenous heparin may offer advantages in such patients. LMWHs are incompletely reversed by protamine sulfate, making them more difficult to use for cardiac bypass surgery. Unfractionated heparin may be preferable in patients who require an invasive procedure on an urgent basis because of its shorter half-life. LMWHs may offer some advantages for patients with acute coronary syndromes.
Danaparoid is a mixture of glycosaminoglycans and is composed of approximately 84 percent heparan sulfate, 12 percent dermatan sulfate, and 4 percent chondroitin sulfate. It is an AT-dependent anticoagulant with predominant anti–factor Xa activity. The plasma half-life is approximately 24 hours with predominant renal clearance. Danaparoid is not reversed by protamine sulfate. Danaparoid differs structurally from heparin and it has been used successfully to treat patients with HIT. Although there is in vitro cross-reactivity of 10 to 20 percent of heparin antibodies with danaparoid, this is of uncertain clinical relevance. Danaparoid is administered subcutaneously, and levels may be monitored with anti–factor Xa assays performed using a danaparoid standard curve. At the time of writing danaparoid has not been approved in the United States, and availability elsewhere was limited.
Fondaparinux is a unique heparin-like anticoagulant with highly selective AT-dependent anti–factor Xa activity.54 It is a completely synthetic pentasaccharide whose structure is based on the heparin sequence that interacts with AT. It binds reversibly and with high affinity to AT, resulting in a conformational change that renders it effective in inhibiting factor Xa, but because of its small size, does not inhibit thrombin. Whereas unfractionated heparin and all LMWHs are derived from animal sources, fondaparinux is synthesized in a structurally homogenous form containing no animal products. Consequently, fondaparinux does not induce allergic responses. Because it inhibits factor Xa but has no direct action on thrombin, its mechanism of action depends on reducing thrombin generation.
Pharmacologic studies show that maximum plasma levels are reached approximately 2 hours after subcutaneous administration with an elimination half-life of approximately 17 hours independent of the dose.65 Bioavailability is nearly complete after subcutaneous or intravenous administration. There is a low intra- and intersubject variability with little accumulation after multiple daily doses. Because elimination is primarily renal and the agent is excreted unchanged in the urine, fondaparinux is contraindicated in patients with severe renal impairment. Fondaparinux plasma levels can be measured with the anti–factor Xa assay, but there is no effect on other coagulation assays including the activated clotting time (ACT), aPTT, or thrombin clotting time.
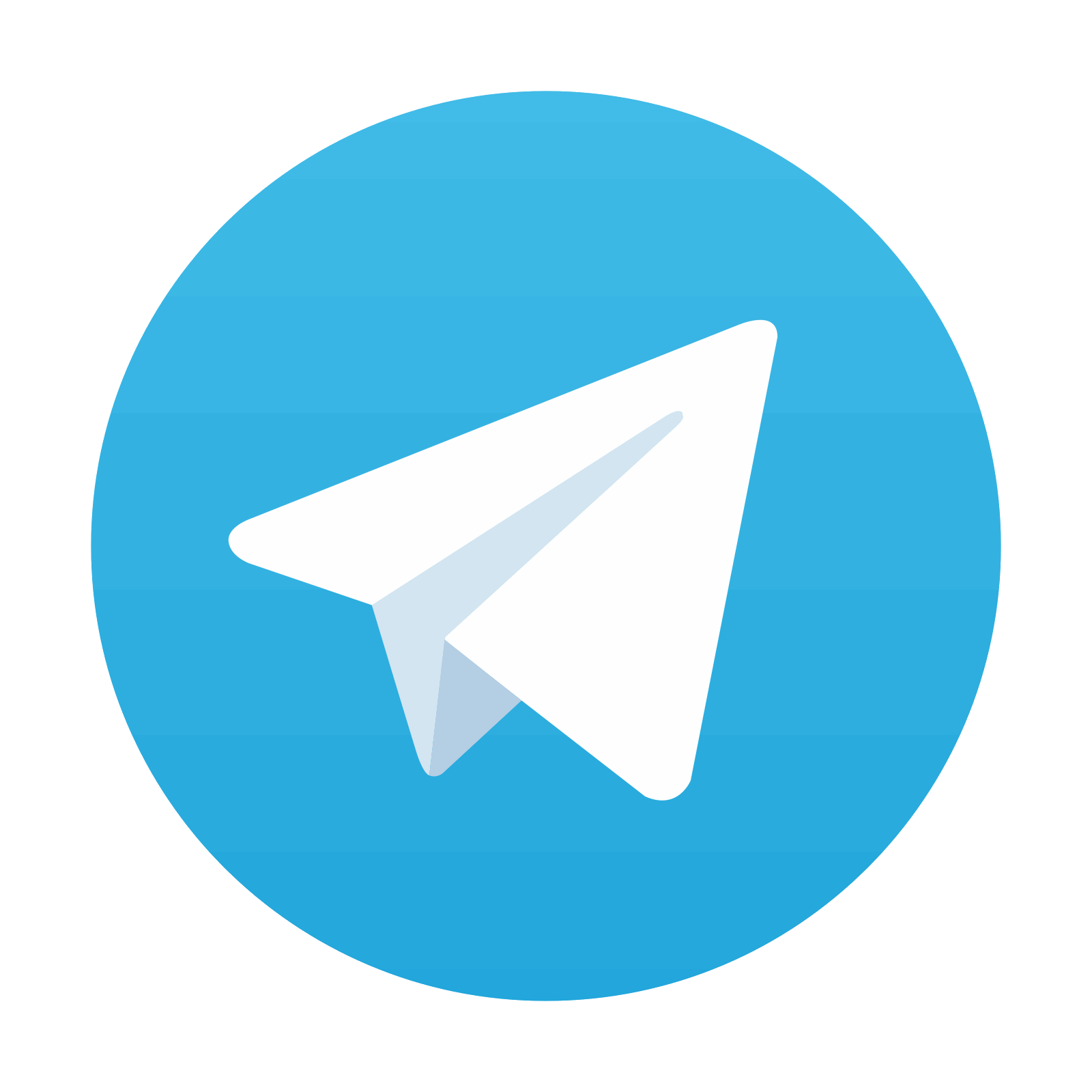
Stay updated, free articles. Join our Telegram channel

Full access? Get Clinical Tree
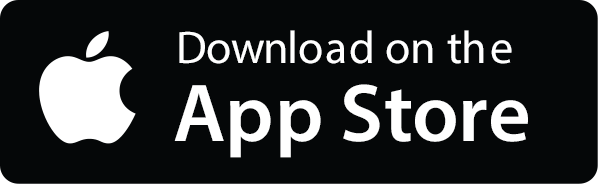
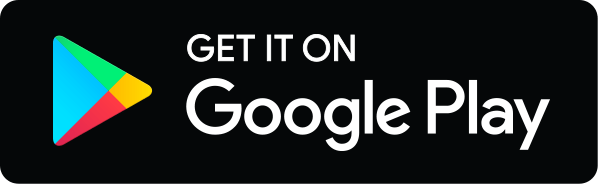