Key points
- •
Thermal ablation is a proven method for treatment of early-stage non–small cell lung carcinoma for patients who are medically inoperable.
- •
Basic approaches to lung biopsy and ablation are similar.
- •
The risks of lung intervention include pneumothorax, hemorrhage, and air embolism.
Introduction
Lung cancer is the leading cause of cancer-related death in the United States for both men and women. Using data acquired through the North American Association of Central Cancer Registries (NAACCR), in 2012, an estimated 226,160 Americans will be diagnosed with lung cancer, and 160,340 people will die of the disease. The 5-year survival rate of all lung cancers is 15.7%, based on data acquired from 1995 to 2001. Primary lung cancer is classified as either non–small cell lung cancer (NSCLC) or small cell lung cancer (SCLC). Non–small cell lung cancer accounts for 87% of cases.
Smoking is implicated in up to 90% cases of primary lung cancer. Other causes include environmental exposures (i.e., air pollution), occupational exposures (i.e., asbestos), pulmonary fibrosis, and exposure to ionizing radiation (i.e., radon). It is generally believed that genetic factors play a role in determining a patient’s risk for ultimately developing cancer. This is supported by evidence that cancer may cluster in individual families. , Lung cancer is up to four times more common in Asian Americans in comparison to White Americans. Epidermal growth factor (EGFR) mutations are common in the lung cancers of East Asians, particularly in those without a smoking history.
Percutaneous thermal ablation of primary lung neoplasms, in the form of radiofrequency ablation (RFA), microwave ablation (MWA), and percutaneous cryoablation therapy (PCT), has emerged as viable alternative to surgical resection for patients with early stage disease who have medical comorbidities that put them at an increased operative risk. Interventional radiologists play a vital role in the multidisciplinary team through detection, tissue diagnosis, treatment, and posttherapeutic follow-up. This chapter will provide a basic overview of lung cancer staging, treatment options, thoracic interventional techniques, and imaging follow-up.
Staging
Lung cancers are divided into non–small cell and small cell cancer based on histology and immunohistochemistry. Both cancers are staged according to the 7th edition of the International Association for the Study of Lung Cancer (IASLC). , There are considerable differences in the treatment of these two subtypes of bronchogenic carcinoma. Treatment within each category is largely based on stage of disease. Table 24-1 provides a schematic for lung cancer staging. This chapter will primarily focus on treatment options for NSCLC.
Stage I and II tumors are considered early stage, and potentially curably through surgical resection. The 5-year survival rates for stage I and II NSCLC are 60%–80% and 40%–50%, respectively. In stage III disease, 5-year survival is 14% (IIIa) and 5% (IIIb). Stage IV lung cancer has a dismal prognosis, with 5-year survival of approximately 1%.
Treatment options
All interventional radiologists must have a working knowledge of the treatment options available to patients with NSCLC in order to be a productive member of the multidisciplinary lung cancer team. The traditional treatment for early-stage lung cancer has been resection, whereas chemotherapy and radiation have been primarily used for advanced-stage disease. Thermal ablation has proven useful in the treatment of patients with early-stage lung cancer, with a medical contraindication to resection, or in a growing population of patients who refuse resection. It is common for patients referred for thermal ablation to have previously undergone one or more of these treatments. In patients who cannot tolerate resection, ablation allows a less invasive tumor treatment, with objectives including size reduction and potential cure.
Surgery
Surgical resection is potentially curative and is the primary therapy for patients with stage I, II, and some IIIa NSCLC, who can tolerate a pneumonectomy or lobectomy. The standard practice for treatment of early-stage lung cancer has been lobectomy and lymph node dissection. This treatment has been shown to provide long-term disease-free survival.
Debate exists as to the extent of resection that is necessary for the treatment of stage Ia disease, with some now favoring a sublobar resection to preserve lung capacity. Multiple single-center studies have suggested no survival benefit for lobectomy in comparison to segmentectomy or wedge resection. , These findings are in contradistinction to the results of the Lung Cancer Study Group, which demonstrated a threefold increased incidence of local disease recurrence and 50% increased mortality for patients treated with sublobar resection in comparison to patients treated with lobectomy for stage Ia disease. Brachytherapy has been shown to decrease the rate of locally recurrent disease after sublobar resection.
Unfortunately, only one-third of patients with stage I or II NSCLC tumors are eligible for resection on the basis of pulmonary function tests. In patients who cannot tolerate resection thermal ablation, it has a role to achieve localized control and cure of disease.
Stage IIIa disease is treated with a combination of chemotherapy, radiotherapy, and surgery, whereas stage IIIb and stage IV cancers are treated with chemotherapy or radiotherapy without resection.
Radiation
Although conventional radiotherapy has traditionally been associated with poor local control of disease and a high rate of radiation-associated toxicity, , the advent of stereotactic body radiation therapy (SBRT) has increased the role of radiation oncology in the treatment of NSCLC. Stage I and II lung cancers are now viewed by many in the radiation oncology community as curable disease. Timmerman et al. studied patients with medically inoperable stage I and II lung cancers treated with SBRT and demonstrated 87% locoregional control of disease and disease-free survival of 48.3% at 3 years. Adjuvant radiation, in contradistinction, is not indicated for surgically resected stage I or II lung cancers.
SBRT and conventional radiotherapy are routinely used to treat stage III and IV lung carcinomas, both to achieve local control and to prevent complications of the cancer, such as superior vena cava syndrome. The delivery of SBRT is effective and well tolerated by patients. Some limitations do exist, particularly when treating central tumors. Radiation toxicity, including pleural effusion, pulmonary hemorrhage, radiation pneumonitis, and pulmonary fibrosis, increases with escalating dose and, in many cases, limits the extent of treatment.
A role for combined external beam radiation or brachytherapy with thermal ablation may exist. These studies have used postablation radiotherapy to enhance the margin of the ablation and have shown better local control of disease when combined-modality treatment is utilized. It is also possible that a radiation-first approach to combined therapy may result in a larger subsequent ablation size through radiation-induced damage to vasculature in and around the target mass, thereby relieving a degree of heat sink (the reduction in effective ablation that results from local vasculature removing heat). This approach is the subject of active study.
Chemotherapy
The past several years have yielded important advances in the chemotherapeutic management of NSCLC. The discovery that lung cancer patients with mutations in the EGFR gene have a treatment response to certain tyrosine kinase inhibitors has signaled the dawn of targeted chemotherapy for lung cancer. The role for chemotherapy in early-stage lung cancers is a topic of debate, whereas it remains the primary treatment for patients with advanced disease.
Mutations in the tyrosine kinase–binding domain of the EGFR gene have been found in a disproportionate number of nonsmoking, east-Asian women presenting with primary lung adenocarcinoma. These changes include base-pair deletions at exon 19 and mutations at exon 21. Patients with these mutations have responded well to tyrosine kinase inhibitors, including gefitinib and erlotinib. , , The Spanish Lung Cancer Study Group selected patients diagnosed with adenocarcinoma EGFR mut and treated them with erlotinib as a first- or second-line agent. These patients had a mean survival of 24 months, in comparison to a historical mean survival of 8 months.
Adjuvant chemotherapy is not indicated following resection of stage I NSCLC. It is recommended following surgery for stage II disease, provided the patient has a good performance status. Chemotherapy is always considered for stage III and IV disease, with or without concurrent radiotherapy. Platinum-based agents have traditionally been the mainstay of treatment.
Image-guided interventions
Interventional radiologists have an established role in providing tissue diagnosis of lung carcinoma via percutaneous biopsy, as well as treatment of localized disease with thermal ablation. This section will provide an overview of percutaneous tissue biopsy and technical approaches to lung interventions.
Preprocedure assessment
A standard preprocedure assessment of the patient is performed, including history, physical examination, and coagulation status. A high percentage of patients undergoing lung intervention are smokers and, therefore, have comorbidities of cardiac and pulmonary disease, factors that may limit tolerance of sedation and some positions on the procedure table. All attempts should be made to optimize these factors prior to elective intervention.
Goals for factors effecting hemostasis include platelets >70,000, INR <1.5, and a partial thromboplastin time (PTT) of <1.5% control value. Patients on warfarin sodium (Coumadin) who are at high risk of complications of thrombosis can be bridged with subcutaneous heparin or enoxaparin. Aspirin and clopidogrel bisulfate (Plavix) should be discontinued for 7 days prior to the procedure.
Standard labs are drawn in the preprocedure area, including complete blood count (CBC), chemistry, and coagulation factors. Some interventionalists prefer the use of a blood patch following biopsy, and 10 mL of blood is obtained in a syringe for use at the completion of the procedure to reduce the incidence of pneumothorax.
Biopsy
The first step toward treating thoracic malignancy is to establish a pathologic diagnosis through tissue biopsy. Technical aspects of placing probes for percutaneous thermal ablation mirror that for biopsy, and therefore, it is advisable to establish good biopsy technique prior to embarking upon thermal ablation.
Despite the wide availability of fluoroscopy and CT for obtaining an image-guided biopsy, lung tissue continues to be obtained largely through surgical techniques. Based on data extrapolated from the National Lung Screening Trial (NLST), up to 50% of initial diagnostic biopsies remain surgical, despite its invasive nature and added risk of general anesthesia.
Image guidance provides a less-invasive method for obtaining tissue and can typically be performed with conscious sedation. Traditionally, fluoroscopy has been used to guide a fine-needle aspiration of the target lesion. With the wide availability of CT, the majority of image-guided lung biopsies are now obtained under CT guidance. CT provides superior resolution of both the target lesion and intervening tissue, which maximizes appropriate sampling and minimizes risks, such as traversing major blood vessels and airways.
Core-needle biopsy has emerged as the preferred method of obtaining lung tissue. Yao et al. established that the safety profile of lung core needle biopsy is on par with fine-needle aspiration. Despite larger needle size, there is no increased risk of adverse events, specifically with regard to pneumothorax and hemorrhage. Most importantly, core needle biopsy allows the pathologist to look at a histologically intact piece of tissue, which improves diagnostic capability and decreases the chance of both a false-negative biopsy and a repeat biopsy.
Core-needle biopsy technique
At the authors institution, after detailed informed consent is obtained, the patient is brought to the CT procedure suite and placed on the table in the anticipated position for the procedure. The patient is attached to a vital signs monitor, placed on oxygen via nasal cannula, and administered conscious sedation. A combination of low-dose narcotic (opiate) and hypnotic (benzodiazepine) is preferred. Morphine sulfate has the added benefit of suppressing the cough reflex, which is particularly helpful during lung interventions.
A focused initial CT scan is performed to identify a site of percutaneous access. The site of access is then marked with a radiopaque marker and a repeat scan is performed to ensure that the marked site is appropriate. The skin is prepped, and a sterile field is created with surgical towels.
The importance of appropriate local anesthesia cannot be emphasized enough. Although conscious sedation is typically employed, lung biopsy and ablation can on occasion be performed with only local anesthesia if the patient has a significant sedation risk. As is typical of most access sites, there are two locations with pain nerve fibers that deserve special attention—in this case, the skin and parietal pleura. These sites require adequate anesthesia prior to advancing a needle into the lung parenchyma to ensure patient comfort, cooperation, and absence of movement during the course of the procedure.
Lidocaine 1% or bupivacaine 0.25% is administered to the skin and subcutaneous soft tissues with a 25-guage hypodermic needle. Care is taken not to advance the needle into the lung tissue. A small skin nick is made with a scalpel. Under CT guidance, typically an 18 or 19-guage coaxial needle is then advanced into the extrapleural space, inside the internal thoracic fascia and at the edge of the parietal pleura. The patient will frequently complain of pain when this space is entered, because of irritation of the pleura. Following confirmation of position with CT, approximately 10 mL of local anesthetic is infused into the space, which should demonstrate a visible convex wheel with mass effect on the pleura during subsequent imaging ( Figure 24-1 , A ). If the patient coughs or complains of a sour taste in the mouth during the administration of pleural anesthesia, this indicates infiltration of the lung parenchyma.
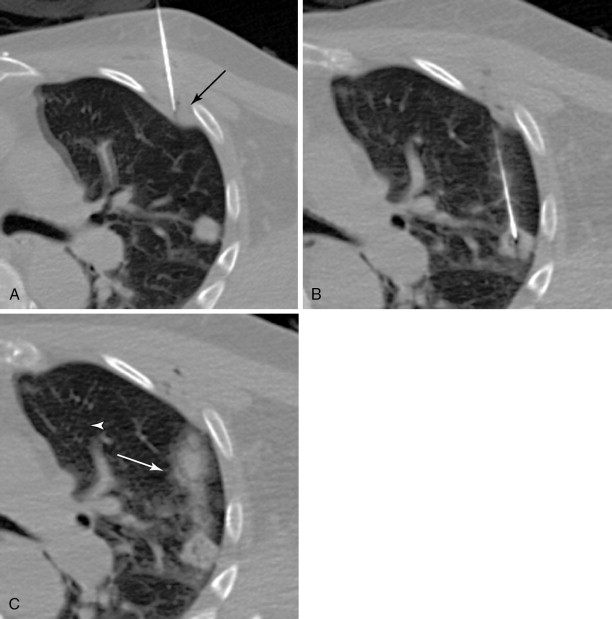
Preparation for entering the lung is completed by a repeat CT, which is used to ensure that the trajectory of the needle is on target. Once the needle is placed into the lung, very little directional correction is possible, as the density of the chest wall soft tissues maintains the needle in its extrapulmonary trajectory.
The coaxial needle is then advanced into the lung. A brisk jab should be used to traverse the pleura to ensure that the lung is appropriately entered; slower pushing movement will tend to displace the lung away and possibly result in a pneumothorax. Roughly 2 cm of lung tissue must be entered to ensure that the needle does not retract intermittently into the pleural space.
The needle is then guided into the target lesion using intermittent CT. Care is taken to avoid traversing major vascular structures and airways. Fine adjustments in needle trajectory are often required to achieve this goal. The needle is advanced to the near-edge of the target. When possible, it is advisable to imbed the needle into the target to ensure appropriate sampling ( Figure 24-1 , B ). This may not be possible with small pulmonary nodules less than 6 mm in diameter.
Core biopsy samples are then obtained using a 20-gauge cutting needle placed through the outer cannula of the coaxial needle. Several samples should be obtained in order to obtain adequate tissue for a diagnosis, and therefore minimize the risk of a false-negative biopsy. In our institution, a touch imprint of the first core biopsy sample is evaluated by a cytotechnologist, who is able to confirm cellularity of the specimen. The remaining cores are then placed directly into formalin. If a lymphoproliferative disorder, most commonly lymphoma, is suspected, the cores are placed into a medium from which flow cytometry can be performed (ex RPMI [Roswell Park Memorial Institute] medium). When lymphoma is suspected, larger cores may be required. Lymphoproliferative masses requiring traversal of lung tissue for biopsy are sampled with a smaller system, as the use of larger coaxial needles has been shown to lead to a higher rate of pneumothorax. Cores should be sent for culture if infection is a possibility.
As the needle is removed, a blood patch may be placed by injecting a suspension of reconstituted blood at a rate of 1 mL/cm to the level of the pleura. This blood should be obtained at the time of laboratory blood draw. The goal of the blood patch is to prevent pneumothorax by occupying airspaces along the needle tract ( Figure 24-1 , C ). Since initial description by McCartney, several studies have evaluated the efficacy of blood patch. The results have been mixed. Lang showed decreased occurrence of pneumothorax when a blood patch was applied. Other studies have failed to find a benefit. ,
A postprocedure CT is performed to ensure no immediate event occurs requiring intervention. The field of view is increased to maximize the evaluation, and should include the heart. Specific attention should be made to evaluate for pneumothorax, hemorrhage, and air embolism. More regarding the management of these adverse events will be discussed in a subsequent section.
The patient is then placed in the decubitus with the biopsied lung in a dependent position to minimize lung excursion, a further effort to prevent development of a pneumothorax. Oxygen is continued via nasal cannula. The patient is transferred to the postprocedure unit and observed for 3 hours. Two portable upright radiographs are obtained with an identical technique, at 1 and 3 hours, to evaluate for delayed or increasing pneumothorax. In the absence of significant or enlarging pneumothorax, the patient may be discharged with instructions to return to the emergency department for increasing shortness of breath, chest pain, or hemoptysis. We prescribe codeine elixir to limit cough in the days following the procedure.
Targeting and trajectory
There are several considerations when choosing a trajectory of a percutaneous chest intervention, including the motion of the chest wall and lung during respiration, as well as the orientation of pleural interfaces, blood vessels, and airways. Preprocedure planning to account for each of these variables is essential for a successful and safe intervention.
Chest wall motion
Although the shortest skin to target approach is often appealing on a static image, it can prove quite frustrating in practice. Significant motion of the chest wall occurs with normal respiration, which can result in considerable movement of the needle or probe, which both decreases targeting, and increases the chance of a complication.
The chest wall and ribs move in an up-and-out bucket handle direction during inspiration, with most motion occurring laterally ( Figure 24-2 ). A lateral intercostal approach should be avoided when possible to avoid considerable needle motion and redirection by moving ribs. The preferred access into the thorax is from close to either the anterior or posterior midline, as there is relatively little motion of the intercostal space at the costovertebral and costochondral junctions (see Figure 24-1 , A ).
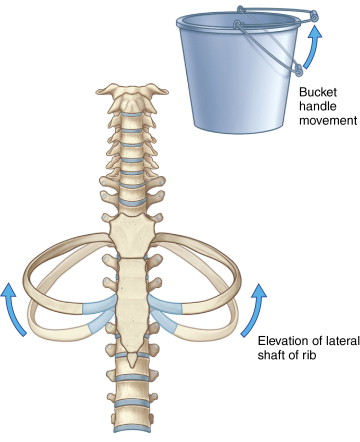
Peripheral lesions and the tangential approach
Similarly, the lungs move considerably during the respiratory cycle, which is particularly problematic when targeting peripheral lesions. If such a lesion is targeted in a direct manner perpendicular to the chest wall, the tip of the needle has the tendency to retract to the level of the pleura during inspiration, particularly if the needle is being held firmly by the chest wall soft tissues. As a result, targeting is made more difficult, and there is increased risk of multiple pleural punctures ( Figure 24-3 ).
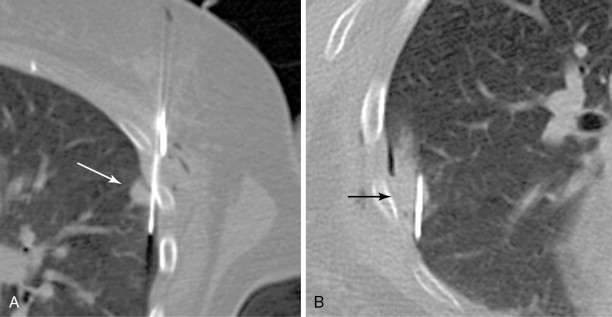
A tangential approach to peripheral lung lesions is quite useful. The tangential approach utilizes a shallow, elongated, intrapulmonary course in parallel to the pleura, directed toward the lesion (see Figure 24-1 , B ). Targeting is aided as the needle and lesion move in tandem with each other. The lung tissue itself acts as a stabilizer of the needle, and there is little chance of the needle backing itself out of the lung. Finally, the tangential approach is essential in many thermal ablations of peripheral lesions in order to limit backward thermal injury of the pleura and chest wall.
Central lesions and the axial approach
The bronchovascular architecture of the lung originates in the hilum and fans out toward the periphery. Centrally located lesions are therefore in closer proximity to a number of blood vessels and airways, adding to the technical difficulty of intervention. These lesions can be targeted successfully, and safely, by using an axial approach. The needle or probe is placed in parallel to the bronchovascular structures, which minimizes the need to traverse large vessels and airways. The final position of the needle at the time of biopsy or ablation will likewise be directed in parallel to these structures, and thereby limit the chance of inadvertent laceration ( Figure 24-4 , B – D ).
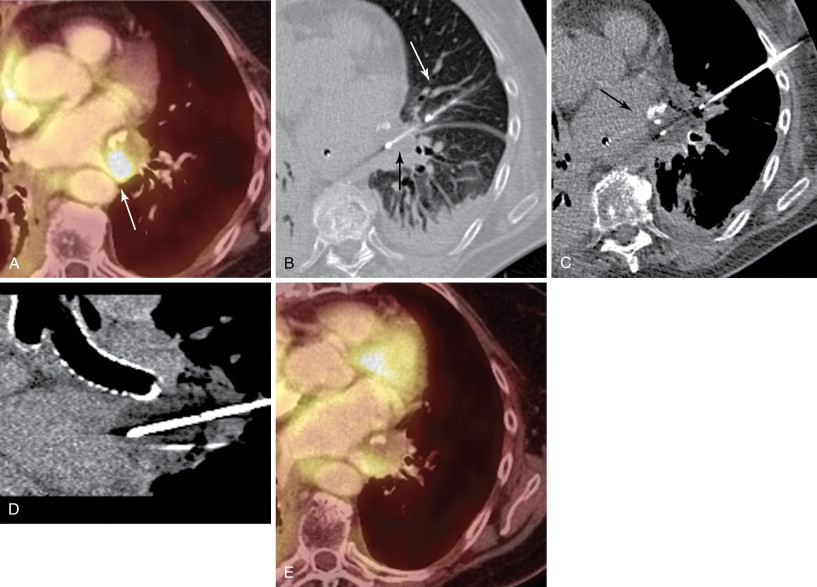
Similarly, it is advisable to use a parallel approach when a target lesion is located in proximity to critical, pulsating central structures, including the aorta, pulmonary arteries and veins, and heart and pericardium ( Figure 24-5 ). These structures have significant excursion during systole, which is not captured on static CT images. Puncture injuries can occur when the needle is directed perpendicular to these structures, which may result in severe blood loss, cardiac tamponade, and death.
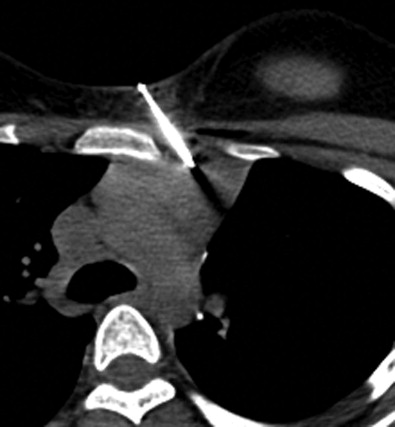
Pleural interfaces
Violation of the visceral pleura when accessing the lung provides a potential communication between the alveolar sacs and the pleural space, and as such, is the primary risk factor for developing a pneumothorax. The visceral pleura should be crossed as little as possible during a procedure, preferably no more than once with a given needle, as each crossing would be expected to add proportionate risk of a subsequent air leak.
Pulmonary fissures are formed from a double layer of in-folded visceral pleura, with the intervening space representing a continuity of the pleural space. The fissures should not be traversed during intervention, unless absolutely necessary, as crossing a single fissure results in two holes in the visceral pleura.
Enlarged airspaces
Many patients presenting for lung intervention will have enlarged airspaces. Causes include bullae and architectural destruction in emphysema, and airspace dilation in airways disease or in the presence of prior lung resection. The decreased density of pulmonary parenchyma results in increased risk of both pneumothorax and significant hemorrhage due to the relative lack of ability to tamponade the hole. Attempts should be made to traverse regions of normal lung architecture to minimize risk ( Figure 24-6 ).
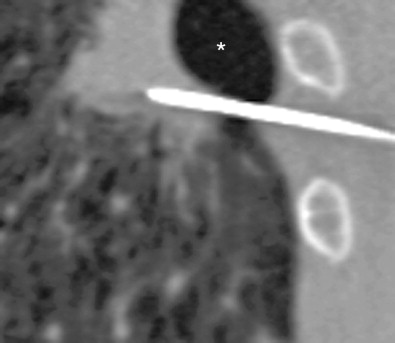
Thermal ablation
Overview
The delivery of cytotoxic thermal energy in the form of heat (RFA and MWA) and cold (PCT) has been well established as an effective means for treating localized primary and secondary tumors of the thorax. Thermal ablation has likewise been used to treat residual or recurrent disease at the margins of surgical resections and radiation fields. All three modalities are well tolerated and safe, with complications not significantly different from percutaneous core-needle biopsy.
All modalities aim to deliver thermal energy to a tumor in order to create a cytotoxic ablation zone that encompasses the entirety of the visualized tumor along with a disease-free margin, theoretically equivalent to that of surgical wedge resection. In general, there is an overlap in selection of one ablative modality over another when treating most thoracic neoplasms. A learning curve exists for each device—comfort with the device is of the upmost importance in achieving an effective ablation.
Although no direct comparison studies of the ablative modalities have been performed, important differences do exist between RFA, MWA, and PCT that can lead to a more effective, better tolerated, and safer ablation on a case-by-case basis. The following sections will provide a general discussion of patient selection for thermal ablation and the thoracic application of each modality.
Patient selection
In general, ablation of a primary lung neoplasm is only appropriate for early-stage, localized disease. It is essential that all patients referred for thermal ablation be well integrated into a multidisciplinary lung cancer team that includes thoracic surgeons, medical oncologists, radiation oncologists, and intervention radiologists. A determination as to whether the patient has surgically resectable disease needs to be made. The patient also requires evaluation by the medical oncologist to ensure appropriate postintervention care and possibly adjuvant chemotherapy.
Most patients who are referred for ablation will have stage I or II disease, and medical comorbidities that preclude surgery. Another subset of patients includes those who have previously had surgical resection or radiation therapy, but have local disease progression. Patients with synchronous or metachronous primary lung cancers may be referred for combined treatment with other modalities, in order to preserve lung volume. This is particularly true for the significant number of patients with preexisting lung compromise secondary to emphysema. Finally, some patients prefer a nonsurgical management of the tumor, in particular for radiologically detected adenocarcinoma in situ or minimally invasive adenocarcinoma.
A decision regarding preferred ablation modality is usually made based on the size, location, and proximity of the tumor to vital structures ( Table 24-2 ). Complete ablations can be compromised by the heat sink effect when the lesion borders blood vessels measuring greater than 3 mm.
Parameters | Radiofrequency | Microwave | Cryoablation |
---|---|---|---|
Size <3 cm | ± | ± | ± |
Size >3 cm | ± | + | ± |
Thermal sink | − | + | ± |
Peripheral | − | ± | + |
Border mediastinum | ± | − | + |
Border large airways | − | − | + |
Hemorrhage | ± | ± | − |
Ablation zone visibility | ± | ± | + |
Pacemaker | − | ± | + |
Time | ± | + | − |
Pneumothorax | ± | − | + |
Pleural effusion | ± | ± | − |
The relative contraindications to lung ablation are similar to those of surgical resection. Patients with decreased pulmonary functional reserve, including those with severe emphysema, airways disease, prior lung resection, and pulmonary arterial hypertension, are at increased risk. Likewise, patients with coronary artery disease, valvular heart disease, and arrhythmia are at increased risk for anesthesia. Although ablation is typically performed with conscious sedation, assistance of an anesthesiologist may be indicated in these cases.
Thermal ablation has been shown to be safe in patients with a cardiac pacemaker or AICD ; however, RFA can disrupt the function of the device. MWA likely has less effect on pacing devices than RFA. It is our practice to obtain a cardiac consultation prior to and following heat-based ablation to ensure appropriate device function. PCT is safe to use with cardiac devices, and obviates cardiology consultation, although patients with cardiac disease taking blood thinners, including warfarin, require bridging with short-acting anticoagulants, such as enoxaparin, prior to the procedure.
Postablation care
Following ablation, patients are instructed to not exert themselves for several days. It is common to have a small amount of blood-tinged sputum, which should resolve after a day or two. Mild chest discomfort is expected, but severe chest pain or shortness of breath are not and may indicate a pneumothorax or increasing pleural effusion.
It is common to experience a flulike “postablation” syndrome following RFA and MWA, which is characterized by low-grade fever, malaise and myalgias, and may last up to 2 weeks. PCT is not associated with development of postablation syndrome.
Clinical follow-up is essential. We attempt to see the patient within 1 week if he or she is not already consulting another clinician. Initial imaging follow-up is in 1 month, with subsequent imaging at 3, 6, 9, 12, 18, and 24 months. Yearly imaging is subsequently obtained for stable disease.
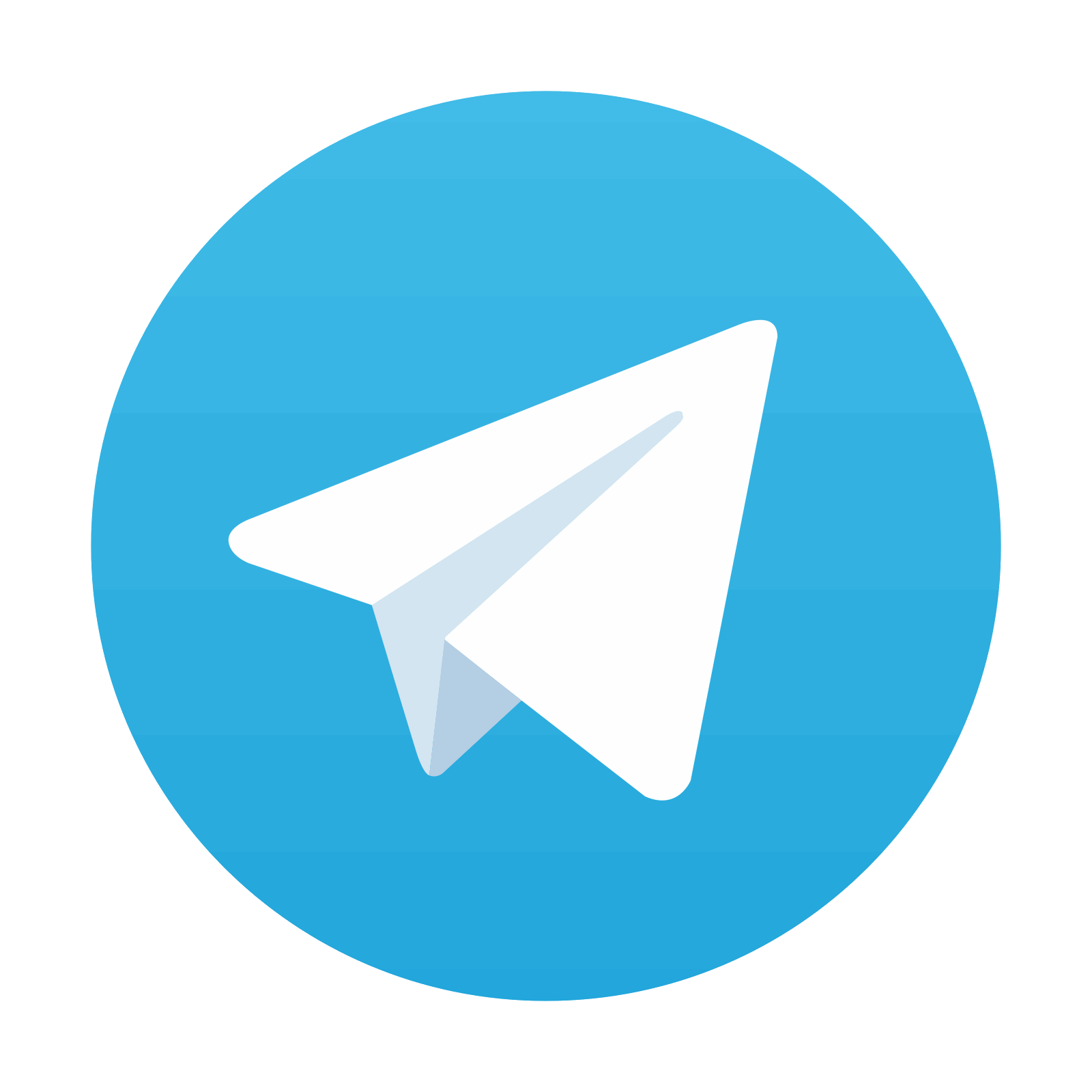
Stay updated, free articles. Join our Telegram channel

Full access? Get Clinical Tree
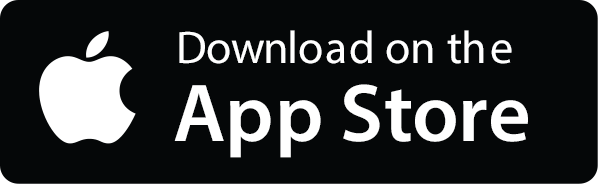
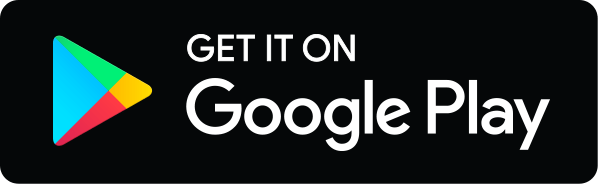