Gene
Disease
Inheritance
Clinical features
Pathogenesis
Immune defects
Associated cancers
ATM (OMIM*607585)
Ataxia-telangiectasia
AR
Cerebellar ataxia, oculocutaneous telangiectasia, chromosomal instability, radiosensitivity, thymic aplasia, recurrent sinopulmonary infections, cancer predisposition (up 40 %)
Disorder of cell cycle checkpoint and DSB repair, role in V(D)J, CSR
Often decreased IgA, IgE, and IgG subclasses, increased IgM, antibodies variably decreased, progressive T-cell lymphopenia, normal B-cell count
NBN (OMIM*602667)
Nijmegen breakage syndrome
AR
Severe microcephaly, bird-like face, mental and growth retardation, chromosomal instability, radiosensitivity, recurrent sinopulmonary infections, strong predisposition to lymphoid malignancy
Disorder of cell cycle checkpoint and DSB repair, role in V(D)J, CSR, SHM
Often decreased IgA, IgE, and IgG subclasses, increased IgM, antibodies variably decreased, decreased B- and T-cell counts
Hodgkin and non-Hodgkin lymphomas, leukemias (mainly B cells), brain tumors [314]
BLM (OMIM*210900)
Bloom syndrome
AR
Short stature, bird-like face, sun-sensitive erythema, erythema, marrow failure, chromosomal instability, cancer predisposition
Role as a RecQ-like helicase
Low IgM and IgA, normal B- and T-cell counts
Leukemias, lymphomas, carcinomas [315]
LIG4 (OMIM*601837)
DNA ligase IV deficiency
AR
Microcephaly, facial dysmorphisms, radiation sensitivity, may present with RS-SCID, Omenn syndrome, or with a delayed clinical onset
Impaired NHEJ, role in V(D)J, CSR
Decreased serum Igs, decreased B- and T-cell counts
DCLRE1C (OMIM*602450)
Artemis deficiency
AR
Radiation sensitivity, may present with RS-SCID or Omenn syndrome
Role in V(D)J, CSR
Decreased serum Igs, markedly decreased B- and T-cell counts
EBV-positive B-cell lymphomas [318]
RMRP (OMIM*250250)
Cartilage hair hypoplasia
AR
Short-limbed dwarfism with metaphyseal dysostosis, sparse hair, bone marrow failure, autoimmunity, predisposition to cancers, impaired spermatogenesis, neuronal dysplasia of the intestine
Role in ribosomal RNA processing, mitochondrial DNA replication, and cell cycle control
Normal or reduced serum Igs, variably decreased antibodies, normal B-cell count, decreased or normal T-cell count, impaired lymphocyte proliferation
Non-Hodgkin lymphomas, basal-cell carcinoma [319]
PMS2 (OMIM*600259)
PMS2 deficiency (class switch recombination deficiency due to impaired mismatch repair)
AR
Recurrent infections, café au lait spots, cancer predisposition
Defective CSR-induced DSBs in Ig switch regions
Low IgG and IgA, elevated IgM, abnormal antibody responses, normal B-cell count, decreased, switched, and non-switched B-cell counts
Leukemias, lymphomas, colorectal carcinoma, brain tumors [320]
18.8.2 Signal Transducer and Activator of Transcription 3 Deficiency
Hyper-IgE syndrome (HIES) is a complex PID characterized by recurrent staphylococcal infections beginning early in infancy, predominantly involving the skin and lungs, chronic eczema, and markedly high serum IgE concentrations [325–327]. Skin infections due to S. aureus lack the usual local or systemic features of inflammation, forming so-called cold abscesses [328]. Recurrent sinopulmonary infections, resulting in bronchiectasis and pneumatocele formation frequently superimposed with bacterial and fungal infections, are the major causes of morbidity and mortality in patients with HIES [329]. Despite having extremely high serum IgE levels and eosinophilia, patients with HIES are usually free from other allergic manifestations, recognized as a marked difference from DOCK8 deficiency [325, 327]. In patients with HIES, serum IgG, IgM, and IgA levels are usually normal; however, most have impaired antigen-specific Ab response to immunization [330]. Diminished circulating memory B cells and defects in the differentiation of Th17 cells have also been demonstrated [330–332]. The multisystem nature of the disease extends beyond the immune system and accounts for the characteristics craniofacial, musculoskeletal, dental, and vascular abnormalities [333–336].
Dominant negative mutations in STAT3 (OMIM*102582) have been identified as the major molecular etiology of autosomal dominant and sporadic cases of HIES [337, 338]. STAT3, one of the seven STAT proteins in the human, is a transcription factor and plays a critical role in responses to many cytokines and growth factors through the shared signal-transducing molecule gp130 [326, 327]. It is crucial for cell proliferation, survival, migration, apoptosis, and inflammation in various tissues [339], probably explaining the diverse clinical findings in patients with HIES.
STAT3 deficiency is associated with an increased risk of LPDs, most notably HL and NHL (relative risk: 259), with the majority of B-cell origin and aggressive histology [340–342]. Other cancers described in patients with HIES include leukemia and cancers of the vulva, liver, and lung [343]. The underlying mechanisms, however, remain unclear. The higher risk of tumor formation has been attributed to defective immunosurveillance and chronic B-cell stimulation, resulting in an increased turnover of B cells and accumulating genetic aberrations, giving rise to malignant B-cell clones [99].
18.8.3 Wiskott–Aldrich Syndrome
Wiskott–Aldrich syndrome (WAS) is a rare X-linked immunodeficiency with highly variable manifestations characterized by thrombocytopenia with small platelets, eczema, and humoral and cellular immunodeficiency with increased susceptibility to pyogenic and opportunistic infections. Patients with WAS may also manifest with an increased incidence of autoimmunity and malignancies [344–349].
The disease is caused by mutations in the WAS gene (OMIM*300392), which is expressed exclusively in hematopoietic cells. Around 300 unique mutations spanning the WAS gene have been described. The effect of a given mutation on WASp expression correlates with the disease severity: mutations that cause decreased WASp levels result in the mild variant X-linked thrombocytopenia (XLT), characterized mainly by thrombocytopenia [350, 351], whereas mutations that abolish WASp expression or result in the expression of a truncated protein are associated with classic WAS. In addition, a third disorder termed X-linked neutropenia (XLN), characterized by neutropenia and variable myelodysplasia, has been attributed to activating mutations in the GTPase-binding domain of WASp [178, 352, 353].
The WAS protein (WASp) is a multifaceted protein which exists in complex with several partners involved in relaying signals from cell surface receptors to the actin cytoskeleton; lack of WASp results in cytoskeletal defects that compromise multiple aspects of normal cellular activity including proliferation, phagocytosis, immune synapse formation, adhesion, and directed migration [347]. It is therefore not surprising that lack of WASp results in a wide range of defects in cellular function involving all hematopoietic cell lineages [347].
Malignancies are relatively common in older patients (adolescent and young adults), especially in those with autoimmune manifestations, and are frequently associated with a poor prognosis [345, 348, 354]. The most frequent malignancy reported is B-cell lymphoma, which often occurs in EBV-positive patients [345, 349]. In a report of 154 patients with WAS, 21 (13 %) developed malignancies, mostly of lymphoreticular origin, with the average age at onset of 9.5 years [345]. Nonlymphoid malignancies, including glioma, acoustic neuroma, testicular carcinoma, and Kaposi sarcoma, have infrequently been reported [345, 355]. The development of hematological malignancies in WAS patients is at least partly due to NK cell and cytotoxic T-lymphocyte dysfunction [356–358], absent of invariant NKT cells [359, 360], and chronic stimulation of autoreactive cells and ineffective clearance of virally infected cells [361, 362]. It has been reported that despite normal expression levels of lytic molecules, the cytotoxic CD8+ T cells from WAS patients failed to effectively kill B-cell lymphoma target cells due to inefficient polarization of cytotoxic granules toward the target tumor cells [356]. Recently, activating mutations in WASp (which give rise to XLN) have been found to lead to genetic instability through dysregulation of actin polymerization. Enhanced and delocalized actin polymerization throughout the cell was shown to inhibit myelopoiesis through defective mitosis and cytokinesis, with micronuclei formation indicative of genomic instability [363]. Despite lack of direct evidence, genomic instability might contribute to the development of malignancies in WAS patients [347].
Early HSCT is the treatment of choice for patients with classic WAS, preferably from a matched related donor [364]. Furthermore, immune reconstitution in WAS patients following HSCT leads to a decrease in cancer risk [364]. Gene therapy is an alternative to HSCT in the treatment of WAS [365]; however, the long-term outcome needs to be further monitored. This could be explained by the fact that the viruses used for therapy integrate in the host genome, with preferential insertion at transcription start sites, promoter and enhancer regions of active genes, and at conserved noncoding DNA, resulting in a high rate of transformations and the development of secondary malignancies [366, 367]. Acute T-cell leukemia due to vector insertion in the vicinity of the T-cell oncogene LMO2 has been reported in one patient [368, 369].
18.8.4 Chromosome 22q11.2 Deletion Syndrome
Chromosome 22q11.2 deletion syndrome is relatively common (estimated in 1 in 4,000 births) [370], and about 6 % of newly diagnosed cases are familial [371]. The presenting symptoms of chromosome 22q11.2 deletion syndrome vary depending on the patients’ age. While developmental delay and speech issues are the usual presenting symptoms in older children and adults, cardiac anomalies, hypocalcemia, and infection are the major manifestations in infants. Cardiac defects are seen in approximately 80 % of patients; on the other hand, tetralogy of Fallot and interrupted aortic arch type B have a strong positive predictive value for chromosome 22q11.2 deletion syndrome [372, 373]. Palatal dysfunction, feeding problems, facial dysmorphism, renal anomalies, and gastrointestinal manifestations also are seen in most of these patients [374]. Patients are also at an increased risk of atopy and autoimmune disease development [375, 376].
The immune system is affected in approximately 75 % of the patients [374, 376, 377]. The severity ranges from absent thymic tissue and no circulating T cells to completely normal T-cell counts. Many infants with low T-cell counts will demonstrate improvement in the first year of life, but after that, T-cell counts decline [378]. Patients may also suffer from variable degrees of B-cell defects [379, 380]. In a cohort of 687 patients with chromosome 22q11.2 deletion syndrome, six cases of malignancy were identified. This gives an overall frequency of 0.9 % (900 per 100,000) in this large pediatric group of patients, whereas the overall risk of malignancy in children under the age of 14 years is 3.4 per 100,000 children [381]. As reported in the literature, patients with chromosome 22q11.2 deletion syndrome have a clearly increased risk of lymphoma, particularly B-cell lymphoma [382–385]. This is a general phenomenon in patients with severe immunodeficiency. There have also been reports of myelodysplasia, acute lymphoblastic leukemia, SCC, astrocytoma, neuroblastoma, hepatoblastoma, renal cell carcinoma, and rhabdoid tumors [381, 386–390].
18.9 Concluding Remarks
The expanded life expectancy of patients with PIDs has increased the overall risk for developing cancers. However, the management of cancers in such patients remains challenging, in part due to the rarity, the increased risk for infection and other complications, as well as the rather slow pace of scientific advancement related to these conditions. Continued progress in understanding the interplay between chronic Ag stimulation, oncogenic viruses, genetic factors, and impaired host immunity during tumor formation in various PIDs will facilitate refinement of current and emerging therapeutic approaches.
References
1.
Al-Herz W, Bousfiha A, Casanova JL, Chapel H, Conley ME, Cunningham-Rundles C, et al. Primary immunodeficiency diseases: an update on the classification from the international union of immunological societies expert committee for primary immunodeficiency. Front Immunol. 2011;2:54.PubMedCentralPubMed
2.
Salavoura K, Kolialexi A, Tsangaris G, Mavrou A. Development of cancer in patients with primary immunodeficiencies. Anticancer Res. 2008;28(2B):1263–9.PubMed
3.
Mueller BU, Pizzo PA. Cancer in children with primary or secondary immunodeficiencies. J Pediatr. 1995;126(1):1–10.PubMed
4.
Filipovich AH, Mathur A, Kamat D, Shapiro RS. Primary immunodeficiencies: genetic risk factors for lymphoma. Cancer Res. 1992;52(19 Suppl):5465s–7.PubMed
5.
Dunn GP, Old LJ, Schreiber RD. The immunobiology of cancer immunosurveillance and immunoediting. Immunity. 2004;21(2):137–48.PubMed
6.
Martin D, Gutkind JS. Human tumor-associated viruses and new insights into the molecular mechanisms of cancer. Oncogene. 2008;27 Suppl 2:S31–42.PubMed
7.
Philip M, Rowley DA, Schreiber H. Inflammation as a tumor promoter in cancer induction. Semin Cancer Biol. 2004;14(6):433–9.PubMed
8.
Bartkova J, Horejsí Z, Koed K, Krämer A, Tort F, Zieger K, et al. DNA damage response as a candidate anti-cancer barrier in early human tumorigenesis. Nature. 2005;434(7035):864–70.PubMed
9.
Tran H, Nourse J, Hall S, Green M, Griffiths L, Gandhi MK. Immunodeficiency-associated lymphomas. Blood Rev. 2008;22(5):261–81.PubMed
10.
Chakraborty R, Sankaranarayanan K. Cancer predisposition, radiosensitivity and the risk of radiation-induced cancers II. A Mendelian single-locus model of cancer predisposition and radiosensitivity for predicting cancer risks in populations. Radiat Res. 1995;143(3):293–301.PubMed
11.
Kamani NR, Kumar S, Hassebroek A, Eapen M, LeRademacher J, Casper J, et al. Malignancies after hematopoietic cell transplantation for primary immune deficiencies: a report from the Center for International Blood and Marrow Transplant Research. Biol Blood Marrow Transplant. 2011;17(12):1783–9.PubMedCentralPubMed
12.
Hammarström L, Vorechovsky I, Webster D. Selective IgA deficiency (SIgAD) and common variable immunodeficiency (CVID). Clin Exp Immunol. 2000;120(2):225–31.PubMedCentralPubMed
13.
Chua I, Quinti I, Grimbacher B. Lymphoma in common variable immunodeficiency: interplay between immune dysregulation, infection and genetics. Curr Opin Hematol. 2008;15(4):368–74.PubMed
14.
Cunningham-Rundles C. How I treat common variable immune deficiency. Blood. 2010;116(1):7–15.PubMedCentralPubMed
15.
Cunningham-Rundles C, Bodian C. Common variable immunodeficiency: clinical and immunological features of 248 patients. Clin Immunol. 1999;92(1):34–48.PubMed
16.
Kinlen LJ, Webster AD, Bird AG, Haile R, Peto J, Soothill JF, et al. Prospective study of cancer in patients with hypogammaglobulinaemia. Lancet. 1985;1(8423):263–6.PubMed
17.
Cunningham-Rundles C, Siegal FP, Cunningham-Rundles S, Lieberman P. Incidence of cancer in 98 patients with common varied immunodeficiency. J Clin Immunol. 1987;7(4):294–9.PubMed
18.
Hermaszewski RA, Webster AD. Primary hypogammaglobulinaemia: a survey of clinical manifestations and complications. Q J Med. 1993;86(1):31–42.PubMed
19.
Zullo A, Romiti A, Rinaldi V, Vecchione A, Tomao S, Aiuti F, et al. Gastric pathology in patients with common variable immunodeficiency. Gut. 1999;45(1):77–81.PubMedCentralPubMed
20.
Grimbacher B, Hutloff A, Schlesier M, Glocker E, Warnatz K, Dräger R, et al. Homozygous loss of ICOS is associated with adult-onset common variable immunodeficiency. Nat Immunol. 2003;4(3):261–8.PubMed
21.
Salzer U, Chapel HM, Webster AD, Pan-Hammarström Q, Schmitt-Graeff A, Schlesier M, et al. Mutations in TNFRSF13B encoding TACI are associated with common variable immunodeficiency in humans. Nat Genet. 2005;37(8):820–8.PubMed
22.
Castigli E, Wilson SA, Garibyan L, Rachid R, Bonilla F, Schneider L, et al. TACI is mutant in common variable immunodeficiency and IgA deficiency. Nat Genet. 2005;37(8):829–34.PubMed
23.
Warnatz K, Salzer U, Rizzi M, Fischer B, Gutenberger S, Böhm J, et al. B-cell activating factor receptor deficiency is associated with an adult-onset antibody deficiency syndrome in humans. Proc Natl Acad Sci U S A. 2009;106(33):13945–50.PubMedCentralPubMed
24.
van Zelm MC, Reisli I, van der Burg M, Castaño D, van Noesel CJ, van Tol MJ, et al. An antibody-deficiency syndrome due to mutations in the CD19 gene. N Engl J Med. 2006;354(18):1901–12.PubMed
25.
Kuijpers TW, Bende RJ, Baars PA, Grummels A, Derks IA, Dolman KM, et al. CD20 deficiency in humans results in impaired T cell-independent antibody responses. J Clin Invest. 2010;120(1):214–22.PubMedCentralPubMed
26.
van Zelm MC, Smet J, Adams B, Mascart F, Schandené L, Janssen F, et al. CD81 gene defect in humans disrupts CD19 complex formation and leads to antibody deficiency. J Clin Invest. 2010;120(4):1265–74.PubMedCentralPubMed
27.
Thiel J, Kimmig L, Salzer U, Grudzien M, Lebrecht D, Hagena T, et al. Genetic CD21 deficiency is associated with hypogammaglobulinemia. J Allergy Clin Immunol. 2012;129(3):801–10.e6.PubMed
28.
Lopez-Herrera G, Tampella G, Pan-Hammarström Q, Herholz P, Trujillo-Vargas CM, Phadwal K, et al. Deleterious mutations in LRBA are associated with a syndrome of immune deficiency and autoimmunity. Am J Hum Genet. 2012;90(6):986–1001.PubMedCentralPubMed
29.
Cunningham-Rundles C. Clinical and immunologic analyses of 103 patients with common variable immunodeficiency. J Clin Immunol. 1989;9(1):22–33.PubMed
30.
Boncristiano M, Majolini MB, D’Elios MM, Pacini S, Valensin S, Ulivieri C, et al. Defective recruitment and activation of ZAP-70 in common variable immunodeficiency patients with T cell defects. Eur J Immunol. 2000;30(9):2632–8.
31.
Gulbranson-Judge A, Tybulewicz VL, Walters AE, Toellner KM, MacLennan IC, Turner M. Defective immunoglobulin class switching in Vav-deficient mice is attributable to compromised T cell help. Eur J Immunol. 1999;29(2):477–87.PubMed
32.
Baumert E, Wolff-Vorbeck G, Schlesier M, Peter HH. Immunophenotypical alterations in a subset of patients with common variable immunodeficiency (CVID). Clin Exp Immunol. 1992;90(1):25–30.PubMedCentralPubMed
33.
Holm AM, Sivertsen EA, Tunheim SH, Haug T, Bjerkeli V, Yndestad A, et al. Gene expression analysis of peripheral T cells in a subgroup of common variable immunodeficiency shows predominance of CCR7(-) effector-memory T cells. Clin Exp Immunol. 2004;138(2):278–89.PubMedCentralPubMed
34.
North ME, Webster AD, Farrant J. Primary defect in CD8+ lymphocytes in the antibody deficiency disease (common variable immunodeficiency): abnormalities in intracellular production of interferon-gamma (IFN-gamma) in CD28+ (‘cytotoxic’) and CD28- (‘suppressor’) CD8+ subsets. Clin Exp Immunol. 1998;111(1):70–5.PubMedCentralPubMed
35.
McQuaid A, Tormey VJ, Trafford B, Webster AD, Bofill M. Evidence for increased expression of regulatory cytokine receptors interleukin-12R and interleukin-18R in common variable immunodeficiency. Clin Exp Immunol. 2003;134(2):321–7.PubMedCentralPubMed
36.
Bayry J, Hermine O, Webster DA, Lévy Y, Kaveri SV. Common variable immunodeficiency: the immune system in chaos. Trends Mol Med. 2005;11(8):370–6.PubMed
37.
Farrington M, Grosmaire LS, Nonoyama S, Fischer SH, Hollenbaugh D, Ledbetter JA, et al. CD40 ligand expression is defective in a subset of patients with common variable immunodeficiency. Proc Natl Acad Sci U S A. 1994;91(3):1099–103.PubMedCentralPubMed
38.
Aspalter RM, Sewell WA, Dolman K, Farrant J, Webster AD. Deficiency in circulating natural killer (NK) cell subsets in common variable immunodeficiency and X-linked agammaglobulinaemia. Clin Exp Immunol. 2000;121(3):506–14.PubMedCentralPubMed
39.
Fulcher DA, Avery DT, Fewings NL, Berglund LJ, Wong S, Riminton DS, et al. Invariant natural killer (iNK) T cell deficiency in patients with common variable immunodeficiency. Clin Exp Immunol. 2009;157(3):365–9.PubMedCentralPubMed
40.
Trujillo CM, Muskus C, Arango J, Patiño PJ, Montoya CJ. Quantitative and functional evaluation of innate immune responses in patients with common variable immunodeficiency. J Investig Allergol Clin Immunol. 2011;21(3):207–15.PubMed
41.
Desar IM, Keuter M, Raemaekers JM, Jansen JB, van Krieken JH, van der Meer JW. Extranodal marginal zone (MALT) lymphoma in common variable immunodeficiency. Neth J Med. 2006;64(5):136–40.PubMed
42.
Hussell T, Isaacson PG, Crabtree JE, Spencer J. The response of cells from low-grade B-cell gastric lymphomas of mucosa-associated lymphoid tissue to Helicobacter pylori. Lancet. 1993;342(8871):571–4.PubMed
43.
Wheat WH, Cool CD, Morimoto Y, Rai PR, Kirkpatrick CH, Lindenbaum BA, et al. Possible role of human herpesvirus 8 in the lymphoproliferative disorders in common variable immunodeficiency. J Exp Med. 2005;202(4):479–84.PubMedCentralPubMed
44.
Raeiszadeh M, Kopycinski J, Paston SJ, Diss T, Lowdell M, Hardy GA, et al. The T cell response to persistent herpes virus infections in common variable immunodeficiency. Clin Exp Immunol. 2006;146(2):234–42.PubMedCentralPubMed
45.
He B, Chadburn A, Jou E, Schattner EJ, Knowles DM, Cerutti A. Lymphoma B cells evade apoptosis through the TNF family members BAFF/BLyS and APRIL. J Immunol. 2004;172(5):3268–79.PubMed
46.
Kern C, Cornuel JF, Billard C, Tang R, Rouillard D, Stenou V, et al. Involvement of BAFF and APRIL in the resistance to apoptosis of B-CLL through an autocrine pathway. Blood. 2004;103(2):679–88.PubMed
47.
Palanduz S, Palanduz A, Yalcin I, Somer A, Ones U, Ustek D, et al. In vitro chromosomal radiosensitivity in common variable immune deficiency. Clin Immunol Immunopathol. 1998;86(2):180–2.PubMed
48.
Vorechovský I, Scott D, Haeney MR, Webster DA. Chromosomal radiosensitivity in common variable immune deficiency. Mutat Res. 1993;290(2):255–64.PubMed
49.
Lederman HM, Winkelstein JA. X-linked agammaglobulinemia: an analysis of 96 patients. Medicine (Baltimore). 1985;64(3):145–56.
50.
Ochs HD, Smith CI. X-linked agammaglobulinemia. A clinical and molecular analysis. Medicine (Baltimore). 1996;75(6):287–99.
51.
McKinney RE, Katz SL, Wilfert CM. Chronic enteroviral meningoencephalitis in agammaglobulinemic patients. Rev Infect Dis. 1987;9(2):334–56.PubMed
52.
Vihinen M, Mattsson PT, Smith CI. Bruton tyrosine kinase (BTK) in X-linked agammaglobulinemia (XLA). Front Biosci. 2000;5:D917–28.PubMed
53.
Aoki Y, Isselbacher KJ, Pillai S. Bruton tyrosine kinase is tyrosine phosphorylated and activated in pre-B lymphocytes and receptor-ligated B cells. Proc Natl Acad Sci U S A. 1994;91(22):10606–9.PubMedCentralPubMed
54.
Afar DE, Park H, Howell BW, Rawlings DJ, Cooper J, Witte ON. Regulation of Btk by Src family tyrosine kinases. Mol Cell Biol. 1996;16(7):3465–71.PubMedCentralPubMed
55.
Takata M, Kurosaki T. A role for Bruton’s tyrosine kinase in B cell antigen receptor-mediated activation of phospholipase C-gamma 2. J Exp Med. 1996;184(1):31–40.PubMed
56.
Scharenberg AM, El-Hillal O, Fruman DA, Beitz LO, Li Z, Lin S, et al. Phosphatidylinositol-3,4,5-trisphosphate (PtdIns-3,4,5-P3)/Tec kinase-dependent calcium signaling pathway: a target for SHIP-mediated inhibitory signals. EMBO J. 1998;17(7):1961–72.PubMedCentralPubMed
57.
Uckun FM, Waddick KG, Mahajan S, Jun X, Takata M, Bolen J, et al. BTK as a mediator of radiation-induced apoptosis in DT-40 lymphoma B cells. Science. 1996;273(5278):1096–100.PubMed
58.
Uckun F, Ozer Z, Vassilev A. Bruton’s tyrosine kinase prevents activation of the anti-apoptotic transcription factor STAT3 and promotes apoptosis in neoplastic B-cells and B-cell precursors exposed to oxidative stress. Br J Haematol. 2007;136(4):574–89.PubMed
59.
Doyle SL, Jefferies CA, Feighery C, O’Neill LA. Signaling by Toll-like receptors 8 and 9 requires Bruton’s tyrosine kinase. J Biol Chem. 2007;282(51):36953–60.PubMed
60.
Sochorová K, Horváth R, Rozková D, Litzman J, Bartunková J, Sedivá A, et al. Impaired Toll-like receptor 8-mediated IL-6 and TNF-alpha production in antigen-presenting cells from patients with X-linked agammaglobulinemia. Blood. 2007;109(6):2553–6.PubMed
61.
Brosens LA, Tytgat KM, Morsink FH, Sinke RJ, Ten Berge IJ, Giardiello FM, et al. Multiple colorectal neoplasms in X-linked agammaglobulinemia. Clin Gastroenterol Hepatol. 2008;6(1):115–9.PubMed
62.
van der Meer JW, Weening RS, Schellekens PT, van Munster IP, Nagengast FM. Colorectal cancer in patients with X-linked agammaglobulinaemia. Lancet. 1993;341(8858):1439–40.PubMed
63.
James RG, Biechele TL, Conrad WH, Camp ND, Fass DM, Major MB, et al. Bruton’s tyrosine kinase revealed as a negative regulator of Wnt-beta-catenin signaling. Sci Signal. 2009;2(72):ra25.PubMed
64.
Reya T, Clevers H. Wnt signalling in stem cells and cancer. Nature. 2005;434(7035):843–50.PubMed
65.
Lavilla P, Gil A, Rodríguez MC, Dupla ML, Pintado V, Fontán G. X-linked agammaglobulinemia and gastric adenocarcinoma. Cancer. 1993;72(5):1528–31.PubMed
66.
Echave-Sustaeta JM, Villena V, Verdugo M, López-Encuentra A, de Agustín P, Alberti N. X-linked agammaglobulinaemia and squamous lung cancer. Eur Respir J. 2001;17(3):570–2.PubMed
67.
Al-Attas RA, Rahi AH. Primary antibody deficiency in Arabs: first report from eastern Saudi Arabia. J Clin Immunol. 1998;18(5):368–71.PubMed
68.
Kanoh T, Mizumoto T, Yasuda N, Koya M, Ohno Y, Uchino H, et al. Selective IgA deficiency in Japanese blood donors: frequency and statistical analysis. Vox Sang. 1986;50(2):81–6.PubMed
69.
Conley ME, Notarangelo LD, Etzioni A. Diagnostic criteria for primary immunodeficiencies. Representing PAGID (Pan-American Group for Immunodeficiency) and ESID (European Society for Immunodeficiencies). Clin Immunol. 1999;93(3):190–7.PubMed
71.
Jacob CM, Pastorino AC, Fahl K, Carneiro-Sampaio M, Monteiro RC. Autoimmunity in IgA deficiency: revisiting the role of IgA as a silent housekeeper. J Clin Immunol. 2008;28 Suppl 1:S56–61.PubMed
72.
Ballow M. Primary immunodeficiency disorders: antibody deficiency. J Allergy Clin Immunol. 2002;109(4):581–91.PubMed
73.
Cunningham-Rundles C. Physiology of IgA and IgA deficiency. J Clin Immunol. 2001;21(5):303–9.PubMed
74.
Chow MA, Lebwohl B, Reilly NR, Green PH. Immunoglobulin A deficiency in celiac disease. J Clin Gastroenterol. 2012;46(10):850–4.PubMed
75.
Dalgic B, Sari S, Basturk B, Ensari A, Egritas O, Bukulmez A, et al. Prevalence of celiac disease in healthy Turkish school children. Am J Gastroenterol. 2011;106(8):1512–7.PubMed
76.
Haimila K, Einarsdottir E, de Kauwe A, Koskinen LL, Pan-Hammarström Q, Kaartinen T, et al. The shared CTLA4-ICOS risk locus in celiac disease, IgA deficiency and common variable immunodeficiency. Genes Immun. 2009;10(2):151–61.PubMed
77.
Wang N, Hammarström L. IgA deficiency: what is new? Curr Opin Allergy Clin Immunol. 2012;12(6):602–8.PubMed
78.
Zenone T, Souquet PJ, Cunningham-Rundles C, Bernard JP. Hodgkin’s disease associated with IgA and IgG subclass deficiency. J Intern Med. 1996;240(2):99–102.PubMed
79.
Cunningham-Rundles C, Pudifin DJ, Armstrong D, Good RA. Selective IgA deficiency and neoplasia. Vox Sang. 1980;38(2):61–7.PubMed
81.
Buckley RH. Clinical and immunologic features of selective IgA deficiency. Birth Defects Orig Artic Ser. 1975;11(1):134–42.PubMed
82.
De Laat PC, Weemaes CM, Gonera R, Van Munster PJ, Bakkeren JA, Stoelinga GB. Clinical manifestations in selective IgA deficiency in childhood. A follow-up report. Acta Paediatr Scand. 1991;80(8–9):798–804.PubMed
83.
Lee CH, Quin JW, Wong CS, Grace CS, Rozenberg MC. IgA deficiency, superior mediastinal tumour with unusual clinical manifestations. Aust N Z J Med. 1979;9(3):306–9.PubMed
84.
Hamoudi AB, Ertel I, Newton WA, Reiner CB, Clatworthy HW. Multiple neoplasms in an adolescent child associated with IGA deficiency. Cancer. 1974;33(4):1134–44.PubMed
85.
Shkalim V, Monselize Y, Segal N, Zan-Bar I, Hoffer V, Garty BZ. Selective IgA deficiency in children in Israel. J Clin Immunol. 2010;30(5):761–5.PubMed
86.
Huck K, Feyen O, Niehues T, Rüschendorf F, Hübner N, Laws HJ, et al. Girls homozygous for an IL-2-inducible T cell kinase mutation that leads to protein deficiency develop fatal EBV-associated lymphoproliferation. J Clin Invest. 2009;119(5):1350–8.PubMedCentralPubMed
87.
Stepensky P, Weintraub M, Yanir A, Revel-Vilk S, Krux F, Huck K, et al. IL-2-inducible T-cell kinase deficiency: clinical presentation and therapeutic approach. Haematologica. 2011;96(3):472–6.PubMedCentralPubMed
88.
AB. New forms of EBV-associated lymphoproliferation and their treatment by allo SCT. In: 3rd Meeting on clinical immunology, allergy and immunodeficiencies. Tehran: 2010.
89.
Linka RM, Huck K, Krux F, Stepenski P, Synaeve C, Vettenranta K, et al. Germline mutations within the IL2-inducible T cell kinase impede T cell differentiation or survival, cause protein destabilisation, loss of membrane recruitment and lead to severe EBV lymphoproliferation. In: 53rd ASH Annual Meeting and Exposition. Orlando: 2010.
90.
Mansouri D, Mahdaviani SA, Khalilzadeh S, Mohajerani SA, Hasanzad M, Sadr S, et al. IL-2-inducible T-cell kinase deficiency with pulmonary manifestations due to disseminated Epstein-Barr virus infection. Int Arch Allergy Immunol. 2012;158(4):418–22.PubMed
91.
Berg LJ, Finkelstein LD, Lucas JA, Schwartzberg PL. Tec family kinases in T lymphocyte development and function. Annu Rev Immunol. 2005;23:549–600.PubMed
92.
Felices M, Falk M, Kosaka Y, Berg LJ. Tec kinases in T cell and mast cell signaling. Adv Immunol. 2007;93:145–84.PubMed
93.
Felices M, Berg LJ. The Tec kinases Itk and Rlk regulate NKT cell maturation, cytokine production, and survival. J Immunol. 2008;180(5):3007–18.PubMed
94.
Schaeffer EM, Broussard C, Debnath J, Anderson S, McVicar DW, Schwartzberg PL. Tec family kinases modulate thresholds for thymocyte development and selection. J Exp Med. 2000;192(7):987–1000.PubMedCentralPubMed
95.
Prince AL, Yin CC, Enos ME, Felices M, Berg LJ. The Tec kinases Itk and Rlk regulate conventional versus innate T-cell development. Immunol Rev. 2009;228(1):115–31.PubMedCentralPubMed
96.
Bachmann MF, Littman DR, Liao XC. Antiviral immune responses in Itk-deficient mice. J Virol. 1997;71(10):7253–7.PubMedCentralPubMed
97.
Atherly LO, Brehm MA, Welsh RM, Berg LJ. Tec kinases Itk and Rlk are required for CD8+ T cell responses to virus infection independent of their role in CD4+ T cell help. J Immunol. 2006;176(3):1571–81.PubMed
98.
Khurana D, Arneson LN, Schoon RA, Dick CJ, Leibson PJ. Differential regulation of human NK cell-mediated cytotoxicity by the tyrosine kinase Itk. J Immunol. 2007;178(6):3575–82.PubMed
99.
Rezaei N, Hedayat M, Aghamohammadi A, Nichols KE. Primary immunodeficiency diseases associated with increased susceptibility to viral infections and malignancies. J Allergy Clin Immunol. 2011;127(6):1329–41.e2. quiz 42–3.PubMed
100.
Markert ML. Purine nucleoside phosphorylase deficiency. Immunodefic Rev. 1991;3(1):45–81.PubMed
101.
Cohen A, Cohen A, Grunebaum E, Arpaia E, Roifman CM. Immunodeficiency caused by purine nucleoside phosphorylase deficiency. Immunol Allergy Clin North Am. 2000;20(1):143–59.
102.
Pannicke U, Tuchschmid P, Friedrich W, Bartram CR, Schwarz K. Two novel missense and frameshift mutations in exons 5 and 6 of the purine nucleoside phosphorylase (PNP) gene in a severe combined immunodeficiency (SCID) patient. Hum Genet. 1996;98(6):706–9.PubMed
103.
Banzhoff A, Schauer U, Riedel F, Gahr M, Rieger CH. Fatal varicella in a 5-year-old boy. Eur J Pediatr. 1997;156(4):333–4.PubMed
104.
Gelfand EW, Dosch HM, Biggar WD, Fox IH. Partial purine nucleoside phosphorylase deficiency. Studies of lymphocyte function. J Clin Invest. 1978;61(4):1071–80.PubMedCentralPubMed
105.
Andrews LG, Markert ML. Exon skipping in purine nucleoside phosphorylase mRNA processing leading to severe immunodeficiency. J Biol Chem. 1992;267(11):7834–8.PubMed
106.
Aust MR, Andrews LG, Barrett MJ, Norby-Slycord CJ, Markert ML. Molecular analysis of mutations in a patient with purine nucleoside phosphorylase deficiency. Am J Hum Genet. 1992;51(4):763–72.PubMedCentralPubMed
107.
Tam DA, Leshner RT. Stroke in purine nucleoside phosphorylase deficiency. Pediatr Neurol. 1995;12(2):146–8.PubMed
108.
Carpenter PA, Ziegler JB, Vowels MR. Late diagnosis and correction of purine nucleoside phosphorylase deficiency with allogeneic bone marrow transplantation. Bone Marrow Transplant. 1996;17(1):121–4.PubMed
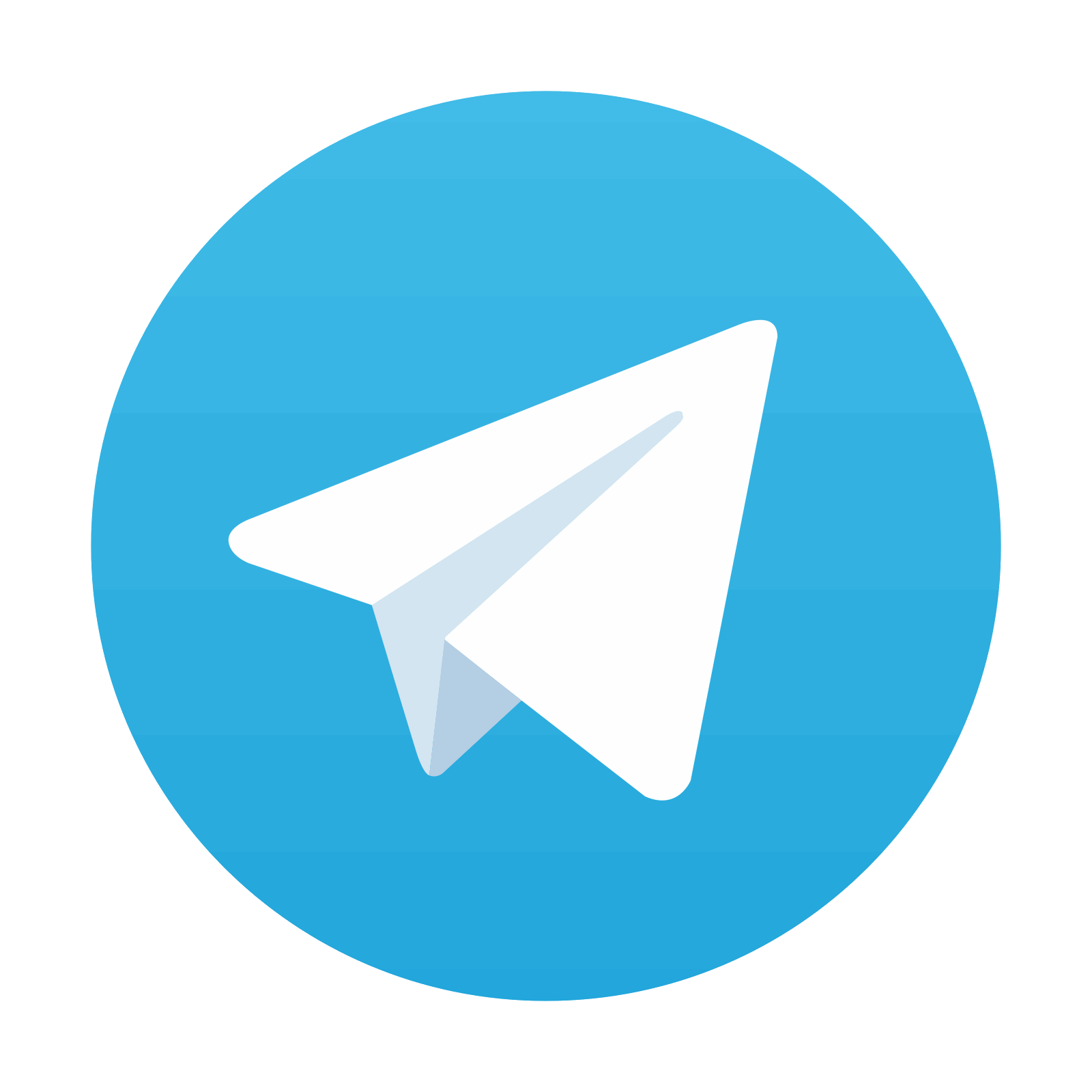
Stay updated, free articles. Join our Telegram channel

Full access? Get Clinical Tree
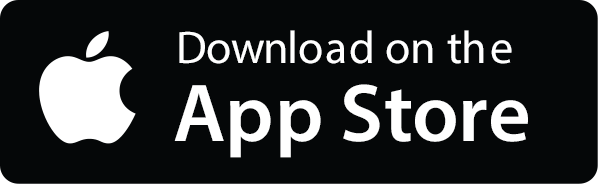
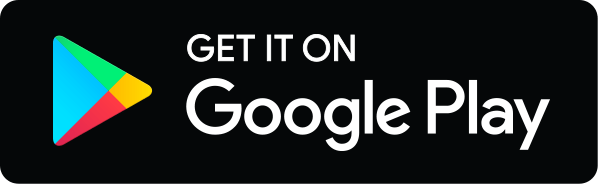