Fig. 12.1
Cancer immunoediting process with its three Es of elimination, equilibrium, and escape. Please note that although in many cases the sequence is followed, in others one or the other phase may be skipped. Although the events from equilibrium phase may proceed either toward escape or back to the elimination phase, the reversibility of the escape phase with or without therapy to other two phases is questionable
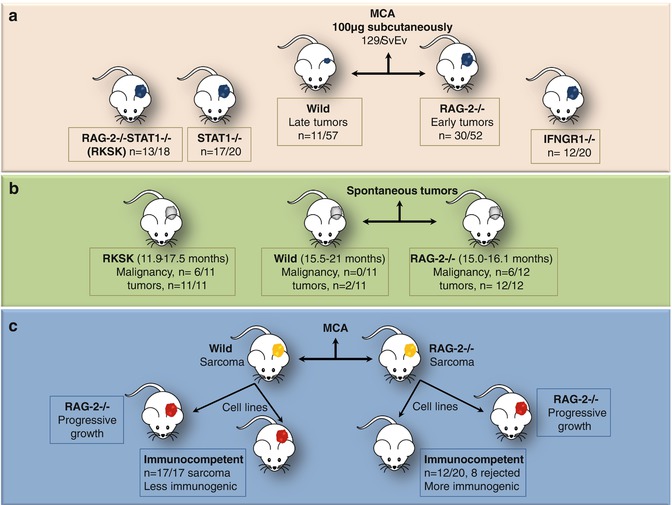
Fig. 12.2
Mice experiments by Shankaran et al. [6] demonstrating surveillance and sculpting roles of immune system. (a) Immunodeficient (RAG-2−/−/IFNGR1/STAT1−/− or combined RAG-2−/− STAT1−/−, RkSk) mice developed tumors earlier than wild type and with greater frequency on subcutaneous injection of MCA, thus necessitating the presence of intact T, NKT, and B cells for prevention of chemically induced tumors. (b) Spontaneous tumor development was also observed to be higher in RAG-2−/− and RkSk mice as compared to unmanipulated 129/SvEv wild-type mice. Moreover, the later merely developed benign tumors and no malignancy was noted. (c) Furthermore, cells were taken from MCA-induced tumors in wild and RAG-2−/− mice and were injected into immunocompetent and RAG-2−/− mice. Progressive tumor growth was noted in immunodeficient mice transplanted with sarcoma cells derived from wild or RAG-2−/− mice. The immunocompetent mice transplanted with sarcoma cells from wild mice also showed progressive tumor growth; however, many mice transplanted with sarcoma cells derived from RAG-2−/− mice rejected the transplanted tumor cells. This occurred due to sculpting of sarcoma by the immune system in wild mice, thus rendering it less immunogenic. Tumors from the immunodeficient mice which were not edited were more immunogenic and thus were rejected by immunocompetent mice
12.2.1 Immune Elimination: Evidences For and Against
The immune elimination phase of cancer immunoediting is sine qua non of the original immunosurveillance process. It envisages the destruction or eradication of cancer by the host immune system and is believed to occur when a cell gets transformed by overcoming its intrinsic tumor suppressor mechanisms, before being able to establish a full-blown tumor. Although the existence of such a phenomenon has been hypothesized since long, the early experiments carried out on nude mice models which are only partially immunodeficient failed to prove it. The definitive experimental proof to its presence came from the work of Shankaran et al. in the last decade (Table 12.1, Fig. 12.2) [6]. However, despite the experimental evidence of its presence in mice, it has been difficult to demonstrate it in the clinical scenario. Still, the data obtained from various cancer registries wherein a higher cancer incidence especially of viral etiology has been observed in immunosuppressed transplant recipients suggests its existence in human subjects as well. Currently, a similar trend has been noticed in the setting of acquired immunodeficiency syndrome [13,14]. The proponents of this stage in cancer immunity state that many of the cell transformation events occurring in our body may be removed quietly by the immune system without us ever being aware about it. Spontaneous regression has been reported in some tumors including cutaneous melanoma, retinoblastoma, osteosarcoma, etc., in humans [15]. Studies have shown that both innate as well as adaptive immune response contribute to fighting off the cancer from our body.
Table 12.1
Timeline of events depicting evolution of cancer immunity from immunosurveillance to immunoediting
Study | Hypothesis/observation/experimental evidence | Results |
---|---|---|
William B Cooley (1891) [10] | Injected cultures of heat-inactivated bacteria or bacterial culture supernatants into cancer patients | Demonstrated marked regression of tumors and prolonged survival after the treatment |
Paul Ehrlich (1909) [1] | Immune system protects the host from malignancy | Gave birth to the idea of immune control of malignancies |
Burnet and Thomas (1957) [2] | Immune system must be removing the carcinogenic events arising out of ongoing evolutionary genetic remodeling taking place in an individual | Formal emergence of immunosurveillance hypothesis |
Several groups (1965–1973) | Induced immunodeficiency by thymectomy or heterologous antilymphocyte serum or pharmacological agents. Immunodeficient animals are more prone to develop cancers | No consensus regarding immunosurveillance |
Stutman O (1975) [11] | The methylcholanthrene (MCA)-induced cancer incidence in immunodeficient nude athymic mice was not higher than the control mice | Rejection of immunosurveillance hypothesis |
Kaplan et al. (1998) [12] | IFN-γ and perforin deficient animals were more prone to MCA-induced tumors as compared to controls | Resurrection of immunosurveillance in cancer |
Shankaran et al. (2001) [6] | Experiments in RAG-2 null mice (lacking T, B, and NKT cells) revealed higher incidence of both MCA-induced sarcomas and spontaneous epithelial tumors in these animals | Definitive evidence of existence of cancer immunosurveillance |
Dunn et al. (2002) [3] | Concept of cancer immunoediting to explain the tumor sculpting role of immune system | Coined the term immune elimination as a part of broader concept of cancer immunoediting with 3 Es of elimination, equilibrium, and escape |
12.2.1.1 The Key Players in Anticancer Immunity
The key players responsible for launching an effective immune response against cancer include the immune cells and soluble molecules secreted into the tumor milieu (Fig. 12.3). In case, the tumor exhibits high immunogenicity, a specific immune response occurs against it. However, if tumor immunogenicity is low, the nonspecific effector responses gain importance.
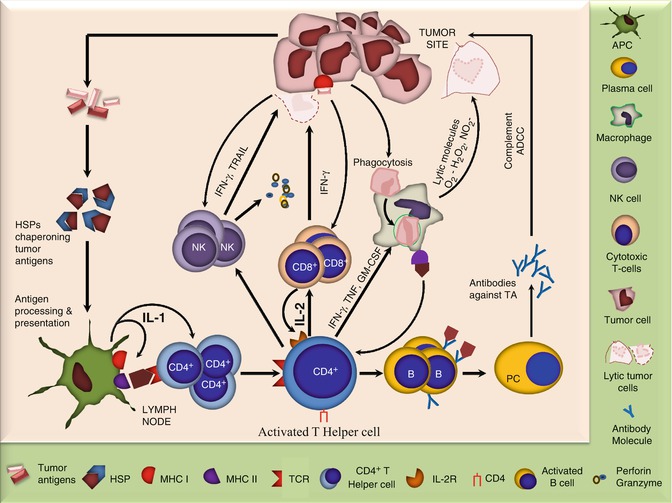
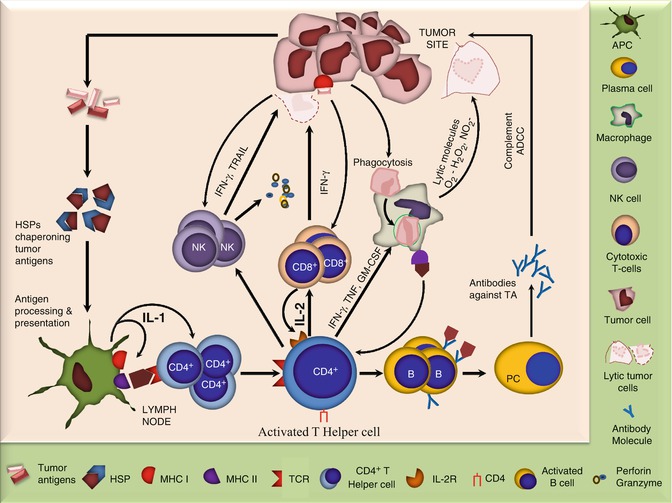
Fig. 12.3
Diagram showing key players involved in antitumor immune response. The tumor releases Ags which are chaperoned by heat-shock proteins and taken up by the APCs which process them and present to CD4+ T cells. The later being the central point of immune response activate various other cells including NK cells, CD8+ cells, macrophages, and B cells which act in various ways to counteract the tumors. In addition, tumors may directly activate the cytotoxic cells including CD8+ and NK cells and phagocytic cells. While the former two can cause direct tumor lysis primarily via perforin and granzymes, the later may engulf tumor cells and kill them by releasing lytic molecules or may process and present tumor Ags to CD4+ T cells
The major cell types involved in an antitumor immune response are adoptive T cells, which not only kill tumor cells directly with the help of TNF-α but are also essential for the activation of other components of the immune machinery. The CD8+ cytotoxic lymphocytes (CTLs) are able to directly recognize tumor cells which express MHC I and can also be activated by CD4+ T-helper cells. They may cause lysis of the tumor cells via perforin- and granzyme-dependent mechanisms. The CD4+ T cells also secrete factors to induce proliferation of B cells and to promote their differentiation to antibody (Ab)-secreting plasma cells. The later may contribute to antitumor immunity by complement-mediated lysis or by antibody-dependent cellular cytotoxity (ADCC). The CD4+ T-helper cells also activate macrophages by secreting IFN-γ, TNF, IL-4, and granulocyte-macrophage colony-stimulating factor (GM-CSF). The activated macrophages may phagocytize tumor cells and kill them by releasing toxic free radicals including O2 − and NO2 − or by becoming antigen-presenting cells (APCs) which present tumor antigens to CD4+ T cells such as dendritic cells (DCs). Natural killer (NK) cells also have the potential to directly recognize and destroy tumor cells via tumor necrosis factor-related apoptosis-inducing ligand (TRAIL) and IFN-γ-dependent mechanisms. Loss of MHC class I as commonly observed in tumors may be responsible for their increased susceptibility to NK-cell-mediated lysis. In addition, NK-cell activity may also be enhanced by IL-2 and IFN-γ produced by the CD4+ T-helper cells. NKT and γδ T cells also recognize the danger signals released from the tumors and become activated. The NKT cells especially the invariant or the type I NKT, which are CD4− CD8− and mainly recognize the lipid/glycolipid antigens (Ags) via CD1d molecule, have been recognized to protect against certain cancers. The protective role is however supposed to be indirectly exerted via secretion of IFN-γ and subsequent activation of NK and CD8+ T cells. The γδ T cells which represent 1–5 % of peripheral blood T cells are also reported to infiltrate and cause lysis of tumors, both in vitro and in vivo [16–20].
In various clinical studies on different cancers including colon, ovary, lung carcinomas, and melanoma, the tumor-infiltrating lymphocytes (TILs) have been associated with increased time to disease recurrence, an enhanced 5-year survival, and an overall good prognosis. Also, in a study on metastatic colorectal cancer, TIL density at the invasive margin was linked to a better chemotherapeutic response. Similarly, increased infiltration by CD3+ and CD8+ T cells, NK cells, and γδT cells has been correlated with improved outcomes in epithelial ovarian cancers. Some of the above studies have done quantitative assessment of the TILs in tumors, thus impressed upon the need to have a scoring system for TILs in order to determine the exact tumor behavior [21,22].
12.2.2 The Equilibrium Phase: The Most Controversial and the Least Understood Phase
This phase represents an intermediate stage of immune response in cancer. During this phase, the cancer and the immune system both coexist without allowing each other to dominate. The immune system cannot eliminate the cancer during this phase; however, it does not allow it to expand or metastasize. The cancer in turn is sculpted by the immune system, thus leading to the emergence of variants resistant to the immunological attack [3].
Various studies in mice have pointed toward the occurrence of the equilibrium phase in cancer immunity. In experiments on MCA-induced tumors in mice, Koebel et al. demonstrated the presence of inert lesions in healthy mice, which grew when subjected to immunological oppression (Fig. 12.4) [23]. The study served to be an important milestone in proving the existence of the equilibrium phase in cancers. Likewise, the tumors have been observed to stay dormant for decades after remission in human cancer patients, which is believed to be due to the fact that immune system keeps them in check. The immune system is believed to synergize with chemoradiotherapy in treatment-induced remission which renders the tumors silent. However, they relapse promptly after any kind of immune insult, thereby, further proving the presence of immune dormancy. The minimal residual disease commonly observed in hematological malignancies and the emerging donor-derived malignancies in immunosuppressed transplant recipients are considered two examples of the equilibrium phase in humans. Even though the immune system prevents monoclonal gammopathy of unknown significance (MGUS) from progressing to myeloma, it fails to eliminate the MGUS cells [24,25].
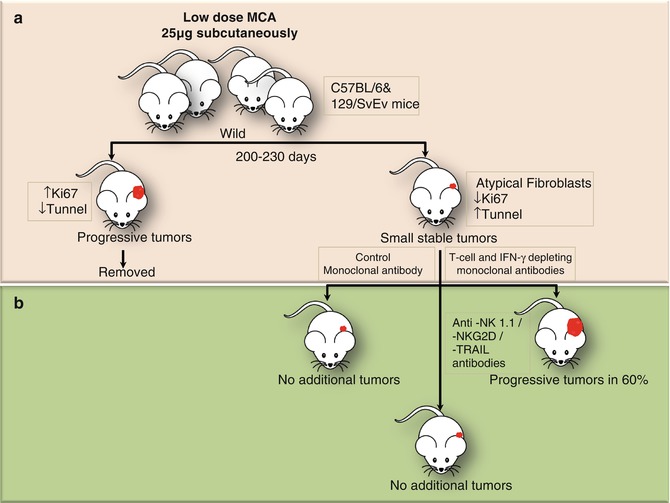
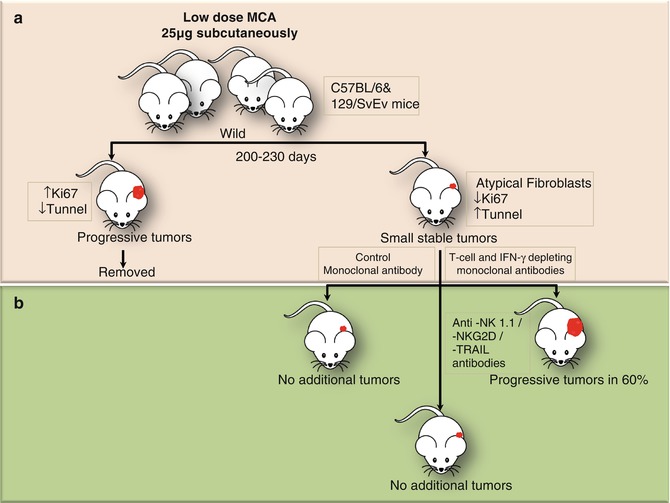
Fig. 12.4
Experiments conducted in mice by Koebel et al. demonstrating the presence of equilibrium phase in tumorigenesis. (a) Groups of wild-type C57BL/6 or 129/SvEv mice were injected with a single low dose of MCA. After monitoring for 200–230 days, the mice with rapidly growing sarcomas were set aside. (b) The remaining mice displaying small stable masses at injection site were injected with control Ab or mAbs depleting specific components of innate and adoptive immunity. The mice in former two groups did not develop any additional tumors; however, those in the last group (T cell and IFN-γ depleted) showed rapid tumor growth. This could only be explained by cancer immune equilibrium in which the tumors were not removed, but restricted by the immune process. However, on suppression of adoptive immunity progressive tumor growth was observed
Adoptive T cells, both CD4+ and CD8+, have been observed to play a pivotal role in cancer immune equilibrium. Immune-sufficient mice with inert tumors are shown to develop into full-fledged tumors only upon depletion of T cells/IFN-γ/IL-12. However, the depletion of innate immune cells was not found to result in the development of tumors. Moreover, tumor cells were found to be highly immunogenic during the equilibrium phase, as they are unedited by the immune system and become less immunogenic at the end of this phase [23,26,27].
In addition, the mechanisms including cellular and angiogenic dormancy also complement the immune system in maintaining cancer cells in the dormant state. In the former, the tumor cells hide themselves in specialized niches, become quiescent, and wait for the opportunity to regrow. In the later condition, expansion is not possible, due to the lack of adequate vascularization. When faced with favorable conditions, tumor cells come out of their slumber and undergo a series of genetic and epigenetic modifications which increase their immune resistance, eventually leading to the next phase of cancer immunity, known as immune escape. Studies are being conducted to identify the genetic and molecular signatures of dormant tumor cells which allow them to retain their dormant status or facilitate their escape [23,26–29].
12.2.3 Immune Escape: The Best Studied Phase
The escape phase represents the final and most extensively studied phase of the immunoediting process. The unleashing of mechanisms underlying the escape phase has formed the basis for the development of various therapeutic agents with the aim to stop the progress of the neoplastic process. Due to increasing genomic instability, cancer cells acquire various characteristics enabling them to ward off the immune process or to modify it in such a way which is beneficial to tumor cells. Tumors utilize a number of strategies to evade an effective immune response (Fig. 12.5). The basis of an effective immune response against any Ag is its recognition as a nonself and its presentation to immune effector cells. Tumors escape recognition by either presenting self Ags to which the immune system is already tolerized or by modulating their antigenicity. The later involves the shedding of tumor Ags into the circulation from where they may be removed [30]. The next line of defense adopted by tumor cells is the modulation of APCs, rendering them incapable of effectively presenting cancer Ags to immune cells. The APCs like DCs are either deleted or functionally compromised in response to the factors secreted by malignant cells [31]. Tumor-induced co-inhibition of the second signal of the Ag presentation and consequent immunosuppression has now been recognized in several cancer types [32]. In addition, the tumors alter MHC molecules especially MHC class I and other components of Ag processing machinery in the APCs, so as to further incapacitate the presentation of its Ags to the immune system [33]. Besides, tumor cells plunge into an active battle against the immune process by attacking its adoptive and innate immune cells. Tumor cells subvert T cells and render them anergic through co-inhibitory molecules including cytotoxic T-lymphocyte antigen-4 (CTLA-4) and PD-L1 [34]. Anergic T cells are unable to produce cytokines such as IL-2 and IFN-γ. Therefore, the autocrine and paracrine activation of CD4+ cells and other immune cells including B cells, macrophages, and CD8+ cells are blocked, leading to further suppression of the immune cascade [35]. Moreover, tumors also express Fas ligands on T cells, leading to lymphocyte apoptosis [36]. Not only do they suppress CD4+ and CD8+ cells, but also promote the suppressor T-cell phenotype such as CD25+Foxp3+ T-regulatory cells. These cells secrete IL-10, TGF-β, and VEGF which suppress the antitumor response and promote tumoral angiogenesis (Table 12.2) [37]. Besides, tumors also inhibit innate immune response by induction of quantitative and qualitative defects in NK cells, macrophages, and neutrophils. NK cells have been found to exhibit decreased cytotoxic potentiality due to the presence of tumor-secreted factors including TGF-β in the tumor microenvironment (TME) [38]. The later along with other cytokines (IL-4, IL-13, etc.) present in the tumor bed favors the accumulation of M2 macrophages, which also induce immunosuppression [39]. Recruitment of immature myeloid cells like myeloid-derived suppressor cells (MDSCs) further complements the tumor-immunodeficient environment by reducing T-cell and NK-cell activation and promoting neovascularization via factors like VEGF [40].
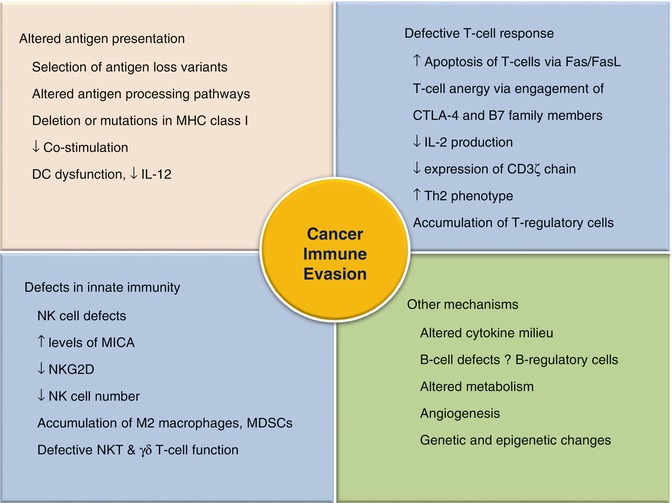
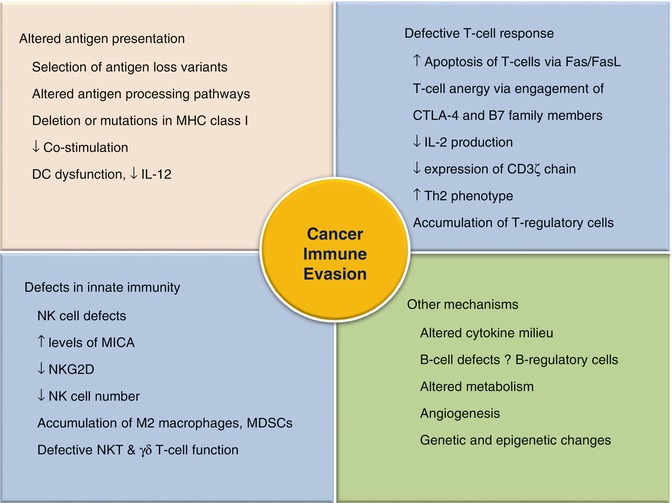
Fig. 12.5
Mechanisms of immune evasion by the cancer
Table 12.2
Mechanisms of immunosuppression induced by T-regulatory cells and myeloid-derived suppressor cells
T-regulatory cells |
Secretion of immunosuppressive molecules like IL-10, IL-35, and TGFβ |
Polarization of DCs toward tolerogenic phenotypes |
Direct cytolysis of effector T cells via granzyme B, TRAIL, and galectin-1 |
Metabolic changes like increased IDO in DCs and increased conversion of ATP to adenosine promoting immunosuppression |
Stimulation of tumoral angiogenesis via VEGF secretion |
Myeloid-derived suppressor cells |
Inhibition of effector T-cell proliferation and function via L-arginine-dependent mechanisms |
T-cell inhibition via production of ROS and TGFβ |
Reduced T-cell homing via depletion of L-selectin |
Promotion of Th2 and T-regulatory phenotypes via IL-10 secretion |
Inhibition of DC function via IL-10 |
Promotion of angiogenesis via secretion of VEGF, basic fibroblast growth factor, HIF-1, etc. |
Other mechanisms such as anaerobic glycolysis, hypoxia, and acidity of the TME along with the existent defects in tryptophan metabolism induced by increased expression of the enzyme indoleamine 2,3-dioxygenase (IDO) further depress the antitumor immunity, thereby leading to cancer progression and metastasis [41–43].
12.3 Tumor Antigens and Cancer Immunoediting
Antigenicity of tumors has always been a matter of discussion. In the past, it was believed that since tumors are derived from self cells, the immune system is more receptive to their Ags. However, it was subsequently noticed that tumors may express Ags which are quantitatively or qualitatively different from self Ags, thus rendering them sensitive to the immune attack. Quantitative differences include significantly increased expression of Ags, which are less expressed in normal or benign conditions or reexpression of Ags only expressed at a specific stage of embryonic development (Table 12.3). Moreover, the lineage-specific Ags expressed normally in specific tissues may be expressed aberrantly in tumor cells. Qualitative differences are produced due to mutational events occurring during carcinogenesis. Over the years, several efforts have been made for the identification and mapping of the Ags expressed on tumor cells; various nomenclatures have been used to characterize them such as tumor-associated Ags and tumor-specific Ags. Antigens capable of evoking a tumor-specific immune response have also been designated as tumor rejection Ags in some textbooks, e.g., tyrosinase, MUC-1, Her-2/neu, β-catenin, caspase-8, etc. [44]. Previous studies on tumor antigens (TAs) have mainly focused on the discovery of new Ags and their classification into two subclasses, a group which can evoke a protective immune response and another group serving as potential therapeutic targets. However, the advent of cancer immunoediting theory has changed our insight on TAs, as they are now considered to be one of the prime targets of the above process. Currently, ongoing studies are attempting to differentiate between the antigenicity of the original or unedited tumors and those sculpted by the immune system [17,45,46]. Differences between the immunogenicity of tumors derived from carcinogen MCA (more immunogenic) and those arising spontaneously (less immunogenic) in mice have been described by DuPage et al. [47]. They also showed that primary sarcomas are edited by the immune system and, hence, become less immunogenic in order to escape the T-cell response. In the same line, Matsushita et al. obtained similar results in their study on tumor exomes [48]. A recent study has revealed the presence of antiinflammatory antibodies to tumor-associated Ags like NY-ESO-1, thereby suggesting the importance of humoral immune system in cancer immunoediting [49]. Novel genetic-based approaches including exome sequencing, in silico analysis, and CD8+ T-cell cloning are likely to further help in understanding the alterations in tumor antigenicity occurring during different phases of cancer immunity [48].
Antigen type | Antigen class | Antigen | Characteristics of antigens | Tumor |
---|---|---|---|---|
Tumor-associated antigens | Oncofetal antigens | CEA | Expressed in fetal tissues, reexpressed in tumors | Colon cancer |
AFP | Germ cell tumors, HCC
![]() Stay updated, free articles. Join our Telegram channel![]() Full access? Get Clinical Tree![]() ![]() ![]() |