Fig. 10.1
GR9 fibrosarcoma tumor model. Cell clones are adapted to tissue culture from the primary tumor and classified according to MHC-I surface expression
The intratumoral heterogeneity in H-2 class I expression presented in GR9 cell lines is not an unusual case since other MCA-induced tumors obtained in our laboratory (GRB7.1, GRB7.2, and GRIR5) presented similar levels of H-2 class I heterogeneity. These differences have a strong influence on in vivo tumor behavior in immunocompetent mice [13]. Local tumor growth of different clones of GR9 in syngeneic immunocompetent BALB/c mice showed an inverse correlation between the MHC-I phenotype of tumor clones and their local tumorigenic capacity [59, 61]. Comparing local tumor growth after subcutaneous injection of 6.25 × 105 cells of A7, B7, C5, and B11, we found that all cell lines grew in vivo locally. A7 and B7 showed similar growth rate, but different from C5 and B11. Thus, local tumors of mice injected with C5 and B11 cell clones began to grow at day 8 and were removed at days 23 and 28, respectively. In contrast, the other two clones, A7 and B7 cells, began to grow later at days 14 and 16 postinjection, respectively; the primary tumor was removed at day 39. Clones with high MHC-I expression are very immunogenic in local tumor growth experiments; in contrast, clones with decreased MHC-I expression grew rapidly in vivo when injected subcutaneously. The behavior is totally opposite in spontaneous metastatic capacity (see following section). In brief, results clearly show that in this tumor model, an inverse correlation between MHC-I surface expression on tumor clones and local tumorigenic capacity exists. Moreover, these differences in local tumor growth were associated with an immune response, since the clones progressed similarly in irradiated syngeneic BALB/c mice [61].
10.3 MHC-I Expression and Metastatic Progression
Metastatic progression is a complex process during which cancer cells leave the heterogeneous primary tumor to spread to secondary sites. Thus, pathogenesis of cancer metastases involves a set of sequential events initiated when tumor cells acquire an invasive phenotype [62–64]. These invasive tumor cells detach from matrix, invade the tissue, and migrate toward blood or lymphatic vessels to finally get access to the systemic circulation. However, most tumor cells are destroyed after extravasation into circulation by the immune system or hemodynamic forces, and only a small proportion eventually extravasate and arrive at the new site [65, 66]. This last step requires complex interactions between tumor cells and distant tissue microenvironment [67, 68]. Some in vitro model systems have contributed to the study of individual steps of metastatic cascade [69, 70]. However, the major limitation of these models is that they do not incorporate the complex interplay between host and tumor cells; therefore, it is necessary to work with in vivo models. One of the most common problems about cancer research and treatment is difficulty reproducing metastatic human disease using in vivo models. Preclinical tumor models must mimic the fundamental steps associated with the metastatic cascade [71, 72]. Three main types of models in vivo have been employed to approximate the situation observed in patients with advanced metastatic disease: genetically engineered mouse models (GEMM), transplantable tumor model systems (GRAFT) or spontaneous metastasis assays, and experimental metastasis assays. At first, an oncogenic alteration is introduced (deletion or overexpression) in a specific tissue [63, 73–75]. The other alternative extensively used, GRAFTs, recapitulate all steps of secondary colonization by spontaneous visceral metastasis. In these models, tumors or tumor cell lines are transplanted into mouse, generating a primary tumor that will be excised to prolong survival of hosts, thus increasing the possibility of distant spontaneous metastases [76–79]. Experimental metastasis assay also is the other common test to investigate biological behavior of tumor cells in vivo. In experimental metastasis assays, tumor cells are directly injected into blood circulation to spread to organs. We considered that spontaneous metastases assay resembles all sequential steps associated with the metastatic cascade, from primary local tumor to secondary colonization. In contrast, experimental metastasis assay is a bypass in the metastatic cascade, evading the first steps: local primary tumor growth, migration, and extravasation into blood and/or lymphatic vessels. Our research group has compared the behavior of different tumor cell lines in experimental and spontaneous metastases assays, finding that it is opposite. Tumor cell lines with high spontaneous metastatic ability showed very low experimental metastatic capacity [59]. In consequence, we think that experimental metastasis assays should not be used as a model for studying metastatic advance disease.
10.3.1 MHC Class I Expression on Primary Tumor Cells May Determine Spontaneous Metastatic Capacity
During the late 1970s, heterogeneity in metastatic potential of tumor populations was demonstrated by Fidler and Kripke, using a mouse malignant melanoma [80]. Great difference between the ability of clones from B16 cell line was observed in terms of developing metastatic colonies in vivo. This fact suggests that a heterogeneous population composed the primary tumor where there were nonmetastatic and metastatic tumor cells. Later research on various cell lines including clones with different metastatic potentials isolated in tumor cell populations of BALB/cfC3H mammary adenocarcinoma [81], methylcholanthrene [82], or ultraviolet-light-induced fibrosarcomas [83, 84] supported these findings. However, Haywood and McKhann were the first to suggest the possible influence of the MHC-I genes on metastatic capacities of tumor cell populations [85]. They compared metastatic capacity of five methylcholanthrene-induced sarcomas, finding that tumors more metastatic had quantitatively more H-2 surface expression. These results, as well as later evidences observed by other groups, showed that the level of MHC-I expression was implicated in the metastatic capacity of the tumor cells. Three different spontaneous tumors originated in mouse, Lewis lung carcinoma (3LL), B16 melanoma, and BW T lymphoma, have been used by Eisenbach’s research group to show whether metastasis disease is influenced by MHC-associated mechanisms. They worked with different tumor cell variants of these tumors, finding that metastatic ability directly correlated with surface expression levels of the H-2 D Ags and inversely with the of H-2 K Ags [86–89]. Moreover, H-2 K-negative/D-positive clones with high metastatic ability reverted their metastatic phenotype, inducing H-2 K-restricted CTLs when transfected with the H-2 K gene [87, 90, 91, 92]. In brief, these results support that the metastatic phenotype is associated with H-2 D surface expression and loss of H-2 K surface expression in primary tumor cells. In this context, Kazav et al. using T10 sarcoma (H-2 b × H-2 k) [induced by methylcholanthrene in a (C57BL/6J X C3HeB/-FeJ) mouse] reported that expression of MHC-I increased the metastatic capacity of tumor cells [93, 94]. Several clones of T10 sarcoma presented differential expression of H-2b and H-2k haplotypes: H-2b x H-2k positive and only H-2b positive. Metastatic clones characterized to express both parental haplotypes and nonmetastatic clones only showed expression of H-2b haplotype [95]. Furthermore, metastatic potential in this tumor system was only acquired when H-2 Dk-Ags were expressed on the surface of tumor clones. Moreover, T10 clones expressing only H-2 Dk-Ags were more metastatic than clones expressing both H-2 Db and H-2 Dk-Ags, while clones merely expressing H-2 Db Ag were nonmetastatic [95, 96].
10.3.2 Different MHC-I Surface Expression on GR9 Tumor Clones Determines Their Spontaneous Metastatic Capacity
In our laboratory, the GR9 fibrosarcoma murine model was used to assess whether levels of MHC-I surface expression on primary tumor cells exert influence on their spontaneous metastatic capacity. Four cell clones (A7, B7, C5, and B11) with different MHC-I surface expressions were chosen for spontaneous metastasis assays (Fig. 10.1). Results showed significant differences in metastatic capacity between these clones [59]. For example, A7 clone with a strong H-2 class I surface expression was highly metastatic, generating metastases in 90 % of the hosts and resulting in 1–50 metastases per animal. Clones with intermediate or low H-2 class I expression, as B7 or C5, presented lower metastatic capacity, 50 and 20 %, respectively. In contrast, MHC-I-negative B11 clone did not present spontaneous metastatic capacity, and the B11 tumor-bearing mice remained free of metastasis at the end of the assays for more than 24 months. In brief, cell clones with high surface expression of H-2 class I molecules were also highly metastatic, but those clones with low or negative H-2 class I expression were weekly or nonmetastatic (Fig. 10.2). Our experimental evidences support the idea that levels of MHC-I surface expression of primary tumor cells directly correlated with spontaneous metastasis ability and inversely with local oncogenicity, as it was shown above [59] (Fig. 10.2). Consequently, extrapolation of oncogenic and metastatic behavior of tumor cells in vivo is not always possible, because they may be completely opposite.
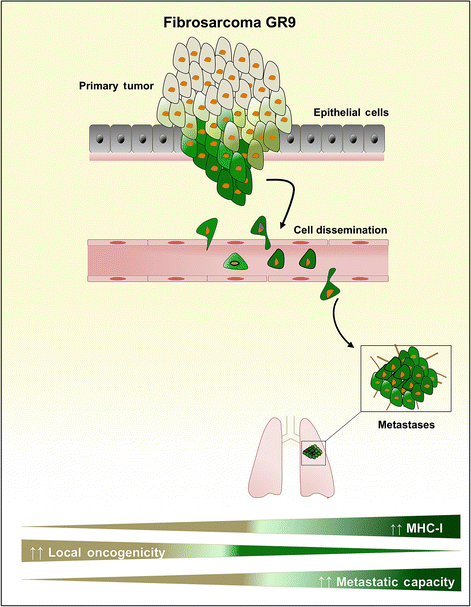
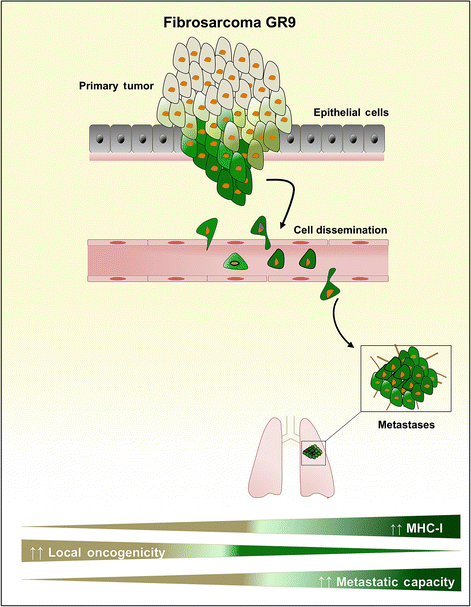
Fig. 10.2
Schematic representation of the dissemination and invasion of GR9 primary tumor cells. MHC-I-positive tumor cells from GR9 primary tumor presented a high spontaneous metastatic capacity, whereas MHC-I-negative tumor cells presented a weak spontaneous metastatic capacity
Analysis of MHC-I cell surface expression on spontaneous metastases derived from these fibrosarcoma clones displayed that in all cases the metastases presented the same or lower MHC-I surface expression than the original clone [59]. In consequence, metastatic progression promoted a downregulation in MHC-I surface expression. Analysis of leukocyte subpopulations in tumor-bearing mice revealed a distinct behavior among different clones. A7 and B7 produced immunosuppression characterized by decrease in T lymphocytes and increase in Treg cells [29]. In contrast, B11 tumor-bearing mice developed a strong immunostimulation characterized by an increase in T lymphocytes, dendritic, and macrophages cells (unpublished observations). In brief, A7 and B7 cells progressed to metastatic disease suppressing the immune response, whereas that B11 clone promoted an immune response which avoided metastatic progression. The other GR9 tumor clone studied was B9, with H-2-negative surface expression and with weak spontaneous metastatic capacity (0–1 metastasis per mouse). In contrast, this clone is highly metastatic using nu/nu BALB/c mice, ranging 5–7 per mouse [28, 97]. Moreover, metastases were H-2 class I negative in immunocompetent hosts and H-2 positive in immunodeficient hosts. Thus, we observed that H-2 phenotype of spontaneous metastases was influenced by immunological state of the hosts.
GR9 fibrosarcoma cell line, composed of different cell clones, presented intermediate levels of H-2 Kd, H-2 Dd, and H-2 Ld molecules. Analysis of spontaneous metastases assay with GR9 tumor cells revealed that GR9 cells have high spontaneous metastatic capacity; 90 % of tumor-bearing mice develop metastases, ranging 1–9 per animal. GR9 produced strong immunosuppression in tumor-bearing mice. Interestingly, 96 % of metastases derived from GR9 clone showed downregulation of MHC-I surface expression. These results suggest that MHC-I-positive clones, as A7 or B7, produced immunosuppression, favoring the growth of MHC-I low or negative clones.
Other experimental evidences from our tumor model also support the idea that in GR9 fibrosarcoma tumor, the amount of MHC-I Ags also affects NK cell cytotoxicity [98]. Since NK cells have been recognized as one of the main host immunological mechanisms against metastasis disease, this notion seems imperative [99]. In our system, tumor clones with no or low expression of MHC-I molecules were found to be sensitive to NK mediated lysis, while clones with high levels of MHC-I expression were relatively resistant [98].
10.4 Immunotherapy as a Treatment Against Cancers Expressing Different MHC-I Surface Expression
10.4.1 Immunotherapy as a Treatment Against Primary Tumors with Different Levels of MHC-I Expression
As mentioned above, MHC-I molecules present TAA to CTLs; therefore, MHC-I surface expression on tumor cells may play an important role in the outcome of immunotherapies as anticancer treatments. During treatment with vaccines containing peptides derived from TAAs, MHC-I-positive surface expression on tumor cells presenting these TAAs is crucial to make this immunotherapy effective. As a consequence, before the application of immunotherapies, MHC-I surface expression on tumor cells must be analyzed. Furthermore, two immunosuppressive mechanisms have been described recently showing evasion of tumor cells from CTLs attack, mediated by expression of noncognate MHC-I molecules or by myeloid-derived suppressor cells (MDSCs) [100, 101].
Several murine tumor models have been used to evaluate the application of different immunotherapies to recover MHC-I surface expression in MHC-I-deficient tumor cells, in order to promote an antitumor immune response. In MHC-I-negative B16 melanoma cells, intratumoral electroporation of IL-12 cDNA promoted an increase in their MHC-I surface expression, mediated by IFN-γ, leading to the eradication of established melanomas by activation of CTLs [102]. In cervical carcinoma cells, administration of synthetic oligodeoxynucleotide-bearing CpG motifs (CpG-ODNs) upregulated MHC-I surface expression causing tumor regression mediated by CTLs [103]. Other studies also have reported that CpG-ODNs immunotherapies delayed the growth or inhibited minimal residual tumor disease of both MHC-deficient and MHC-positive tumors [104, 105]. Moreover, combination of dendritic cell-based vaccines with CpG generated inhibition of tumor growth in MHC-positive and MHC-negative tumors [106]. CpG-ODN 1585 only produced regression of MHC-deficient tumors, principally activating NK cells [105]. In other assays, depletion of T(reg) cells avoided the growth of recurrent tumors after surgery of MHC-negative and MHC-positive tumors [107]. In all these assays, the action against MHC-I-deficient tumors was mediated by NK or NK1.1+ cells [108]. Previous to the application of immunotherapy, MHC-I-deficient tumor cells may be treated with agents to upregulate MHC-I surface expression. Epigenetic mechanisms are frequently implicated in MHC-I downregulation of tumor cells; as a result, application of agents as 5-azacytidine (5AC) or trichostatin A could increase MHC-I surface expression [109, 110]. Treatment of 5AC with CpG-ODN or with IL-12 showed additive effect against MHC-I-deficient tumors, being the immune response mediated by CD8+ T cells [111]. Other chemo-immunotherapies, based on ifosfamide derivative CBM-4A together with IL-12, also led to significant inhibition in the growth of MHC-I-deficient tumors [112].
10.4.2 Immunotherapy as a Treatment Against Metastatic Progression Derived from Primary Tumors with Different MHC-I Expression
Immunotherapy has also been used as an antimetastatic treatment against spontaneous metastasis derived from primary tumors with different MHC-I expressions. As mentioned above, studies performed by Eisenbach’s et al. showed an inverse correlation between H-2 K tumor cell surface expression and spontaneous metastatic capacity [86, 89, 90, 113]. Tumor cell lines derived from H-2 K-low or H-2 K-deficient primary tumors presented high spontaneous metastatic capacity, which was reverted by transfection of tumor cells with H-2 K gene [86, 114, 115]. Moreover, injection of the H-2 K-transfected tumor cells that protect against metastatic disease originated from H-2 K-low or H-2 K-deficient tumors. Furthermore, therapy with IFN-γ-treated tumor cells or with H-2 K-transfected tumor cells promoted upregulation of H-2 K surface expression and protected against metastatic dissemination from parental tumor cells [113, 115]. An additional effect was reached when tumor cells were jointly transfected with IFN-γ and allogeneic MHC class I genes [116].
In GR9 murine tumor model, the influence of MHC-I cell surface expression on primary tumors has been investigated with respect to the success of immunotherapy as antimetastatic treatment. A7 is a fibrosarcoma clone with strong spontaneous metastatic capacity. Four treatments were used: two immunotherapies (CpG + irradiated autologous A7 cells, and PSK) [117], one chemotherapy (docetaxel), and one chemo-immunotherapy (PSK + docetaxel). A7 tumor clone was injected subcutaneously in BALB/c mice, and the primary tumor was excised when the large tumor diameter reached 10 mm. Treatment began 1 week after tumor removal, on a weekly basis during 6 weeks; 1 week after the last dose, mice were euthanized and autopsy was performed. Interestingly, all mice treated with each immunotherapy or chemo-immunotherapy appeared metastases-free (Fig. 10.3) [29]. In contrast, partial reduction in the number of metastases occurred in the mice treated with chemotherapy. In the control group, mice injected with A7 tumor cells and treated with saline solution, a high number of spontaneous metastases in all mice were observed (Fig. 10.3) [29]. In brief, the two immunotherapy protocols and the one chemo-immunotherapy protocol eradicated metastasis completely and cured the mice, whereas chemotherapy treatment reduced the number of metastases partially. When the same four treatment protocols were applied against spontaneous metastases generated from B7 fibrosarcoma clone (intermediate MHC-I expression level and with lower spontaneous metastatic capacity than A7 clone), the antimetastatic effect was not as effective (Fig. 10.3). PSK, PSK + docetaxel, and docetaxel promoted partial reduction in the number of metastases, whereas that CpG + irradiated autologous B7 cells treatment did not produce any antimetastatic effect (unpublished data). In the case of spontaneous metastases derived from GR9 fibrosarcoma, neither treatment had any antimetastatic effect. Analysis of lymphocyte subpopulations in different assays showed that growth of local tumors promotes strong immunosuppression in the three cases. However, this immunosuppression was completely reverted by immunotherapies in the case of A7-injected mice, partially reverted for B7-injected mice, and remained unchanged in GR9-injected mice [29]. All these results suggest that immunotherapies may be potential antimetastatic treatments against primary tumors with high MHC-I cell surface expression.
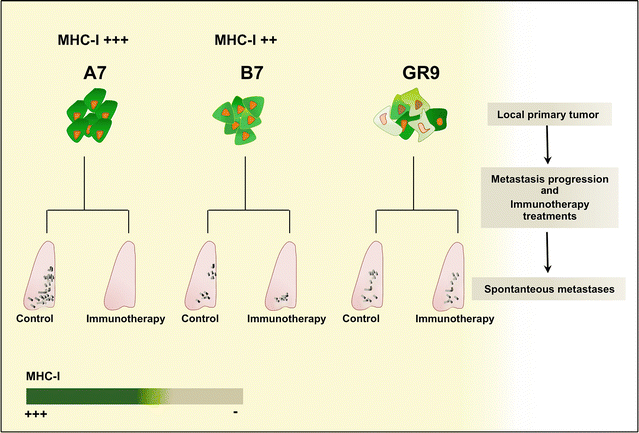
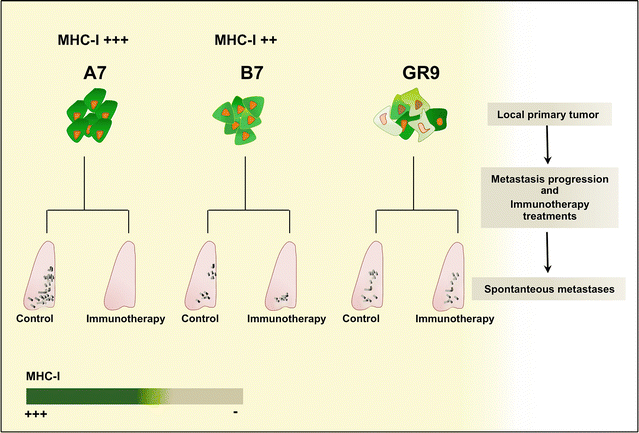
Fig. 10.3
Immunotherapy as an antimetastatic treatment against tumors with different MHC-I expression. Immunotherapy was completely effective in inhibiting spontaneous metastatic progression in A7 tumor clone (MHC-I highly positive). For B7 tumor clone (intermediate level of MHC-I expression), immunotherapy accomplished partial reduction in the number of spontaneous metastases. In the case of GR9 fibrosarcoma, immunotherapy had no antimetastatic effect
10.5 Concluding Remarks
In tumor cells, MHC-I molecules may present peptides derived from tumor-associated antigens, which are new proteins expressed or overexpressed in tumor cells. Presentation of these new peptides may allow recognition and destruction of tumor cells by CD8+ T lymphocytes. Loss of MHC-I expression on tumor cells is a widespread and frequent mechanism developed to escape from immunosurveillance. Alteration in MHC-I in both human and murine experimental tumors has been widely reported. Results show an inverse correlation between MHC-I expression on tumor cells and primary tumor growth, i.e., MHC-I-negative tumors grew more rapidly compared to MHC-I-positive tumors. In contrast, a direct correlation was found between MHC-I expression on primary tumors and spontaneous metastatic capacity. Immunotherapy as an antimetastatic treatment was completely effective against MHC-I highly positive tumors and was partially effective on tumors with intermediate level of MHC-I expression.
Acknowledgements
The authors would like to thank I. Linares, A.B. Rodriguez, and E. Arias for technical advice. This study was supported by grants from the ISCIII-FEDER (CP03/0111, PI12/02031, PI 08/1265; PI 11/01022, RETIC RD 06/020), Junta de Andalucía (Group CTS-143 and CTS-695, CTS-3952, CVI-4740 grants), and European Community (LSHC-CT-2004-503306, OJ 2004/c158, 18234). A.M.G.L. was supported by Miguel Servet Contract CP03/0111 and Contract I3 from ISCIII and FPS, I.R. by Rio-Hortega contract CM12/00033 from ISCIII.
References
1.
Gorer PA. The significance of studies with transplanted tumours. Br J Cancer. 1948;2(2):103–7.PubMedCentralPubMed
2.
Dausset J. The agglutination mechanism of trypsin modified red cells. Blood. 1952;7(8):816–25.PubMed
3.
Bjorkman PJ, Saper MA, Samraoui B, Bennett WS, Strominger JL, Wiley DC. Structure of the human class I histocompatibility antigen, HLA-A2. Nature. 1987;329(6139):506–12.PubMed
4.
Bjorkman PJ, Parham P. Structure, function, and diversity of class I major histocompatibility complex molecules. Annu Rev Biochem. 1990;59:253–88.PubMed
5.
Le Bouteiller P. HLA class I chromosomal region, genes, and products: facts and questions. Crit Rev Immunol. 1994;14(2):89–129.PubMed
6.
Parham P, Ohta T. Population biology of antigen presentation by MHC class I molecules. Science. 1996;272(5258):67–74.PubMed
7.
Grandea 3rd AG, Van Kaer L. Tapasin: an ER chaperone that controls MHC class I assembly with peptide. Trends Immunol. 2001;22(4):194–9.PubMed
8.
Maffei A, Papadopoulos K, Harris PE. MHC class I antigen processing pathways. Hum Immunol. 1997;54(2):91–103.PubMed
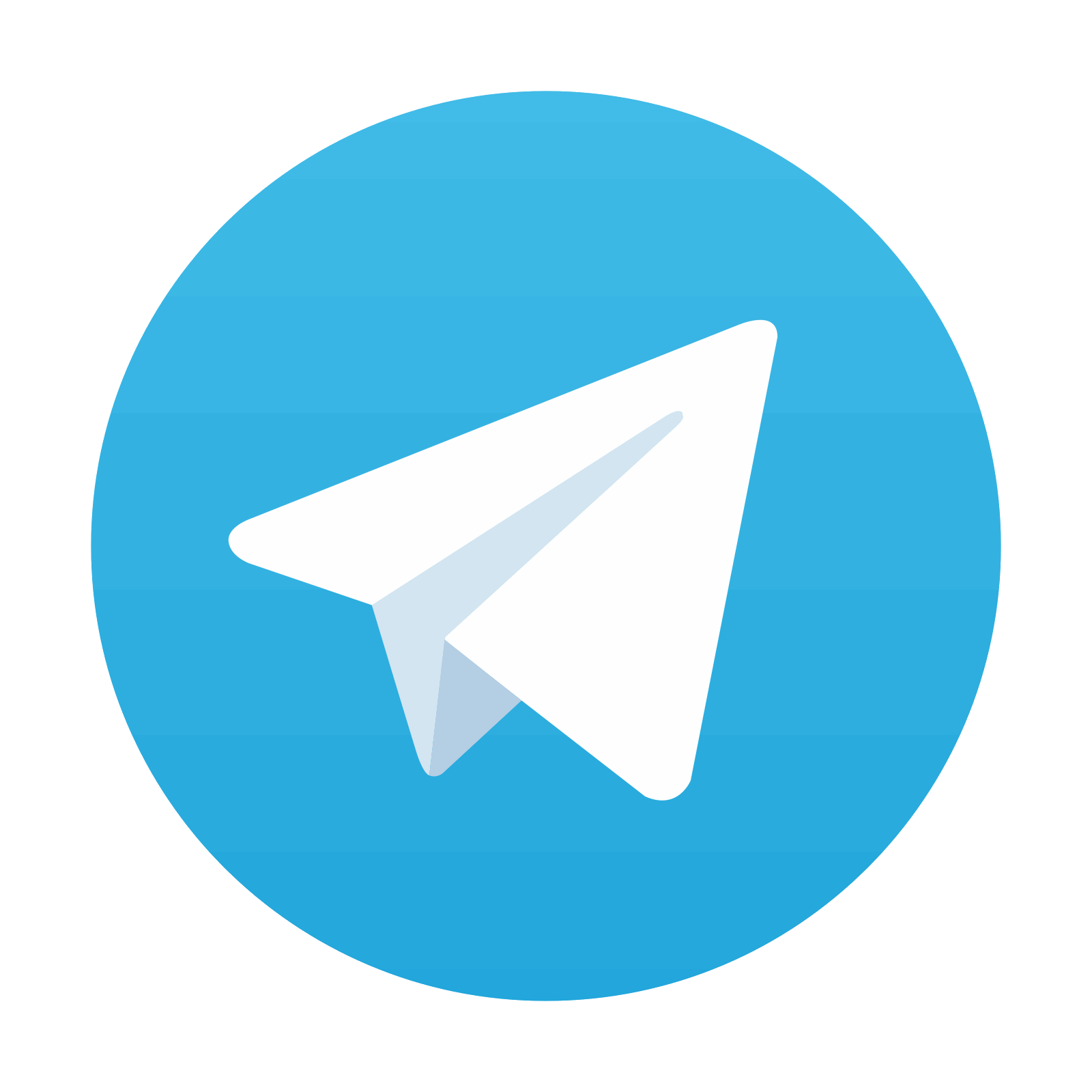
Stay updated, free articles. Join our Telegram channel

Full access? Get Clinical Tree
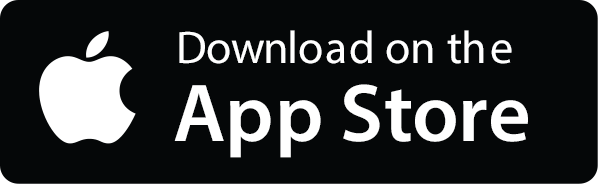
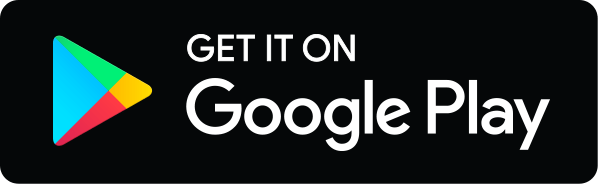