Environmental Exposure
Exposure to ionizing radiation is the strongest single factor linked to an increased risk of multiple myeloma.16 This has been documented in atomic bomb survivors who have a five times greater incidence than a control group with a latent period of approximately 20 years from exposure.17 People exposed to low levels of radiation also demonstrate an increased incidence of myeloma, including radiologists, employees in the nuclear industry, or those handling radioactive materials. An association between exposure to various chemicals and the risk of multiple myeloma remains ill defined. Exposure to metals, especially nickel; agricultural chemicals; benzene and petroleum products; other aromatic hydrocarbons; agent orange; and silicon have been considered as potential risk factors.16,18–20 In contrast, alcohol and tobacco consumption has not been clearly linked to myeloma. Among medications, only mineral oil used as a laxative has been reported to be associated with an increased risk of multiple myeloma in some patients.21,22
Hereditary and genetic factors may predispose patients to myeloma development.23,24 Among 37 families with at least two family members who had myeloma, occurrence among siblings was reported in 25 of the families. However, direct genetic linkage has not been established. Myeloma risk also appears to be enhanced by the presence of HLA-Cw2 in both African American and Caucasian populations. In a study in 917 Ghanaian men, the prevalence of MGUS was twice that in Caucasian men, implicating race-related genetic susceptibility in the higher rates of MGUS in African populations.14 More recently, high throughput genomic studies have identified various novel genomic changes in myeloma with significant clinical implications. A meta-analysis of two genome-wide association studies in myeloma (N = 1,661) showed that the t(11;14)(q13;q32) translocation, in which CCND1 is placed under the control of the immunoglobulin heavy chain enhancer, was strongly associated with the CCND1 c.870G>A polymorphism (p = 7.96 × 10[−11]), suggesting a model where a specific chromosomal translocation is associated with a constitutive genetic factor.25 Recently, Genome Wide Association Studies (GWAS) have identified common single nucleotide polymorphisms (SNP) at 2p23.3(rs6746082), 3p22.1(rs1052501), 3q26.2(rs10936599), 6p21.33(rs2285803), 7p15.3(rs4487645), 17p11.2(rs4273077), and 22q13.1(rs877529) to influence MM risk; the same SNPs were also evaluated for MGUS risk and four of these SNPs (rs1052501, rs2285803, rs4487645, and rs4273077) independently also influenced the development of MGUS. Collectively, these data support the view that genetic variation predisposes one to MGUS, thereby influencing the risk of developing MM.26
MGUS has been considered to be a premalignant condition; however, the rate of conversion to myeloma remains extremely low and is often associated with additional genetic changes.27,28 Repeated infections or antigenic stimulation of the plasma cell compartment has also been proposed as a possible predisposing condition for developing myeloma. In one interesting patient report in the literature, a prior therapy with horse antiserum against tetanus led to a subsequent development of MGUS that lasted for 3 decades before conversion to MM. At the time of myeloma diagnosis, the serum IgG component was found to react specifically against horse α-2 macroglobulin.29 This report suggests an initial antigen-driven stimulation of monoclonal protein-producing plasma cells, which eventually became malignant after acquiring additional genetic alterations. MGUS has been observed in mice dependent on strain of mice, age, preexisting immune status, and antigenic stimulation. MGUS has been associated with immune disorders and infectious diseases. In one report of 57 patients with MGUS who had undergone an evaluation for Helicobacter pylori infection for various gastrointestinal (GI) symptoms, 39 (68%) had evidence of H. pylori infection and 11 of these 39 patients (28%) had normalization of the serum paraproteins following eradication of this infection.30 Seroprevalence of H. pylori, however, has not been consistently correlated with MGUS.31 Development of MGUS has also been reported with T-cell deficiency disorders as in AIDS.32 Importantly, a recent study indicates that the diagnosis of symptomatic MM is always preceded by MGUS by 2 or more years.33
Although epidemiologic studies have not been able to conclusively establish an association between MM and infectious or autoimmune diseases, a recent retrospective cohort study in US veterans demonstrated significantly elevated risks of MM in patients with a history of autoimmune, infectious, and inflammatory disorders. Risks for MGUS were generally of similar magnitude. These results indicate that various types of immune-mediated conditions might act as triggers for MM/MGUS development.34 Although an initial report suggested the presence of the human herpes virus 8 (HHV8; Kaposi’s sarcoma herpes virus) in the bone marrow dendritic cells of the majority of patients with MM,35 analogous to its association with other lymphoproliferative diseases such as Castleman’s disease,36 body-cavity lymphoma,37 and Kaposi’s sarcoma,38 other investigators have failed to identify HHV8 in myeloma cells or dendritic cells from various sources including mobilized peripheral blood stem cells.39–42 Because HHV8 produces unique gene products, including possible growth promoting factors for myeloma such as analogs of interleukin 6 (IL-6), insulin-like growth factor 1 (IGF-1), and an IL-8, the possible linkage of HHV8 to myeloma was intriguing. However, even antibodies against HHV8 have not been observed in MM.43
MM is a germinal center–derived tumor with a mainly postswitch B-cell phenotype characterized by extensive Ig gene hypermutation in a pattern suggesting antigen selection. This is reflected in the exceedingly rare occurrence of IgM myeloma. Somatic mutations of other loci, such as B-cell lymphoma 6 (BCL-6), have also been reported in myeloma B cells, along with characteristic immunoglobulin gene rearrangement.44 Similar mechanisms may be affecting other cell-cycle control genes whose products regulate cell proliferation and malignant transformation.
As in most malignancies, the pathogenesis of MM appears to be associated with dysregulated expression and function of multiple key cellular genes controlling apoptosis, cell growth, and proliferation. Understanding the evolution of myeloma from MGUS has provided a background for a multistep process involving alterations in various oncogenes and tumor suppressor genes.45 In one study, the presence of 14q32 abnormalities were reported in patients with MGUS, and the addition of chromosome 13 change was associated with a transformation to overt MM.46 This led to a theory that a subset of myeloma may derive from prior MGUS with a high incidence of monosomy 13, versus a second group of de novo myeloma in which other genetic abnormalities may be involved.46 However, two recent reports suggest that all myelomas are preceded by MGUS33,47 and that a small subset of MGUS carry del(13) along with t(4;14), which is not associated with an increased rate of progression to myeloma.48,49 In one study in patients with smoldering myeloma, high-risk chromosomal aberrations (del(17p13), t(4;14), and +1q21) were observed in 35.9% of patients and predicted adverse prognostic factors for the progression to active myeloma, independent of tumor mass. Moreover, hyperdiploidy, which in myeloma is considered to be a low-risk feature, was present in 43.3% of smoldering and indolent multiple myeloma (SMM) patients and was an adverse prognostic factor.50
Myeloma occurs not only in humans, but also in mice, canines, and hamsters. In fact, genetic susceptibility to plasma cell tumors has been demonstrated in an inbred strain of mice. A common factor in various species has been the prevalence of endogenous retroviruses.51,52 Animal models are now providing a basis for understanding the role of activation of oncogenes and tumor suppressor genes, cytokines, and the role of the bone marrow microenvironment in promoting and sustaining myeloma cell growth.
Cytogenetic and Molecular Genetic Alterations
Myeloma karyotypes are complex, with an average of 11 numeric and structural abnormalities per cell.53 The relative incidence of gain or loss of various chromosomes with involved p and q arms are shown in Figure 112.2. The low proliferative activity of the tumor cells and possible clonal evolution have been obstacles to the identification of specific chromosomal and molecular changes in myeloma. The frequency and complexity of the chromosomal aberrations increases with advanced disease, and is uniformly abnormal in plasma cell leukemia. The detection of a complex karyotype predicts for poor prognosis. The newer techniques of multicolor fluorescent in situ hybridization (FISH) and spectral karyotyping, along with refined G-banding techniques, have identified many nonrandom changes in a large number of patients.54–56 Using these techniques, over 90% of patients have involvement of at least one chromosome. A substantial fraction of MGUS plasma cells are also reported to have aneuploidy. By FISH analysis, the incidence of trisomy for at least one chromosome was reported in over 40% of MGUS cells.57 The characteristic numerical abnormalities are monosomy 13 and trisomies of chromosome 3, 5, 7, 9, 11, 15, and 19.
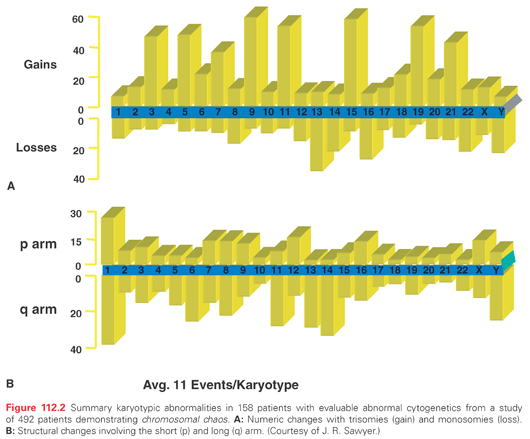
The most frequent structural abnormality involves chromosome 1 and the immunoglobulin heavy chain gene at 14q32. By conventional cytogenetics, the 14q32 region is involved in translocation in 20% to 40% of cases, and by molecular and FISH techniques it is detectable at higher frequency, ranging from 50% in MGUS to 90% in advanced myeloma.53,58,59 The demonstration of this abnormality in MGUS suggests its involvement in the initial step of transformation.46,60,61 Light-chain translocations involving Igλ (22q11) or Igκ (2p12) are less commonly observed, with only 20% of cases even in advanced MM. The most common translocation involving 14q32 results in the overexpression of cyclins D1 (on 11q13) and D3 (on 6p21).58,62 The other biologically important partner chromosomes are 4p16 (fibroblast growth factor receptor 3 [FGFR3] and multiple myeloma SET domain [MMSET]), 16q23 (c-MAF), and 20q11 (MAFB) (Table 112.1). Other recurrent partner loci less frequently identified include 8q24 (c-myc) in less than 5% cases, 18q21 (bcl-2), (11q23 mixed lineage leukemia 1 [MLL-1]), and 6p21.1. The 4p16 region contains FGFR3 and MMSET genes. FGFR3 and its activating mutations trigger mitogen-activated protein (MAP) kinase signaling and growth of myeloma cells.63 Mutated FGFR3 also confers resistance to caspase 3–related apoptosis.64–66 This translocation also activates the MMSET gene, a homolog of MLL1, which is also independently involved in 11q23 translocation. With the enhanced sensitivity of spectral karyotyping, a nonrandom involvement of t(14:16) (q32:q22-23) has been described. A molecular analysis of the locus at chromosome 16q22 shows fusion of the immunoglobulin heavy chain with the sequence near the c-MAF oncogene, a b-ZIP transcription factor.67 Additionally, translocation partners t(9;14) involving the paired box gene 5 (PAX-5) and t(6;14) involving interferon regulatory factor 4 (IRF4) gene have been described.68,69 In the majority of cases, however, the translocating partner chromosome locus is not yet identified. Although 14q32 is one of the common translocations, its role in myeloma pathogenesis remains unclear due to the variety of partner chromosomes involved and its lack of prognostic significance.
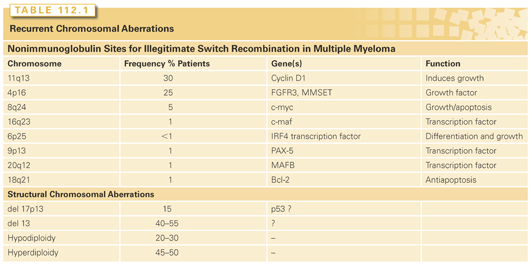
Standard cytogenetics techniques did not identify rearrangements involving 8q24, which contains the c-MYC oncogene and is commonly involved in murine plasmacytoma. However, FISH analyses in one study identified karyotypic abnormalities that involve c-MYC in 45% cases of advanced myeloma.70 Another study using interphase FISH confirmed c-MYC rearrangement in 15% of MM cases, with increasing frequency correlating with severity of disease. Interestingly, c-myc involvement is heterogeneous, suggesting its role in the evolution of disease.71 Changes in c-MYC in the form of either abnormal size transcript or high level of expression have been reported in a majority of patients in one study.72,73
A deletion of chromosome 13 or 13q arm identified by conventional karyotyping confers a poor prognosis, even after high-dose therapy.74 Using the RB1 gene as a probe, a FISH analysis reveals RB1 deletion in >40% of these patients.75 In a detailed analysis of the 13q chromosome using an 11 probe FISH panel, >80% of 50 patients showed molecular deletions, with 13q14 representing a critical region most frequently involved.76 Additionally, constitutive phosphorylation of retiniblastoma (pRB) in myeloma cells can be further enhanced by IL-6.77 Cyclin D, cyclin-dependent kinases (CDK), and CDK inhibitors p15 and p16 (ink), p21 and p27 (cip), and p57 (kip), have also been investigated in myeloma due to their effect on pRB phosphorylation. Abnormalities in p16 and p15 have been reported in 75% and 67% of myeloma patients, respectively, suggesting an important defect in the pRB regulatory pathway.78–80
Mutations involving Ras are observed in about 39% of newly diagnosed MM patients, and are more frequently observed following progressive disease. Activating mutations of the ras oncogenes may also result in growth factor independence and the suppression of apoptosis in MM. One of the commonly altered genes in many malignancies is p53. In myeloma, abnormalities in p53 are detected in less than 10% of patients with early stage disease.81,82; however, p53 abnormalities represent an important late event associated with progression to an aggressive form of the disease. A study of p53 gene mutations in 52 patients with myeloma showed 7 of 52 patients to have p53 abnormalities, all with a clinically advanced and aggressive acute/leukemic stage of MM.81 Murine double minute 2 (MDM2), an important inhibitor of p53 function, is overexpressed in the majority of myeloma cell lines; however, increased MDM2 expression is infrequently observed in primary myeloma cells.83
An important antiapoptotic gene, BCL-2, is uniformly overexpressed in low-grade non-Hodgkin’s lymphoma. In this family of genes, BCL-2 and BCL-XL are antiapoptotic genes, whereas BAX, BAD, and BCLXS are proapoptotic genes. A balance between these genes determines cell survival. The t(14:18) translocation involving the BCL-2 gene is quite rare (2% to 3%) in myeloma. However, numerous myeloma cells lines as well as primary cells, express high levels of BCL-2.84,85 Its relationship to development of drug resistance, as well as radiation resistance in myeloma cells is also well described.86,87 One study in 63 patients showed a significant correlation between BCL-2 expression and resistance to therapy with interferon, but not melphalan and prednisone.88 The association of BCL-2 expression with prognosis remains controversial, as one small study failed to show a correlation with short survival. BCL-XL is upregulated in myeloma cells as a consequence of IL-6–induced activation of signal transducers and activators of transcription 3 (STAT-3).89 It confers a drug-resistant phenotype and, in conjunction with bcl- 2, leads to increased genetic instability. Mcl-1, another antiapoptotic gene, is overexpressed in MM and is upregulated by IL-6. Its overexpression mediates potent resistance to apoptosis, and conversely, its downregulation by antisense oligonucleotide triggers apoptosis. Finally, Bcl-6 expression in MM cells is induced by its interaction with BMSC and is modulated, at least in part, via Janus kinase (JAK)/STAT-3 and canonical nuclear factor kappa B (NF-κB) pathways, and targeting Bcl-6, either directly or via these cascades, inhibits MM cell growth in the bone marrow (BM) milieu.90
High telomerase activity has also been demonstrated in myeloma cells, relative to normal cells and other malignant cell lines.91 Telomerase activity confers growth and survival in MM, and is therefore an additional target for therapeutic intervention.
Transcriptional and Genomic Studies
Gene expression profiling, as well as studies focused on relative gains or loss of genomic DNA, have provided both prognostic and therapeutic information in MM. Molecular diagnostic tools and novel therapeutics now offer the potential for more accurate prognosis and personalized treatment.
The expression profiling studies in MM have provided insight into the progression of MGUS to MM, and provided the basis to predict outcome, as well as to identify potential therapeutic targets.45 Expression studies have identified subtypes within the nonhyperdiploid group associated with specific chromosomal translocations.92 A comprehensive expression profiling the survey of uniformly treated MM patients has identified 70 genes predictive of early disease-related death. Interestingly, one-third of these genes are located on chromosome 1, confirming the potential significance of chromosome 1 in MM pathobiology.93 Patients with a high risk score using this gene signature had significantly shorter complete response (CR) duration (20% patients at 3 years) compared to those patients without such high risk features (60% at 5 years). A multivariate discriminant analysis identified a 17-gene signature that performed as well as the 70-gene model. A second large study by the Intergroupe Francophone du Myelome (IFM) group studied gene expression profiles in 182 newly diagnosed patients and developed a 15-gene model to calculate a risk score associated with overall survival. In this study, the high-risk group had an overexpression of cell cycle progression and its surveillance-related genes, whereas a hyperdiploid signature and heterogeneous gene expression characterized low-risk patients. Overall survival at 3 years in the low-risk group was 91% versus only 47% in the high-risk group, and these results were independent of traditional prognostic factors.94 In a recent study in 320 newly diagnosed myeloma patients from the Dutch–Belgian/German HOVON-65/GMMG-HD4 trial, gene expression profiling identified a 92-gene signature and 10 subgroups in MM, which may represent unique diagnostic entities with potential novel therapeutic targets.95 Additional expression profile studies have begun to define subtypes of myeloma with different prognostic significance following various therapies. An apparent lack of uniformity in the genes identified by these investigations suggests that therapy-related factors may determine the influence of different classes of genes in MM. Based on cytogenetics as well as expression data, cyclin D dysregulation occurs in the early pathogenesis of MM.
A high-resolution analysis of recurrent copy number alterations, coupled with expression analyses in MM cell lines and primary MM cells using array comparative genomic hybridization (aCGH), has identified distinct genomic subtypes. Additionally, this study has defined 87 discrete minimal common regions that have identified gene candidates for targeted drug discovery, improved the understanding of MM initiation and progression, and improved the prediction of clinical outcomes (Fig. 112.3). A high-density, SNP array analysis of myeloma cells from 192 newly diagnosed patients identified genomic copy number alteration in 98% of patients.96 Amplifications in 1q and deletions in 1p, 12p, 14q, 16q, and 22q were the most frequent lesions associated with adverse prognosis, whereas amplifications of chromosomes 5, 9, 11, 15, and 19 were linked to a favorable prognosis. Amp(1q23.3), amp(5q31.3), and del(12p13.31) have been identified in a multivariate analysis to be independent prognostic factors. This prognostic model was validated in an independent validation cohort of 273 patients with myeloma, and identified patients with amp(5q31.3) alone and low serum beta-2-M to have an excellent prognosis (5-year overall survival, 87%) versus patients with del(12p13.31) alone or amp(5q31.3) and del(12p13.31) and high serum beta-2-M with a poor outcome (5-year overall survival, 20%).
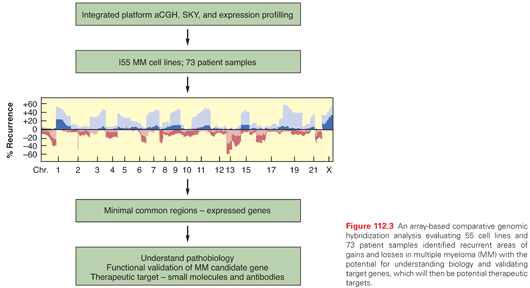
Epigenetic changes modulating myeloma cell growth and survival genes are also reported. For example, methylation of p16, a negative cell cycle regulator, is common and reported to be an early event even in MGUS. However, p16 methylation was not predictive of overall survival in a single large cohort study. The acetyl-lysine recognition domains (bromodomains) of putative coactivator proteins have been shown to play a significant role in transcriptional initiation and elongation as well as chromatin-dependent signal transduction. The bromodomain and extra-terminal (BET) bromodomain proteins have been identified as regulatory factors for c-Myc expression in myeloma, and their inhibition induces antiproliferative effects associated with cell-cycle arrest and cellular senescence in both in vitro and in vivo models.97 A recent global methylation analysis (N = 159) of purified myeloma cells has identified prognostically important, epigenetically inactivated tumor suppressor genes. In this analysis, the combination of DNA methylation and gene expression profile identified hypermethylated GPX3, RBP1, SPARC, and TGFBI genes to be associated with significantly shorter overall survival, independent of other high-risk features such as International Staging System (ISS) stage or adverse cytogenetics.98
A role of microRNAs (miR) and potential therapeutic targeting of multiple mRNAs has recently been described in myeloma. miRNA expression profiling of MM and MGUS cells compared to normal donor plasma cells identified the overexpression of miR-21, miR-106b, and miR-181a and b in MM and MGUS samples, as well as selective upregulation of miR-32 and miR-17-92 in MM, but not in MGUS.99 Another study analyzed the expression level of miRs and the gene expression profile in 60 newly diagnosed myeloma patients, and identified significantly dysregulated miRs expression in cytogenetically distinct subtypes.100 The putative targets and their function are now being defined (e.g., miR-192, 194, and 215), which are downregulated in a subset of newly diagnosed myeloma, are transcriptionally activated by p53 and then modulate MDM2 expression. In addition, miR-192 and 215 target the IGF pathway, prevent the enhanced migration of plasma cells into bone marrow, and are positive regulators of p53, suggesting that their downregulation plays a key role in myeloma pathogenesis.101 Recently, a role for miR-21,102 miR-29,103 and miR-34a104 in supporting myeloma cell growth and survival have been defined, suggesting their potential as novel therapeutic targets.
Mutational changes in myeloma have now been evaluated using whole genome sequencing. An initial report using massively parallel sequencing of 38 tumor genomes and their comparison to matched normal DNAs identified several new oncogenic mechanisms based on the pattern of somatic mutations across the data set. These include mutations of genes involved in protein translation (seen in nearly half of the patients), histone methylation, and blood coagulation as well as NF-κB signaling, evidenced by mutations in 11 members of the NF-κB pathway.105
Using high-resolution SNP arrays (N = 24), clonal content and evolution was evaluated in myeloma. This analysis suggested selection and growth of genetically distinct subclones, which were not initially competitive against the dominant population but survived following therapy, with new acquired anomalies and subsequent outgrowth. These data point to the need to target and eradicate even a minor clone.106 Recently, whole exome sequencing, copy number profiling, and a cytogenetics analysis performed in 84 myeloma samples have identified new candidate genes, including truncations of SP140, Lymphotoxin-beta (LTB), roundabout, axon guidance receptor, homolog 1 (ROBO1), and clustered missense mutations in early growth response protein 1 (EGR1). A further analysis revealed a complex subclonal structure including subclonal driver mutations. Serial sampling was performed in 15 patients, which revealed diverse patterns of clonal evolution: linear evolution, differential clonal response, and branching evolution. Diverse processes contributing to the mutational repertoire including kataegis and somatic hypermutation have been identified, and their relative contribution changed over time. This study demonstrates that the myeloma genome is heterogeneous, with clonal diversity at diagnosis and further evolution over time.107
Microenvironment and Cell Signaling
Myeloma cells express adhesion molecules that mediate interaction with the microenvironment, including both bone marrow stromal cell elements and extracellular matrix proteins (Table 112.2). These adhesion molecules mediate both homotypic and heterotypic adhesion. Adhesion not only plays a role in migration and localization of myeloma cells in the bone marrow, but also induces tumor cell growth and survival. For example, syndecan-1, a cell surface transmembrane heparan sulfate proteoglycan present on MM cells, interacts with type I collagen, and regulates growth of MM cells; it also mediates increased osteoclast activity.108,109 Elevated levels of syndecan-1 shed into serum correlate with increased tumor mass, decreased matrix metalloproteinase-9 activity in the serum, and a poor prognosis.108
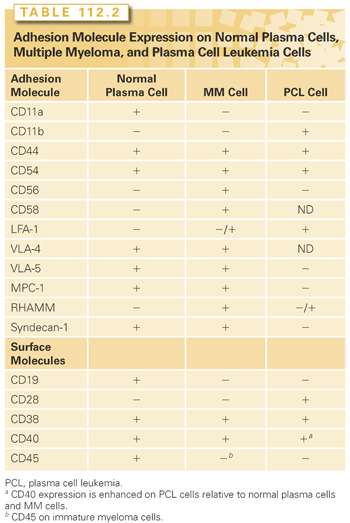
The BM microenvironment consists of a variety of cell types including stromal cells (BMSC), endothelial cells, osteoclasts, osteoblasts, as wel l as immune cells. The physical interaction between myeloma cells and these cells in the BM milieu plays a crucial role in MM pathogenesis both by direct adhesion-mediated signaling, as well as by secretion of factors such as IL-6, IGF-1, vascular endothelial growth factor (VEGF), B-cell activating factor (BAFF), fibroblast growth factor (FGF), stromal cell–derived factor (SDF) 1α, and tumor necrosis factor alpha (TNF-α), which mediate tumor cell growth, survival, drug resistance, and migration.110–114 These interactions lead to the activation of several proliferative/antiapoptotic signaling cascades in MM cells: PI3K/Akt, Ras/Raf/mitogen-activated protein kinase (MAPK) kinase (MEK)/extracellular signal-related kinase (ERK), JAK 2/ STAT-3, and NF-κB pathways. These pathways lead to MM cell growth, survival, antiapoptosis, migration, and development of drug resistance. Additionally, adhesion as well as cytokines secreted from MM cells and accessory cells in turn further augment cytokine secretion from these cells.
Activation of NF-κB has been noted in myeloma cells, especially following their interaction with BMSC. A number of abnormalities contributing to the dysregulation of NF-κB and constitutive activation of the noncanonical NF-κB pathway have recently been described.115 Elevated expression of NF-κB-inducing kinase (NIK) due to genomic alterations or protein stabilization, and inactivating mutations of TNF receptor-associated factor 3 (TRAF3) able to trigger both classical and alternative NF-κB pathways have been reported.116 These alterations activating the NF-κB pathway may allow MM cells to achieve autonomy from the bone marrow microenvironment.117
Each accessory cell in the BM milieu contributes differently to the overall effect of the microenvironment. The biologic and clinical relevance of increased angiogenesis, although established in solid tumors, has only recently been appreciated in hematologic malignancies. Increased bone marrow microvessel density (MVD) has been reported in MM patients compared to individuals with MGUS.118–120 Moreover, the degree of MVD myeloma BM has been correlated with the prognosis.121,122 Immunohistochemical studies show that the angiogenic factor VEGF is expressed by MM cells.123 Hepatocyte growth factor, which also promotes angiogenesis, is increased in the serum of myeloma patients and predicts for poor outcome, especially in patients with increased β2 microglobulin levels.124 Finally, novel agents such as thalidomide inhibit angiogenesis and can also overcome drug resistance in myeloma. Plasmacytoid dendritic cells (pDC), another component of the BM microenvironment, support MM cell growth, survival, and drug resistance, as well as mediate the immune deficiency characteristic of MM. Therefore, targeting pDC–MM interactions represent a therapeutic strategy to both overcome drug resistance and restore immune function in MM.125
Role of Cytokines
Myeloma cells and BMSCs produce cytokines including IL-6,110 IGF-1,114 VEGF,112 SDF-1,113 TNF-α,111 TGF-β,126 IL-21,127 and others that mediate tumor cell growth, survival, antiapoptosis, migration, and the development of drug resistance.
Interleukin 6
IL-6 is an essential growth and survival factor for myeloma. The IL-6 receptor expressed by myeloma cells is composed of two polypeptide components: the α chain (gp80, IL-6Rα) and the signal transducing β chain (gp130). The gp130 component is shared by a family of cytokines including oncostatin M and leukemia inhibitory factor. The interaction of IL-6 with its receptor activates Ras/RAF/MEK/ERK, JAK/STAT, and PI3K/Akt signaling pathways, mediating growth, survival, and drug resistance (Fig. 112.4). IL-6 is mainly produced by stromal cells following the binding of myeloma cells and is also triggered by other cytokines including TNF-α,111 IL-1β VEGF, and IL-17 in the BM milieu.112 IL-6 mediates both autocrine and paracrine growth of myeloma cells. It increases the proportion of cells in S phase, prevents apoptosis of malignant plasma cells, and confers resistance to antitumor agents such as dexamethasone (Dex). Soluble IL-6Rα, shed by myeloma cells into the serum, can amplify the response of myeloma cells to IL-6; both high serum IL-6Rα levels and high serum IL-6 levels portend a poor prognosis. IL-6 and soluble IL-6Rα also mediate enhanced bone resorption by osteoclasts. To date, IL-6 has been targeted therapeutically using antibodies specific for IL-6 or its receptor. However, these treatment approaches have produced only transient responses in a small number of patients.
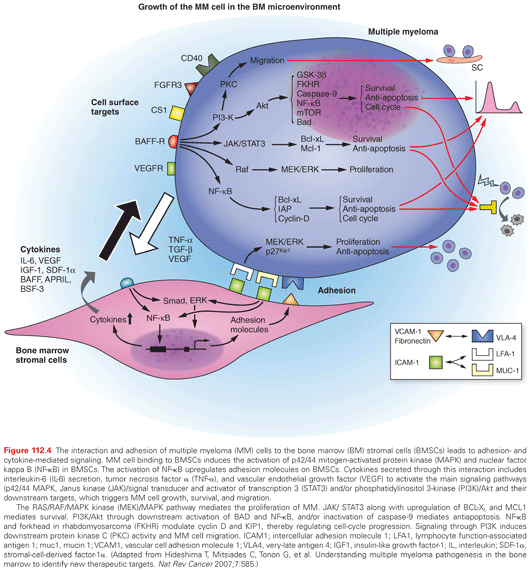
Insulin-like Growth Factor 1
IGF-1 is a growth and survival factor for human MM, which activates PI3K and MAPK signaling pathways mediating proliferation and antiapoptosis.128,129 It induces more potent protection against Dex than does IL-6. IGF-1 upregulates FLICE-like inhibitory protein (FLIP), X-linked inhibitor of apoptosis (XIAP), and Bcl-2-related protein A1 (A1/Bfl1),128 and increases telomerase activity, thereby further enhancing tumor cell growth and survival.130 IGF-1 also mediates the adhesion and migration of myeloma cells via β1-integrin.114 These studies have identified IGF-1 as a novel therapeutic target; both antibodies and small molecule inhibitors against IGF-1 have been investigated in preclinical studies.
Vascular Endothelial Growth Factor
VEGF has only modest proliferative effects on myeloma cells; however, it plays a more important role in triggering tumor cell migration and angiogenesis.131,132 Its production in the BM milieu is upregulated both by myeloma cell adhesion to BMSCs and by IL-6.133 Because it is a specific endothelial cell mitogen, elevated levels in myeloma may account, at least in part, for increased angiogenesis.123 Myeloma cells express fms-related tyrosine kinase 1 (FLT1), and VEGF triggers its phosphorylation and activation of downstream MEK and protein kinase C (PKC) signaling. These data have provided the preclinical rationale to evaluate VEGF as a therapeutic target. Although PTK787, a potent inhibitor of VEGF receptor, has shown antimyeloma activity in vitro,134 it has not shown clinical activity.
Other Cytokines
TNF-α is secreted by myeloma cells and does not have any significant direct effect on myeloma cell growth and survival; however, it induces the secretion of IL-6 by BMSCs. It is also a strong inducer of NF-κB activation, thereby upregulating adhesion molecules, with resultant binding of myeloma cells to BM and cell adhesion–mediated drug resistance (CAMDR). Although specific antibody inhibitors of TNF-α have not shown a clinical response, thalidomide and its immunomodulatpry drug (IMiD) analogs have potent anti-TNF-α activity and can overcome CAMDR.
SDF-1 is expressed by BMSCs, and its receptor CXCR4 is expressed by myeloma cells. It induces only a minimal proliferative effect; however, it plays a more important role mediating migration.
TGF-β is produced by MM cells and induces the secretion of IL-6 by BMSCs; it also contributes to immunosuppression, which is characteristic of myeloma.
Increased serum levels of IL-17, IL-21, IL-22, and IL-23, the proinflammatory cytokines associated with Th17 cells, are also observed in myeloma. IL-17 promotes myeloma cell growth and adhesion to bone marrow stromal cells, as well as contributes to bone disease in myeloma. In combination with IL-22, it also inhibits the production of Th1-mediated cytokines including interferon gamma (IFN-γ).135 IL-21 induces proliferation and inhibits apoptosis independent of IL-6 signaling. It triggers phosphorylation of Jak1, Stat3, and Erk1/2 (p44/42 MAPK). TNF-α upregulates expression of both IL-21 and IL-21 receptor (IL-21R).
Intrinsic and acquired resistance of plasma cells to conventional chemotherapy is common, and as a result, only 50% of patients achieve a partial response, with few complete responses to melphalan. Drug resistance is mediated by several mechanisms.136 An altered intracellular drug concentration may be due to overexpression of the MDR1 gene, encoding for P-glycoprotein, an integral membrane protein that functions as an ATP-dependent drug efflux pump, a multidrug resistance–associated protein (MRP), and a lung resistance–related protein (LRP), a member of the class of major vault proteins.137 To date, however, strategies targeting these mechanisms have not been successful in overcoming drug resistance. Recently, mechanisms operative in inducing resistance to proteasome inhibition have been identified that have included upregulation or activation of Hsp90, Akt, and aggresomal protein degradation. Downregulation of Cereblon or lack of degradation of IKZF1 or 3 has been shown to mediate resistance to immunomodulatory agents.
Phenotype
Myeloma cells display heterogeneous cell surface phenotypes, with differences both between different patients and within the same patient at different disease stages. In general, all myeloma cells express high levels of CD38, with immature plasma cells additionally expressing CD45 and the IL-6 receptor.138–140 More mature myeloma cells do not express CD45 and lack IL-6 receptor expression.141 A subpopulation of myeloma cells may also express CD10, CD56, or CD49e (very late activation antigen 5 [VLA5]).141–143 CD28 expression is associated with more aggressive disease144; CD20 expression is present on 20% to 30% of myeloma patients, and can be further upregulated with IFN-α.145 The identity of the myeloma stem cell still remains an enigma. B cells expressing CD19 and CD11b can be induced to mature on stromal cells into monotypic plasma cells, suggesting that this cellular compartment may contain myeloma cell progenitors.146,147 Using allele-specific oligonucleotide polymerase chain reaction (PCR) and the severe combined immunodeficiency (SCID)-hu model, the myeloma stem cell will be better defined in the future. These cell surface characteristics have also allowed for the development of eight color flow cytometries to measure minimal residual disease.
Immune Status
Myeloma patients present with suppressed immune function due to a variety of factors. Most significant is the suppression of uninvolved immunoglobulins (e.g., in patients with IgG myeloma, there is suppression of serum IgA and IgM levels).148 The factors causing this suppression include a direct effect of monoclonal immunoglobulin, increased soluble Fc receptor or Fc expressing cells, suppression of helper cell functions, monoclonal Ig, and macrophage-related factors that affect B-cell maturation to plasma cells.149 The recovery of uninvolved immunoglobulins to normal levels following effective therapy has been associated with both improved survival and protection from infectious complications.
The total T-cell count may be decreased; however, in a substantial number of patients, it may be normal, with no significant changes in CD8 cells.150–152 A stage-dependent suppression of natural killer (NK) cells has been observed.153 Deficiency of CD4 helper cells is also pronounced.154 In one study, the proliferation and frequency of Epstein Barr virus (EBV)- and influenza A (inf A)- specific T cells was significantly reduced in a cohort of 24 newly diagnosed or conventionally treated MM patients when compared with 19 healthy individuals, suggesting an impaired response of CD8+ T cells in MM patients.155 Although a defect in NK T-cell function has also been detected in patients with progressive myeloma compared to patients with MGUS or nonprogressive disease,156 invariant NK T cells from myeloma patients can be activated and expanded in vitro.157 Dysfunctional T regulatory cells have been associated with disturbed immune homeostasis in both MGUS and MM.158 Elevated levels of IL-17 producing Th17 cells, possibly related to high IL-6 and transforming growth factor beta (TGF-β) expression, have also been described in myeloma, and promote myeloma cell growth, immune suppression, and osteoclast function.135 CD11b(+)CD14(−)HLA-DR(−/low)CD33(+)CD15(+) myeloid-derived suppressor cells (MDSC) are a heterogeneous, immature myeloid cell population that is also significantly increased in both the peripheral blood and the bone marrow of patients with active MM; these cells both induce MM growth and suppress T-cell–mediated immune responses.159
Anti-idiotype T-cell response has been demonstrated in the majority of patients, with higher Id-specific T-cell frequency in MGUS and early stages of myeloma compared to advanced disease.160 This observation has lead to a provocative hypothesis that immunologic response plays an important role in controlling the proliferation of the malignant clone in early stages of the disease, whereas loss of immune regulation is associated with evolution to an overt or more aggressive form of the disease. These data also provide the scientific basis to induce idiotype-specific T-cell responses for therapeutic application through either vaccination in vivo or the production of idiotype- or myeloma-specific cytotoxic T lymphocytes (CTL) in vitro.161
Murine Models
Three different models of murine myeloma have been described to study myeloma pathogenesis. In an inbred C57BL/Ka strain of mice, 16% spontaneously developed monoclonal gammopathies without tumor formation by 2 years.51,52,162 In another model, BALB/c mice with a low spontaneous incidence of monoclonal gammopathies, the induction of plasmacytoma or myeloma is observed after the intraperitoneal injection of mineral oil or pristane.51 Plasmacytomas, which develop within the oil, or other foreign body–mediated granulomas and lymphoplasmacytic infiltration can be blocked by the administration of indomethacin and accelerated by subsequent infection of the mice with Abelson’s virus. Interestingly, however, the C57BL/Ka strain, with a high incidence of spontaneous monoclonal gammopathies, is relatively resistant to the induction of plasmacytoma by mineral oil. Plasmacytoma progression is associated with the dysregulated expression of c-myc as a result of translocation analogous to t(8;14) in humans. These plasmacytomas produce IgA immunoglobulins, and a growth factor present in the peritoneal fluid has been confirmed to be IL-6. Additionally, when animals are raised in a germ-free environment, the incidence of myeloma after mineral oil stimulation is markedly reduced, whereas that of other lymphoid neoplasms increases.51 These studies suggest an important role of immune stimulation in myeloma development. A third model uses subcutaneous growth of murine plasmacytoma cell lines such as MOPC11 in immunocompetent mice, which allows for the study of immune modulation.
Human myeloma cell lines can grow and disseminate in a SCID mouse model, providing a unique opportunity to study this disease in an in vivo setting.163 The introduction of fetal human bone into SCID mice (SCID-hu) has allowed for the engraftment and proliferation of the stromal cell–dependent human myeloma cell line164 as well as primary human myeloma cells165,166 in >80% mice and the monitoring of tumor burden by the detection of human myeloma–specific protein (soluble IL-6 receptor) or human Ig and light chains in murine blood samples, respectively. In this murine model of primary human disease, the fetal bone undergoes osteoporotic and osteolytic change as a consequence of clonotypic plasma cell proliferation and production of human cytokines. This model provides a unique opportunity to study the importance of stromal cell–myeloma cell interactions, as well as genetic and molecular mechanisms critical for myeloma growth and dissemination in vivo, and may provide clues to the origin of myeloma stem cells, thereby providing the opportunity to evaluate new treatment approaches targeting the myeloma cell and its microenvironment and bone disease in myeloma. A transgenic Eu-directed X-box Binding Protein-1 (XBP-1) spliced isoform mouse model has been generated in which mice develop features of MGUS and progress to MM.167 XBP-1 is a transcription factor that is required for plasma cell differentiation, which is expressed at high levels in MM cells versus normal plasma cells.168 This model provides a unique opportunity to study the biology of human myeloma and assess novel therapies in vivo. Recently, conditional MYC transgene expression has been demonstrated in Vk*MYC mice, which eventually develop an indolent multiple myeloma with features characteristic of human disease. This model serves to highlight the role of myc in myeloma and provides a unique animal model to test therapeutic or preventative strategies in myeloma.169
Patients with MM may be entirely asymptomatic and diagnosed on routine blood work or may present with a myriad of symptoms such as hematologic manifestations, bone-related problems, infections, various organ dysfunctions, neurologic complaints, or bleeding tendencies (Table 112.3). These signs and symptoms result from direct tumor involvement in BM or extramedullary plasmacytomas, the effect of the protein produced by the tumor cells deposited in various organs, the production of cytokines by the tumor cells or by the BM microenvironment, and effects on the immune system.
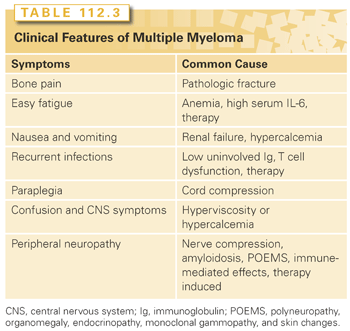
Anemia
A normochromic normocytic anemia is usually observed in myeloma patients due to tumor cell involvement of the marrow as well as inadequate erythropoietin responsiveness. The suppressive effects of various cytokines on erythropoiesis and the effect of renal dysfunction on erythropoietin production are also contributing factors. High immunoglobulin levels exacerbate the anemia due to dilutional effects. Anemia gives rise to fatigue, weakness, and occasionally, shortness of breath. Erythropoietin (Epo) administration is, therefore, an important supportive care therapy for patients with symptomatic anemia. In one study, improvement in hemoglobin by more than 2 g per deciliter was observed in 60% of treated patients, and responses were more frequent in patients with low Epo levels than in patients with normal or high levels (72% versus 20%).
Renal Failure
Nephropathy is one of the serious adverse complications that can be observed at the time of clinical presentation. The etiology of renal failure can be multifactorial. The most common cause is the development of light chain tubular casts leading to interstitial nephritis (myeloma kidney).170 Another common cause of renal dysfunction is hypercalcemia leading to osmotic diuresis, volume depletion, and prerenal azotemia. Other modes of kidney involvement in myeloma include light chain deposition disease, which is more commonly associated with kappa light chain proteins and impaired glomerular filtration; AL amyloidosis, which is more frequently associated with lambda light chain (especially lambda light chain subtype VI) and may have an initial presentation as nephrotic range proteinuria; and renal calcium deposition, leading to interstitial nephritis.171–173 The presence of lambda light chains in the urine is also more commonly associated with myeloma kidney. Bence Jones proteins bind to a common peptide segment of Tom-Horsfall glycoprotein to promote heterotypic aggregation and deposition in the kidney.174 Additional factors exacerbating renal failure in myeloma patients include the use of nonsteroidal anti-inflammatory drugs for pain control, hyperuricemia, nephrotoxic chemotherapeutic agents, intravenous contrast for radiographic studies, bisphosphonate therapy, as well as calcium deposition and stones in the kidney. The proteinuria observed in patients with amyloidosis is more often nonspecific, which can help to differentiate it from typical myeloma-related kidney disease characterized by excessive light chain excretion.175 Pathologic renal changes similar to human myeloma–related nephropathy develop in IL-6 transgenic mice expressing IL-6 under metallothionein-1 promoter, indicating a relationship between constitutive high IL-6 expression in the liver, dysproteinemia and long acute-phase response, and renal changes.176
Hypercalcemia and Bone Disease
The mechanism of bone abnormalities in myeloma, especially destruction, is an unbalanced process of increased osteoclast activity and suppressed osteoblast activity. These changes are due to an increase in osteoclast-activating factors produced predominantly by the BM microenvironment and also by myeloma cells.177,178 These factors include IL-1β, TNF-β (lymphotoxin), IL-6, and macrophage inflammatory proteins-1 (MIP-1) alpha.179–182 The receptor activator of nuclear factor kappa B ligand (RANKL) plays an important role in osteoclast differentiation via its receptor located on the osteoclast membrane. A member of the TNF family, it was originally described as a factor secreted by T cells, which induces maturation of dendritic cells. RANKL is also secreted by stromal cells and osteoblasts and induces differentiation and maturation of osteoclast progenitors. Moreover, its production is elicited by factors such as parathyroid hormone (PTH), PTH-related peptide (PTHrP), and OAFs.178,183 Osteoprotegerin (OPG) acts as a decoy receptor for RANKL,184,185 and has been implicated in the development of bone changes in myeloma. Additionally, a recently identified soluble factor produced by myeloma cells, DKK-1, inhibits osteoblast activity and is being therapeutically targeted.186,187 Similarly, activin A, a TGF-β family member that induces osteolysis by inhibiting osteoblast differentiation via SMAD2-dependent distal-less homeobox-5 downregulation, is now being targeted therapeutically.188
All of these factors contribute to the development of osteoporosis and lytic bone lesions. Radiographic findings of such destruction are shown in Figure 112.5. These bone changes frequently involve the vertebral column and result in compression fractures, lytic bone lesions, and related pain.
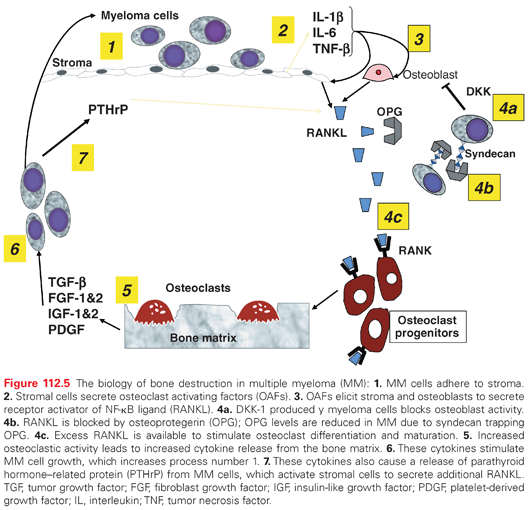
A new onset of back pain or other bone pain is a frequent presenting symptom in myeloma patients. Changes in the cytokine milieu and bone destruction may also lead to the development of hypercalcemia, which is observed in approximately 25% of patients at some stage of the disease. Symptoms of high calcium include mental status changes, lethargy, constipation, and vomiting. High paraprotein levels, low albumin levels, or both are commonly observed in patients with myeloma, and require measurement of ionized calcium. Hypercalcemia may also contribute to renal failure and should, therefore, be considered an oncologic emergency requiring prompt intervention.
Infections
Myeloma patients are at risk for developing recurrent bacterial infections due to deficiencies in both humoral and cellular immunity.149,189,190 Various factors including high monoclonal immunoglobulin levels, soluble Fc receptor in serum, and TGF-β lead to the suppression of B-cell function, which in turn leads to depressed uninvolved immunoglobulins.126,191 This impairment in the patients’ ability to mount humoral responses predisposes patients to infections with bacteria that are ordinarily opsonized by antibodies against bacterial antigens. Patients also have profound T-cell dysfunction due to various immunosuppressive cytokines such as TGF-β and IL-6 secreted by the microenvironment and fas ligand, which is present on the membrane of myeloma cells. Additional causes of immune suppression include programmed death-ligand 1 (PD-L1) and programmed cell death protein 1 (PD-1) expression on MM cells and MM T cells, respectively, as well as increased T-regulatory cells in MM. The therapy for myeloma, especially high-dose corticosteroids, increases infection-related risks in these patients. Therapy with bortezomib is also associated with a higher frequency of herpes zoster.192 The highest risk of infection is within the first 2 months of initiation of therapy, as well as in patients with renal failure and in those with relapsed and refractory disease. Recurrent bacterial, fungal, and viral infections in myeloma require prompt diagnosis and treatment with additional prophylactic measures while receiving immunosuppressive therapy. Infections are an important cause of morbidity and the most common cause of death in patients with myeloma.193
Neurologic Symptoms
The most common cause of neurologic abnormalities is related to a tumor mass effect, especially compression of the spinal cord or cranial or spinal nerves. This may present as motor or, less frequently, sensory neuropathy. An interesting constellation of symptoms described as POEMS syndrome (polyneuropathy, organomegaly, endocrinopathy, monoclonal gammopathy, and skin changes) is observed in osteosclerotic myeloma with prominent sensory neuropathy.194–197 The biologic and cellular basis of these manifestations is not yet well understood. Additionally, neurologic symptoms may occur as a consequence of hypercalcemia or hyperviscosity. Leptomeningeal involvement in myeloma with manifestations involving the central nervous system (CNS) has been described, usually in the late phase of the disease and associated with high-risk chromosomal abnormalities, plasmablastic morphology, and extramedullary manifestations.198,199 Paraneoplastic CNS syndromes have also been described, possibly related to an immune mechanism directed at proteins present in the CNS, including the cerebellum. Peripheral neuropathy in myeloma may be due to an infiltrative process associated with the deposition of amyloid protein in the paraneural or vasa nervorum; due to a metabolic abnormality such as hypercalcemia, uremia, or hyperviscosity; or mediated by an autoimmune process or cytokines.200 Peripheral neuropathy is also observed in patients with MGUS and more frequently associated with IgM paraprotein. More recently, peripheral neuropathy has been observed frequently with therapeutics, including thalidomide and bortezomib, especially with their prolonged use.
Hyperviscosity
The M components in myeloma can cause hyperviscosity and compromise circulation when the serum immunoglobulin levels exceed certain levels. The incidence is highest in Waldenström’s macroglobulinemia with IgM, followed by IgA myeloma (25% patients), and is least common in IgG myeloma (<10% patients).201–203 It can also be observed when immunoglobulins have a self-aggregating property leading to increased viscosity—for example, the IgG3 subclass is more commonly associated with hyperviscosity.204 The syndrome is usually observed when serum viscosity exceeds 4.0 centipoise (cP) units relative to normal serum and manifests with circulatory compromise involving the CNS, the kidneys, and the lungs; it may also be associated with bleeding complications. Due to varying characteristics of idiotypes, the same level of increased viscosity may produce different severities of symptoms in individual patients. A high level of suspicion for this syndrome is important in any patient with paraproteinemia and either mental status changes or pulmonary distress, because prompt plasmapheresis can alleviate symptoms and avoid irreversible organ damage.
Coagulopathy
Myeloma patients may acquire coagulation abnormalities related to a high level of paraprotein interfering with the normal coagulation cascade or exhibit specific antibody activity leading to a clinical syndrome similar to acquired deficiency of factor VIII.205,206 Additional factors, such as thrombosis in capillary circulation associated with hyperviscosity and anoxia, may lead to coagulation-related complications in 15% of patients with IgG myeloma and in more than 33% of patients with IgA myeloma. Although platelet counts are not suppressed in the early stages of myeloma, functional abnormalities of platelets have been described and may also contribute to bleeding.
Acquired activated protein C resistance is reported as a common single transitory baseline coagulation abnormality associated with venous thrombo embolism (VTE) in myeloma patients.207 Additionally, patients may also present in a hypercoagulable state related to acquired deficiencies in protein S, or lupus anticoagulants leading to thromboembolic complications.208 The fab fragment of the myeloma protein binds to fibrin and may prevent its aggregation.209 Factor X deficiency is reported in patients with systemic AL amyloidosis210; however, an inhibitor has not been demonstrated in vitro to account for this manifestation.
Therapy may also increase the hypercoagulable state in myeloma. An increased incidence of deep venous thrombosis (12% to 24%) is observed in patients taking thalidomide and lenalidomide, especially along with dexamethasone or other combination chemotherapies. In one study, 12 out of 50 patients (24%) receiving thalidomide developed deep vein thrombosis (DVT), compared to 2 out of 50 (4%) patients receiving identical therapy without thalidomide.211 Activated protein C resistance in the absence of factor V Leiden mutation and high serum homocysteine levels are associated with an increased risk of thrombotic complications with thalidomide.212
Extramedullary Disease
Extramedullary disease manifestations are uncommon in patients with myeloma at presentation. However, such manifestations have been observed more frequently in the setting of advanced stage disease or relapse following allogeneic transplantation. In a large study of 1,965 patients, primary extramedullary plasmacytoma was detected in 66 patients and secondary plasmacytoma was detected in 35 patients. The most common sites for extramedullary disease at diagnosis were the skin and soft tissues, whereas liver involvement was most prominent at relapse or progression. Extramedullary involvement may be suspected in patients who have more aggressive features of myeloma, including high lactate dehydrogenase levels, immunoblastic morphology, high tumor cell labeling index, and complex karyotypic features.213 In the 70- and 80-gene risk models, it is associated with high-risk features and shorter survival.214
Diagnosis
As myeloma patients present with a variety of symptoms not specific to the disease, the diagnosis of myeloma is quite often delayed. An older patient with a new onset of unexplained back pain or bone pain, recurrent infection, anemia, or renal insufficiency should be screened for myeloma. Additional findings, including hyperproteinemia or proteinuria, anemia, hypoalbuminemia, low immunoglobulin levels, or marked elevation of erythrocyte sedimentation rate, should prompt for a further complete evaluation for a diagnosis of plasma cell myeloma.
The first step in the evaluation includes tests to confirm the presence, type, and quantity of monoclonal protein, as well as the detection and quantification of clonal plasma cells (Table 112.4). The second component to differentiate MGUS, SMM versus symptomatic myeloma is to identify end organ damage by performing a hemogram to detect anemia, a complete skeletal radiographic survey to detect bone lesions, and a chemistry profile to detect renal dysfunction and hypercalcemia. The diagnostic criteria for MGUS, SMM, and active MM are shown in Table 112.5.215 A third component in the investigative workup involves an evaluation of prognostic variables including markers of tumor burden, genomic profiles, and therapy-related changes. Guidelines by the International Myeloma Workshop and National Comprehensive Cancer Center Network summarizes the standard investigative workup in myeloma.216,217
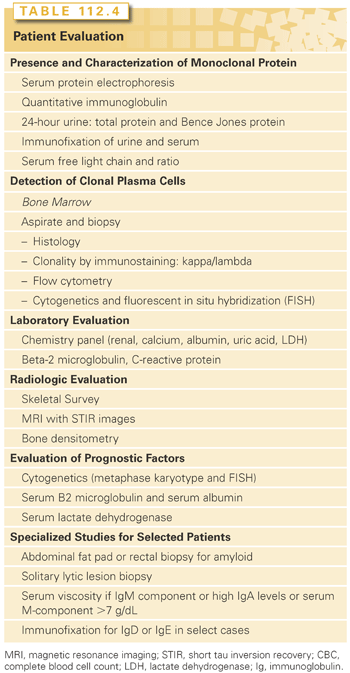
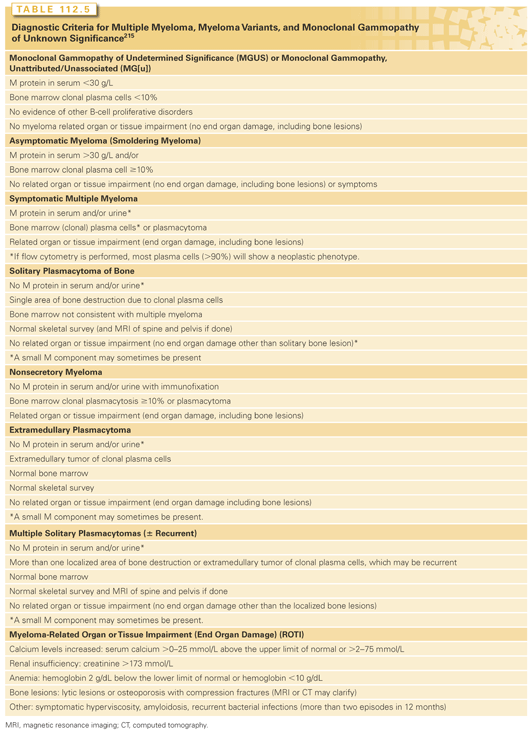
Protein Electrophoresis
Among patients with myeloma, 70% have IgG, 20% have IgA, and 5% to 10% have production of monoclonal light chains only. A small proportion (less than 1%) of patients produce monoclonal IgD, IgE, IgM, or have nonsecretory myeloma. The suppression of uninvolved immunoglobulins (e.g., IgM and IgA in IgG myeloma) is present in a majority of the patients at diagnosis. Suppression of all three major classes of immunoglobulins should raise the possibility that the patient may have light chain–only disease, IgD or IgE myeloma, or nonsecretory disease. Patients producing intact immunoglobulin can also have excess light chain production and excretion in the urine (Fig. 112.6). The distribution of κ and λ light chains in the majority of myeloma cases is similar, except in IgD myeloma in which the λ light chain is more common. Currently, there is no difference in therapeutic approach between the different types of myeloma; however, patients with IgA myeloma, despite a higher initial response rate, have inferior survival.
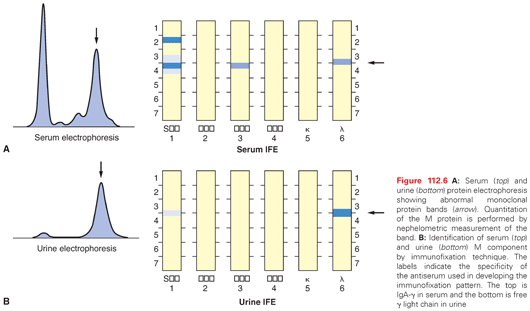
Myeloma plasma cells usually produce a single, abnormal, and unique monoclonal antibody with a constant isotype and light-chain restriction. Rare occurrences of biclonal and triclonal cases have been reported at the time of diagnosis.218 Occurrence of isotype switch and the appearance of abnormal protein bands have been reported in myeloma patients after treatment, especially high-dose therapy,219 which appears to be related to the recovery of normal immunoglobulin production rather than alteration in disease biology. This change is also associated with improved survival. Occasionally, patients with initial intact Ig production relapse with only Bence Jones proteinuria (light chain escape) or nonsecretory disease, and this change has been correlated with more aggressive disease.220
A further analysis of a unique variable region in the myeloma-related idiotype (e.g., CDRIII) provides information on the monoclonal nature of the protein and also provides a tool to investigate minimal residual disease.221
Serum Free Light and Heavy/Light Chain
The measurement of serum free light chain (FLC) concentration has become an important tool to diagnose and follow the disease process, including response to therapy. It is especially useful in patients with light chain–only disease, oligo or nonsecretory myeloma, renal disease, amyloidosis, and as a prognostic marker, in MGUS and SMM, where an abnormal kappa/lambda free light chain ratio predicts a higher likelihood of progression to active myeloma.222–226 Recently, a novel heavy/light chain (HLC) assay has been developed to quantitate the different light chain types of each immunoglobulin class (e.g., IgGκ, IgGλ, IgAκ, and IgAλ), which has been applied for both response assessments227 and for prognostication.228 For example, in a study where both HLC and FLC testing was utilized in 156 patients with IgG or IgA myeloma, the HLC ratio identified the presence of disease in 8 out of 31 patients with CR by conventional criteria.
Bone Marrow Examination
Various degrees of BM infiltration are observed in myeloma, with the majority of patients having an excess number of plasma cells. The pattern of BM involvement (diffuse versus nodular) is important, because patients with nodular disease seem to have poorer outcomes (in contrast to CLL).229 The morphology of the plasma cell seems to be an important factor determining the severity of the disease. This is based on a histologic examination (Bartl grade) in which grade I suggests a slow growing disease, whereas grade III represents plasmablastic disease with an aggressive course.230 There is also an increased incidence of cytogenetic abnormalities in patients with higher grade disease. Plasma cells contain cytoplasmic immunoglobulins with a constant heavy and light chain, which can be evaluated by a flow cytometric analysis or immunohistochemical staining of plasma cells.231 When coupled with DNA staining using propidium iodide, a two-parameter analysis can detect changes in DNA content in myeloma cells (Fig. 112.7). DNA aneuploidy is observed in the BM of more than 80% patients, suggesting the existence of chromosomal abnormalities in the majority of patients.231 This analysis also provides an objective marker to evaluate the response to therapy and to distinguish reactive from clonal plasmacytosis, especially in nonsecretory disease. A hypodiploid tumor cell has also been associated with refractoriness to standard-dose therapy.
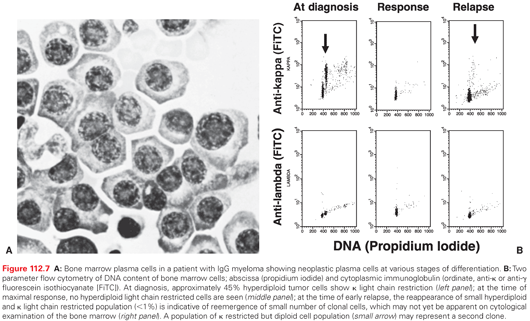
Radiographic Evaluation
The radiographic survey of bone still remains a standard diagnostic evaluation, which shows osteopenia in an early phase of the disease and lytic punched out lesions associated with increasing tumor burden (Fig. 112.8). Osteosclerotic lesions are observed in POEMS syndrome.194,196 Due to the predominant osteoclastic activity with osteoblastic inactivity, bone scans are seldom positive and are therefore not useful in the diagnosis of MM.
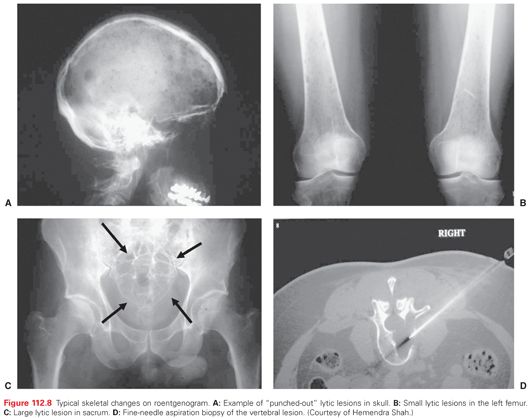
As demineralization of bone (osteoporosis) is one of the common manifestations of myeloma, measurement of bone mineral density (BMD) by dual-energy X-ray absorptiometry (DEXA) is an important evaluation at diagnosis.232 In a study of 66 patients at diagnosis, the majority of the patients had decreased BMD with lumbar mean BMD value (Z score) −1.24 +/− 1.45. Following standard-dose therapy, lumbar BMD increased by 0.7%, whereas, in a group treated with high-dose therapy, the improvement was by 4.6% (p = 0.02).233 Similar improvements in BMD have also been noted in patients undergoing high-dose therapy with the addition of bisphosphonates.234 Differential effects of pamidronate on cortical and cancellous bone have been described in patients with myeloma undergoing autotransplants.233,234
Magnetic resonance imaging (MRI) of bone marrow provides a better assessment of tumor burden. More than 95% of myeloma patients have MRI abnormalities: one-third each have diffuse involvement of the bone marrow, focal lesions, or heterogeneous focal and diffused marrow involvement (Fig. 112.9A). Because myeloma is a macrofocal disease, random BM sampling may not be diagnostic or predictive of disease status, and MRI short tau inversion recovery images (STIR) may provide a better assessment of bone marrow involvement in myeloma.235–237 An MRI of the spine and pelvis is required in all patients with a solitary plasmacytoma and SMM, both to detect occult lesions and to predict progression. In symptomatic myeloma, an MRI can be considered as a routine evaluation to detect unsuspected focal lesions and plasmacytomas involving the spine and pelvis, to define patterns of bone marrow involvement (i.e., diffuse pattern or a high number of focal lesions), to obtain a detailed evaluation of a painful area of the skeleton, and to investigate suspicion of cord compression.238,239 A focal marrow plasmacytoma can be further analyzed through computerized tomography (CT)-guided fine needle aspiration (see Fig. 112.8), allowing for cytologic diagnosis. With effective therapy, the MRI pattern may change (Fig. 112.9B): Diffuse involvement of the marrow may evolve into focal disease, and normalization of MRI abnormalities may provide a better definition of complete responses.237 Positron-emission tomography (PET) scanning has also been evaluated in a small number of studies and may provide a better functional definition of lesions observed on MRI or CT scans, as well as allowing for the selection of lesions for biopsy.237,240 The PET–CT scan is utilized for the detection of extraosseous soft tissue masses, as well as for the evaluation of rib and appendicular bone lesions. A combination of PET–CT and MRI scans may improve the diagnostic accuracy for solitary plasmacytoma.238 A recent study has identified the independent predictive value of baseline fludeoxyglucose (FDG)-PET/CT and of FDG suppression before high-dose therapy.241
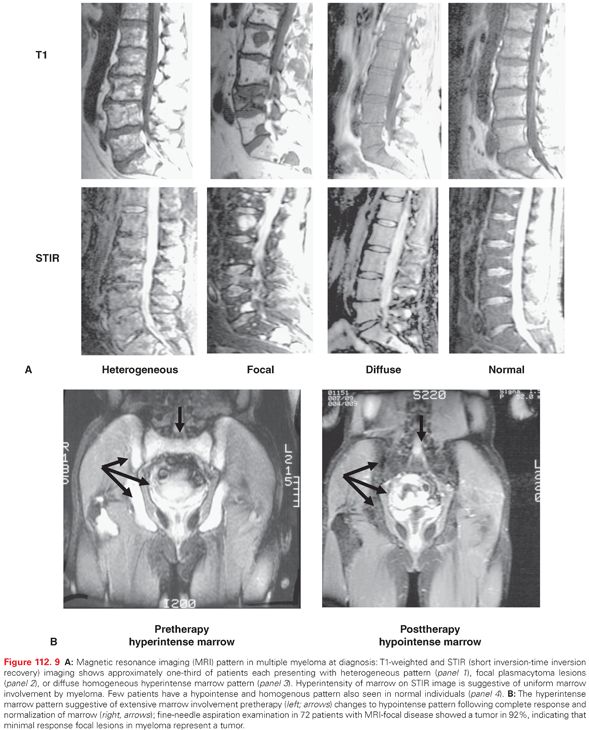
Differential Diagnosis
In the presence of end organ damage associated with monoclonal protein and clonal plasma cells in bone marrow, the distinction of active myeloma from SMM and MGUS can be readily established (see Table 112.5). Patients with nonsecretory myeloma are diagnosed based on marrow plasmacytosis and the presence of bone lesions. MRI abnormalities and CT- or MRI-guided fine-needle aspiration biopsy of involved anatomic sites are important for the follow-up of the disease.
The diagnosis of solitary plasmacytoma of bone or soft tissue requires intense investigation to rule out systemic disease. Bone marrow examination in a true solitary lesion is normal, with no evidence of a clonal cell population. An MRI evaluation for myelomatous involvement of the BM helps detect early lesions before their detection by standard roentgenographic examination. The detection of such lesions and cytologic confirmation through CT- or MRI-guided fine-needle aspiration biopsy may help confirm a solitary plasmacytoma and its genetic makeup. In an individual with MGUS, such detection may change the diagnosis to solitary plasmacytoma or MM. It is important to note that patients with MGUS or solitary plasmacytoma seldom have suppression of uninvolved immunoglobulins. Conventional cytogenetic results are usually normal in MGUS; however, monoclonal plasma cells in some individuals with MGUS may be aneuploidy, and chromosomal abnormalities including IgH translocations and chromosome 13 deletions of unclear prognostic significance have been reported in MGUS.
Besides plasma cell neoplasms, various other conditions can present with monoclonal immunoglobulin secretion. These conditions include other B-cell neoplasms including CLL and B-cell non-Hodgkin’s lymphoma; autoimmune conditions such as cold agglutinin diseases, mixed cryoglobulinemia, hypergammaglobulinemia, and Sjögren’s syndrome; inflammatory or storage diseases such as lichen myxedema, Gaucher’s disease, sarcoidosis, and cirrhosis; and rarely, other malignancies such as chronic myeloid leukemia as well as colon, breast, or prostate cancer.
Protein deposition disease involving various organs requires additional special diagnostic procedures. Deposition of amyloid protein (amyloidosis) can be clinically suspected based on macroglossia, vascular fragility (raccoon’s eyes, periorbital subcutaneous hemorrhages), carpal tunnel syndrome, organomegaly, nephropathy, and cardiomegaly with arrhythmia. The detection of Congo red-positive amyloid with classic apple-green birefringence when visualized under polarized light in perivascular areas and subcutaneous fat, as well as bone marrow or rectal biopsy specimens, are diagnostic of AL amyloid. Electrocardiography may reveal low voltage, and an echocardiographic evaluation shows thickening of the interventricular septum or classic speckled pattern in the myocardium. Endomyocardial biopsy may establish the diagnosis of cardiac amyloid, which is usually associated with elevated serum brain natriuretic peptide (BNP) levels. Another manifestation of amyloid deposition includes autonomic dysfunction due to amyloid deposition in the vasa nervorum of the autonomic nerves, leading to orthostatic hypotension. Amyloid deposition in adrenal glands leads to hypoadrenalism; in the spleen, it may lead to hyposplenism with thrombocytosis; in the liver, it may be suspected based on elevated alkaline phosphatase and γ-glutamyl transpeptidase; and in the gastrointestinal tract, it may lead to malabsorption syndrome. Renal dysfunction must be further investigated with a renal biopsy, because light chain cast nephropathy or light chain deposition disease may be reversible following aggressive therapy, whereas deposition of amyloid requires a different therapeutic approach. As the deposition of immunoglobulin and light chain can mimic many manifestations of AL amyloid, an immunofluorescence analysis of unfixed tissue is important for a diagnosis.
Staging and Risk Assessment
Following the diagnostic investigation, more detailed cellular and molecular studies are required to stage myeloma and evaluate prognostic variables that determine the patient’s probable outcome.
Patients with MM have variable disease courses, with survival ranging from less than 1 year with high-risk aggressive disease to more than 10 years with indolent presentation or sensitive disease. Various characteristics have been identified to predict the possible course of the disease. An evaluation of prognostic factors is important to define therapeutic strategies, permit comparison of clinical trial results, and predict life expectancy after diagnosis. The current risk stratification is applicable to newly diagnosed patients using parameters obtained at the diagnosis. On relapse, there is often an acquisition of additional or new risk features in which case patients should be reclassified as having high-risk disease. As shown in Table 112.6, prognostic factors are related to the tumor burden, the intrinsic property of the tumor, host and microenvironmental influences, and treatment/intervention-related factors.
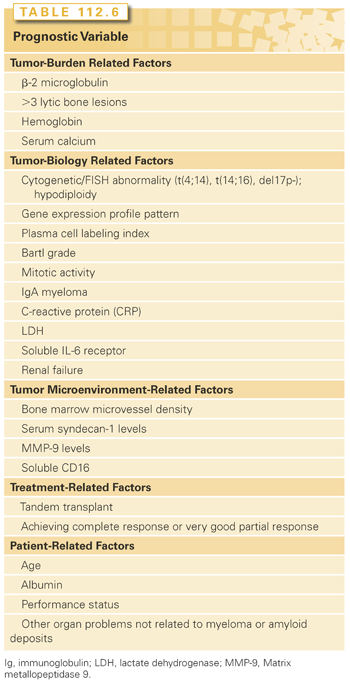
A clinical staging system for MM using a standard laboratory measurement was developed by Durie and Salmon, which was predictive of clinical outcomes after standard-dose chemotherapy.242 However, this staging system is not predictive of outcomes in patients undergoing high-dose chemotherapy as well as novel agents-based therapy and is no longer used clinically.
β2-microglobulin (β2M) has been identified as one of the most consistent predictors of survival in plasma cell myeloma. β2M, the light chain gene of the class I histocompatibility antigens expressed on the surface of all nucleated cells, is shed into the blood. Its renal excretion explains its elevation in renal failure. In MM, β2M, therefore, reflects both tumor burden and renal function.243,244 High β2M (>2.5 mg per liter) levels carry a poor prognosis for treatment with both standard-dose and high-dose therapy.245 The combination of serum β2M along with serum albumin has been proposed as a three-stage ISS (Table 112.7). Although this system predicts for outcome following both high-dose therapy as well as novel agents-based treatment, it lacks consideration of tumor biology–related factors, such as cytogenetics or molecular markers.
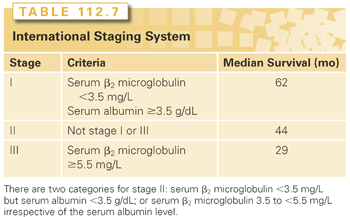
Cytogenetics
Because the myeloma cell represents a mature differentiated cell with low proliferative activity, cytogenetic abnormalities are not frequently detected. Abnormalities are observed in only one-third of the patients at the time of diagnosis; however, a repeated analysis increases the yield to almost one-half of patients. The normal karyotypic pattern observed in the remaining half most likely originates from dividing normal hematopoietic cells.53
Cytogenetic abnormalities have been identified as a major prognostic factor in plasma cell myeloma. Although the detection of any cytogenetic abnormality is considered to suggest a higher risk disease, the specific abnormalities considered as poor risk are cytogenetically detected chromosomal 13 or 13q deletion and detection by FISH of t(4;14); t(14;16) and del17p. Del13 or 13q- detected only by FISH and in the absence of other abnormality does not carry significantly higher risk, whereas t(11;14) does not predict superior outcome. The cytogenetic and interphase FISH analysis has identified numeric aberrations involving trisomies of chromosomes 3, 5, 7, 9, 11, 15, 19, and 21, which predict for favorable outcomes.55 Limited studies have shown that 1q34+, and del1p may have clinical significance as a poor risk feature. Importantly, both bortezomib and lenalidomide are able to overcome adverse outcomes associated with chromosome 13 deletion and, to a lesser extent, t(4;14).246
Other independent factors associated with poor prognosis include elevated C-reactive protein (CRP), elevated serum lactate dehydrogenase (LDH) with extramedullary disease, serum IL-6, serum soluble IL-6 receptor, and IgA isotype.247 Because CRP levels reflect IL-6 activity and elevated CRP levels can be associated with acute-phase reactions including inflammation and infections, the predictive value of elevated CRP in myeloma is important only when other possible causes for its elevation are ruled out. BM plasmacytosis reflects tumor burden, but does not predict survival. Peripheral blood monoclonal plasma cells predict for survival in myeloma: In a study of 254 patients, blood monoclonal plasma cell counts ≥4% in 57% patients were associated with a median survival of 2.4 years compared with 4.4 years in patients with less than 4% circulating plasma cells.248
Among the various other disease biology–related variables, plasma cell proliferation rate, as measured by the labeling index (LI), is a valuable prognostic factor: Early in the disease, the proportion of myeloma cells in the cell cycle is small; bromodeoxyuridine or tritiated thymidine methods show a median of 1% cycling cells at the diagnosis. With progressive disease, the LI increases, suggesting a more proliferative phenotype. The LI has important prognostic significance, because patients with more than 1% cells in the S-phase in BM have worse outcomes231,249,250; however, the lack of standardized reproducible methods to measure the LI has limited its use as a prognostic marker. One study combining β2M and LI identified a low-risk group with both parameters low, an intermediate-risk group with one parameter high, and a high-risk group with both parameters high to have median survivals of 71 months, 40 months, and 15 months, respectively.250 Additional tumor-related factors predictive of inferior survival include an increased soluble IL-6 receptor level, elevated serum LDH with extramedullary disease, and increased tumor cell mitotic activity (greater than 1 per high power field).
Among the microenvironment-related factors, BM MVD has been identified as an important prognosticator. High MVD in BM (≥4 per high power field) at the diagnosis confers shorter event-free survival (EFS; 2.7 versus 4.3 years; p = 0.03) and overall survival (OS; 7.9+ versus 4.3 years; p = 0.006) after high-dose chemotherapy.251 An increased level of serum syndecan-1, as well as reduced levels of soluble CD16, have been described to portend a poor prognosis.252
Among therapy- and intervention-related prognostic factors, the type of response and length of response to prior therapy are additional risk stratification criteria; progression while on therapy and a short duration of response to prior therapy are poor risk features. The speed of response does not suggest poor overall outcomes with newer agents.
The risk stratification is applicable to newly diagnosed patients. However, the myeloma cell can acquire new changes over time, acquiring the same genomic abnormalities at relapse that are predictive of poor outcomes at diagnosis. Thus, in good-risk patients, it is necessary to evaluate for high-risk features at relapse. There is also a consensus that the high-risk features will change in the future, with the introduction of other new agents or possibly new combinations.253
An analysis of genome-wide copy number alterations (CNA) in 192 newly diagnosed, uniformly treated patients with MM using high-density SNP array suggested global genomic instability in MM.96 One of three distinct patterns of CNAs are present in 98% of cases. A multivariate analysis identified a prognostic model that includes amp(1q23.3), amp(5q31.3), and del(12p13.31) as the most powerful independent adverse markers (p <0.0001). The availability of larger scale expression profiling data in uniformly treated patient populations has provided the basis for RNA-based prognostic classification systems.92 Three large studies have evaluated the prognostic significance of gene expression profiling to identify poor-risk patient populations; the UAMS 70-gene model,93 the 15-gene IFM model,94 and the 92-gene HOVON model.95 Interestingly, these studies identified patients with a short survival, but were not designed to select patients with very good risk. It is intriguing that none of these models share common genes, highlighting the redundancy in the genes and pathways that control growth, proliferation, and survival; differences in the treatment used to define the patient population; and the complexity of tumor cell biology. These initial attempts at molecular classification and prognostication will need further validation and incorporation into more commonly available methods for larger application. Moreover, the high-risk features identified previously are highly dependent on the therapeutic intervention used. For example, the newer biologically based therapies such as lenalidomide and bortezomib are able to overcome drug resistance, and some traditional adverse prognostic factors are no longer predictive of survival. Additional molecular studies with FISH analysis, as well as proteomic and genomic analyses, including SNP, may identify future uniformly applicable prognostic systems.
The therapeutic intervention in plasma cell disorders is dependent on the presenting condition. For example, individuals with the diagnosis of MGUS do not require immediate treatment. Patients with solitary plasmacytomas can be treated with local therapy only, whereas those with indolent asymptomatic myeloma can smolder for a long period of time prior to becoming symptomatic and requiring treatment.
Solitary Plasmacytoma
Solitary plasmacytoma requires specialized techniques for accurate staging, including a CT scan and MRI to exclude more disseminated disease. Solitary plasmacytomas of the bone involve vertebral bodies in one-third of patients and frequently affect men (70%) at a younger age (median 56 years).254 A monoclonal protein in the serum is observed in 24% to 54% of patients, but no detectable monoclonal protein is observed, even on immunofixation, in the remaining cases. Extramedullary plasmacytomas are diagnosed less frequently and require a workup including MRI and PET scanning to rule out additional sites or disseminated disease. The optimal therapy for true solitary plasmacytoma is curative-dose (4,000 to 5,000 cGy) radiotherapy.255,256 With this dose, local tumor recurrence rates have been less than 10%; 30% of patients with solitary osseous plasmacytomas versus more than 70% of patients with solitary extramedullary plasmacytomas achieve long disease-free survival.257,258 Monoclonal protein disappears after radiotherapy in 25% to 50% of patients, suggesting a possible eradication of the disease; conversely, the reappearance of monoclonal protein predicts for recurrence of the disease. With better staging using MRI, true solitary plasmacytoma of bone can be cured in a high proportion of patients.
Monoclonal Gammopathy of Unknown Significance and Smoldering Myeloma
Patents with MGUS or smoldering myeloma have a low tumor mass and indolent disease course presenting without specific symptoms. Such patients do not have end organ damage. In patients with indolent light chain disease, Bence Jones proteinuria does not exceed >10 g per day. About 1% patients with MGUS per year progress to symptomatic myeloma. Non-IgG subtype, abnormal kappa/lambda free light chain ratio, and serum M protein more than 1.5 g per deciliter are associated with higher incidences of progression from MGUS to myeloma. Patients with none of these risk features have a 5% chance of progression, whereas those with all three features have a 60% chance of progression to myeloma in 20 years. The features responsible for the higher risk of progression from smoldering myeloma to active MM are bone marrow plasmacytosis >30%, abnormal kappa/lambda free light chain ratio, and serum M protein >30 g per liter (3.0 g per deciliter). Patients with all three adverse features have a nearly 50% chance of progression in 2 years.259,260 Typically, patients with MGUS require no therapy. Similarly, patients with smoldering myeloma are also not treated routinely until disease progression or the appearance of end organ damage, such as the development of bone lesions or anemia. However, ongoing clinical trials have focused on evaluating the role of early intervention to prevent the progression of smoldering to symptomatic myeloma. For example, an evaluation of thalidomide in 31 patients with indolent myeloma showed responses in 66% of patients, with the potential to delay the progression to symptomatic disease.261
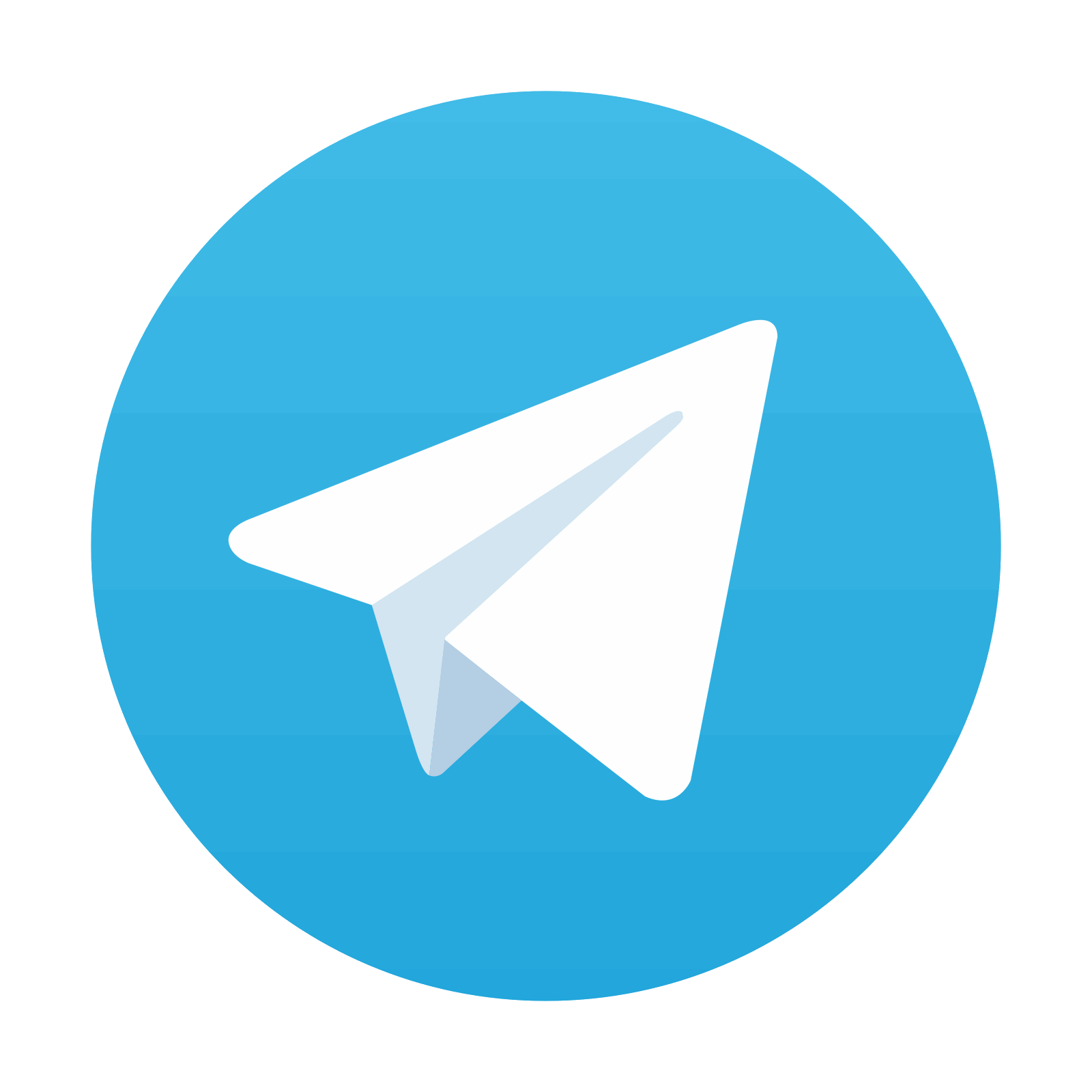
Stay updated, free articles. Join our Telegram channel

Full access? Get Clinical Tree
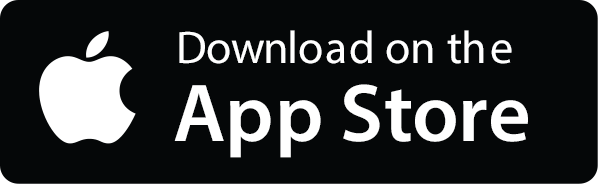
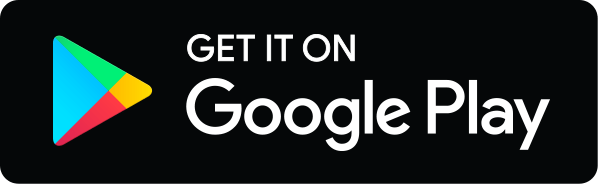