Pancreas and Islet Transplantation
Gordon C. Weir
THE PROBLEM
The devastating complications associated with both type 1 and type 2 diabetes are now clearly linked to hyperglycemia (1,2,3). The implication of these findings is that normalization of glucose levels with proper treatment early in the course of the disease would prevent the development of the microvascular and neuropathic complications and probably much of the macrovascular disease. The cost of these complications, both in personal and financial terms, is enormous, and the incidence of both forms of diabetes is increasing. Impressive improvements in treatment have been made thanks to self-glucose monitoring, advances in insulin therapy, new oral medications, and higher standards of care, but most people with diabetes continue to develop disabling complications. Although progress is being made in the development of approaches that could prevent autoimmune diabetes, the prospects for preventing type 2 diabetes seems less promising. A mechanical β-cell equivalent consisting of a glucose sensor and an insulin pump could provide patients with normoglycemia, but efforts to develop a satisfactory glucose sensor have been frustrating in spite of many ingenious approaches (4,5). The most obvious solution is to provide patients with the β-cells they are missing, which can be done with pancreas, islet, or β-cell transplants. This appealing concept even was tested clinically as early as 1893 in Bristol, England, when a physician unsuccessfully transplanted pieces of sheep pancreas into the subcutaneous space of a 15-year-old boy with diabetes (6). This conceptually simple goal continues to look like an attractive solution to the problem of diabetes but has turned out to be extraordinarily difficult to accomplish.
β-CELL REPLACEMENT AS A TREATMENT FOR TYPE 1 AND TYPE 2 DIABETES
While it is generally assumed that β-cell-replacement therapy will be useful for people with type 1 diabetes, many fail to appreciate that it could be a very effective treatment for those with type 2 diabetes, thinking that the main problem in type 2 diabetes is insulin resistance, with a deficiency in insulin secretion making only a small contribution. This misses the point that type 2 diabetes develops only when β-cells fail to compensate
for insulin resistance (7). The incidence of type 2 diabetes has skyrocketed, largely because of our sedentary Western lifestyle, with its plentiful food, which leads to central obesity with its concomitant insulin resistance. However, to understand the role of the β-cell, it must be appreciated that most people with insulin resistance never become hyperglycemic because their β-cells compensate with increased insulin secretion: hence the key role of β-cell failure in the development of diabetes.
for insulin resistance (7). The incidence of type 2 diabetes has skyrocketed, largely because of our sedentary Western lifestyle, with its plentiful food, which leads to central obesity with its concomitant insulin resistance. However, to understand the role of the β-cell, it must be appreciated that most people with insulin resistance never become hyperglycemic because their β-cells compensate with increased insulin secretion: hence the key role of β-cell failure in the development of diabetes.
People argue that a prohibitive number of islets would be required for people with type 2 diabetes and that the hyperinsulinemia would be atherogenic. In fact, many people with type 2 diabetes have insulin requirements that are not very different from those of people with type 1 diabetes, in part because of the residual insulin production in the latter. Moreover, the requirement for a large number of islets for so many patients may be solved once ways are found to make insulin-producing cells readily accessible. The health benefits of such transplants would be enormous. The microvascular and neuropathic complications of diabetes should be prevented, and there are reasons to think that the cardiovascular events would be less common. After such a transplant, an individual would still be left with obesity and insulin resistance, with their health consequences, but these would be associated with far less illness than when diabetes is superimposed. There are many approaches that could help with the problem of type 2 diabetes, such as improving insulin sensitivity with new drugs and reducing obesity, but β-cell replacement also could provide major benefits. If one follows the same arguments, β-cell replacement could prevent the hyperglycemia-related complications for almost all forms of diabetes, such as various forms of maturity-onset diabetes of the young (MODY), mitochondrial diabetes, cystic fibrosis, and diabetes secondary to pancreatectomy. In fact, these non-type 1 forms of diabetes may actually be easier targets for β-cell replacement because the transplanted islets would be encountering only allograft rejection and not autoimmunity.
PANCREAS TRANSPLANTATION
Pancreas transplants were first performed on an experimental basis in the 1960s, but the procedure was not widely applied until the mid-1980s (8,9,10,11). By the year 2000, more than 1,200 transplants were being performed yearly, with many of these being made possible by an increase in access to insurance coverage. The vast majority of these transplants have been done in the United States and Europe. Improvements in outcome have been due to advances in organ preservation, surgical techniques, and immunosuppressive drugs. The most common transplants and the best results have been obtained with simultaneous kidney/pancreas (SKP) transplants given to patients with type 1 diabetes who have advanced nephropathy. Much less common are pancreas transplants done after a kidney allograft (PAK), which usually require more immunosuppression. Decisions about pancreas transplants often are driven by the availability of a kidney from a living related donor because of the superior outcomes of such transplants compared with dialysis and even transplantation with a cadaveric kidney. Few patients receive a pancreas transplant alone (PTA), although a single center recently reported 225 of these cases (12). Justification of PTA, with its risks of mortality, morbidity, and immunosuppression, requires that patients have serious problems with their diabetes, which might include life-threatening insulin reactions, instability of control, and various psychological problems. A final, even less common, approach is the use of the distal portion of the pancreas provided by a living related donor. There continues to be debate about the efficacy of using only half of the pancreas and the risk of development of either glucose intolerance or frank diabetes in the donors (13).
The excellent results obtainable by simultaneous transplantation of a kidney and pancreas (SPK) are now being experienced by an increasing number of centers, with approximately 85% of the pancreases maintaining euglycemia in the recipient 1 year after transplantation and approximately 50% functioning well after 5 years. The euglycemia means that recipients have normal glucose levels around the clock, are without increased risk of reactive hypoglycemia (14), have normal glycohemoglobin levels, and have no dietary restrictions, even if they are taking medications such as cyclosporine or tacrolimus (FK-506) and glucocorticoids that can inhibit insulin secretion (15,16). Results reported for PAK and PTA have not been as good, but they have been improving steadily (10).
Strategies for drainage of transplanted pancreases have evolved, with most centers now using enteric drainage with a side-to-side anastomosis of the donor duodenum and the recipient ileum (17) (Fig. 45.1). Although this procedure is associated with some risk of infection, it avoids the problems that plagued the use of bladder drainage via the donor duodenum: acidosis, dehydration, infection, and a variety of other problems (18). Bladder drainage was widely used for about 10 years, but many patients who originally had bladder drainage were later converted to enteric drainage. The venous drainage from a transplanted pancreas usually goes directly into the systemic circulation, but some centers are now employing the superior mesenteric vein to allow drainage into the portal vein, which is more physiologically correct but a more technically demanding procedure (19,20).
The most commonly used immunosuppressive therapy in the past has been triple therapy with cyclosporine, azathioprine, and prednisone, but centers are now more likely to use tacrolimus (FK-506), mycophenolate mofetil, and prednisone (21). Antibodies to T cells or the interleukin-2 (IL-2) receptor are usually used during the induction phase. In general, higher doses of immunosuppressive drugs are required for patients who receive pancreas transplants than for those who receive kidney transplants alone, which is worrisome because of the increased risks of infection and malignancy (22). For SKP transplants, kidney rejection is used as a surrogate marker for rejection of the pancreas. Detection of problems with the pancreas is more difficult for PAK and PTA procedures. Some have considered amylase output a useful marker for patients with bladder drainage, but current approaches are more likely to use serum levels of amylase and lipase and pancreas biopsies (23). Immunosuppression is necessary to control not only allograft rejection but also the autoimmunity that originally caused type 1 diabetes in the recipients. The persistent autoimmunity may be especially aggressive, as indicated by SKP transplants performed between identical twins who were not given immunosuppressive medication (24). Neither the exocrine pancreas nor the kidney was rejected, but diabetes recurred, with immune destruction of the islets demonstrated by biopsy. Remarkably, this destruction occurred in only a matter of weeks, which is far more rapid than the normal progression of type 1 diabetes, which typically takes years to produce hyperglycemia (25).
Risk and Benefits of Pancreas Transplantation
Debate continues about how much patients benefit from pancreas transplants (26). This transplantation requires complex surgery and is accompanied by significant mortality and morbidity; patients frequently are hospitalized for extended periods and readmitted for problems such as intraabdominal infection and vascular thrombosis (27). Nonetheless, some studies suggest
that survival is better for patients with SKP transplants than for those with kidney transplants alone (28,29), although it is difficult to find studies with well-matched treatment groups. The impact of these transplants on the complications of diabetes seems to be modest, which is not surprising considering that so many recipients already have advanced abnormalities when they receive the transplant. Various studies have found some stabilization of retinopathy and improvement in nerve conduction velocity, but these changes seem to have little clinical impact (30,31,32). A recent study found that some histologic improvement of transplanted kidneys can take place after pancreas transplantation (33). Specifically, biopsies of kidneys of patients with PTA that were obtained 5 years after the pancreas transplant showed no benefit, but biopsies at 10 years showed impressive reversal of histologic abnormalities toward normal. A provocative study suggested that patients with autonomic neuropathy before a pancreas transplant have better survival after 7 years than those with failed grafts (34); although encouraging, this finding needs to be confirmed. The most obvious benefit of pancreas transplants is that patients consider their quality of life improved, particularly because of their freedom from insulin injections, hypoglycemic episodes, and food restrictions. It must be remembered that quality of life is a difficult parameter to evaluate (8,26). For example, it has been difficult to show, using standard parameters such as whether patients are more active or perform better at work, that their lives are improved. Nonetheless, the most striking finding, and of undeniable importance, is that patients are very happy to be free of their diabetes.
that survival is better for patients with SKP transplants than for those with kidney transplants alone (28,29), although it is difficult to find studies with well-matched treatment groups. The impact of these transplants on the complications of diabetes seems to be modest, which is not surprising considering that so many recipients already have advanced abnormalities when they receive the transplant. Various studies have found some stabilization of retinopathy and improvement in nerve conduction velocity, but these changes seem to have little clinical impact (30,31,32). A recent study found that some histologic improvement of transplanted kidneys can take place after pancreas transplantation (33). Specifically, biopsies of kidneys of patients with PTA that were obtained 5 years after the pancreas transplant showed no benefit, but biopsies at 10 years showed impressive reversal of histologic abnormalities toward normal. A provocative study suggested that patients with autonomic neuropathy before a pancreas transplant have better survival after 7 years than those with failed grafts (34); although encouraging, this finding needs to be confirmed. The most obvious benefit of pancreas transplants is that patients consider their quality of life improved, particularly because of their freedom from insulin injections, hypoglycemic episodes, and food restrictions. It must be remembered that quality of life is a difficult parameter to evaluate (8,26). For example, it has been difficult to show, using standard parameters such as whether patients are more active or perform better at work, that their lives are improved. Nonetheless, the most striking finding, and of undeniable importance, is that patients are very happy to be free of their diabetes.
Debates continue about the risk-to-benefit ratio of pancreas transplantation, particularly with a cost of approximately $100,000 per patient (26). Now that more insurance programs have agreed to cover the costs of this procedure, the demand has increased. There is no prospect for doing large controlled trials of pancreas transplantation, but new knowledge about its value will continue to emerge from smaller studies. Until a major advance occurs in islet transplantation or in the development of some kind of improved insulin delivery system, it seems likely that pancreas transplantation will continue. The use of half of a pancreas might make this form of β-cell replacement therapy available to more patients. The possibility of doing more PTAs may receive more scrutiny, particularly as a procedure for patients with early proteinuria, with its poor prognosis. As renal failure worsens in these individuals, their risk of macrovascular disease and mortality is markedly increased. The Diabetes Control and Complications Trial (DCCT) has proven that improved glycemic control is strongly protective against the development of kidney disease (1); thus, pancreas transplantation could prove valuable for patients headed toward renal failure. This possibility becomes even more attractive because of recent evidence that the progression of diabetic nephropathy might be partially reversible in patients with pancreas transplants (33).
ISLET TRANSPLANTATION
The first successful islet transplants, performed in rodents in the early 1970s (35,36), were made possible by the development of a method of isolating islets from pancreases with collagenase (37), leading to the expectation that such transplants would soon be available to everyone with type 1 diabetes. At the beginning of the 21st century, the failure to meet these expectations has been heartbreaking for countless patients, their friends and families, and the investigators struggling to progress as rapidly as possible. Indeed, there has been much debate about how science should proceed when there are so many opinions about how to balance applied versus basic research (38). The simple explanation for why the problem of islet transplantation has not been solved is that, despite the conceptual simplicity of the task, it has turned out to be extraordinarily difficult. Nonetheless, despite the frustrations and missteps, substantial progress has been made that should provide a foundation for eventual success.
Two Major Barriers to Successful Islet Transplantation
For the sake of simplicity, one can consolidate the problem of islet transplantation into two major barriers: how to provide an adequate supply of insulin-producing cells and how to prevent the processes of transplant rejection and autoimmunity from destroying these cells once transplanted.
Human Islet Allografts
Much work with small and large animal models was necessary before the first serious human islet allografts could be provided in the late 1980s to immunosuppressed patients with kidney transplants (39,40,41,42,43). These early transplants initially used islets obtained from as many as five donor pancreases, with some of these islets being cryopreserved. The islets were injected into the portal vein, using a direct approach with dissection along the umbilical vein; more recently, however, a transhepatic angiographic procedure has gained favor (Fig. 45.2). These islets become wedged in the portal tributaries and engraft, presumably receiving most of their vascular supply from host vessels growing into the islets (44). Portal hypertension, hemorrhage, or thrombosis can occur, but such complications have been rare (45). There were a few early successes, with some recipients being insulin-free for more than 2 years, but then it became apparent that most of these transplants were failures. The initial results were disappointing. Data from the International
Islet Transplantation Registry show that between 1990 and 1998, only 33 (12%) of the 267 recipients of islet allografts remained insulin-free for more than 1 week and only 8% maintained that status for over 1 year (45,46). The longest period of independence from insulin was 70 months. About 35% of these allografts had continuing graft function (C-peptide greater than 0.5 ng/mL) after 1 year. However, about 30% of the patients had a marked reduction in C-peptide levels within 1 month, a phenomenon called primary nonfunction. Because such a rapid loss is rarely seen with allografts in the absence of autoimmunity, autoimmune destruction is thought to be largely responsible.
Islet Transplantation Registry show that between 1990 and 1998, only 33 (12%) of the 267 recipients of islet allografts remained insulin-free for more than 1 week and only 8% maintained that status for over 1 year (45,46). The longest period of independence from insulin was 70 months. About 35% of these allografts had continuing graft function (C-peptide greater than 0.5 ng/mL) after 1 year. However, about 30% of the patients had a marked reduction in C-peptide levels within 1 month, a phenomenon called primary nonfunction. Because such a rapid loss is rarely seen with allografts in the absence of autoimmunity, autoimmune destruction is thought to be largely responsible.
The poor results obtained during the 1990s were very disheartening and discouraged the expansion of clinical trials. Fortunately, the few centers that persisted with transplants made advances that produced important insights (40,45,46,47,48,49,50). Although very few recipients were freed of their insulin requirements, it became apparent that the function of the grafted islets persisted in some of them, as evidenced by measurable C-peptide production. As is the case with the early stages of type 1 diabetes, residual insulin secretion greatly improved glycemic control even though insulin treatment was still required. Thus, in about 20% to 30% of carefully performed, well-documented islet transplants, C-peptide secretion did persist and appeared to lead to improved glycohemoglobin levels and fewer severe insulin reactions (47).
The Complexities of Islet Allografts
As investigators became more rigorous about details, results began to improve, although they continued to be disappointing. Better results could be obtained if the cold ischemia time of the cadaver pancreases was limited to less than 8 hours before islet isolation and if more than 6,000 islet equivalents per kilogram were transplanted (45,46). Better techniques for islet isolation have evolved. Some find that the new standardized collagenase preparation, Liberase, gives better and more consistent islet yields (51), and efforts have been made to minimize the amount of endotoxin in the reagents. The period immediately after the transplant may be critical. The phenomenon of primary nonfunction, with rapid disappearance of measurable C-peptide, is likely caused by autoimmunity, because such rapid failure virtually never occurs with autotransplants. Other complex events during the early implantation phase must lead to considerable loss of islet mass. For example, there must be some obligatory loss of cells to local hypoxia in nonvascularized clumps of islet tissue (52,53). In addition, a nonspecific inflammatory response (54) and possibly localized clotting (55) may enhance the immune processes of rejection and autoimmunity and activate the innate immune system (56). Hyperglycemia may produce increased oxygen consumption by β-cells, which would further deprive them of oxygen in their local environment, so every effort should be made to maintain euglycemia with aggressive insulin therapy. A case may be made for using intravenous insulin during the first 10 days after the transplant while new vessels from the host enter the islet grafts (44). The hyperglycemic environment appears to have an adverse influence on the outcome of islet transplants (57). There was evidence that the use of anti-thymocyte globulin during the induction period was beneficial (45,50). Some thought the use of nicotinamide, verapamil, pentoxifylline, and vitamin E was valuable (45), but the efficacy of these agents remains to be established.
Despite these partial successes, the results were far inferior to those of pancreas transplantation, which results in the immediate achievement of normoglycemia in 85% of recipients and in the remaining 15% for at least a year. This indicates that the islets contained in one pancreas should be enough and that both transplant rejection and autoimmunity can be controlled by conventional immunosuppression. Somehow, the islets contained in their normal home in the pancreas must be less vulnerable to immune injury and/or the toxic effects of the immunosuppressive agents. It is also possible that the ability of the pancreas to generate new islets from ducts may be helpful (58). Moreover, the presence of draining lymph nodes contained in the whole pancreas seems to provide a protective effect against autoimmunity (59,60).
A puzzling finding is that the mean time required for the development of insulin independence in the 33 reported successful allograft recipients was 179 ± 24 days (45). One explanation is that insulin independence tended to coincide with the lowering of the doses of immunosuppressive medications such as prednisone, which are diabetogenic, but the actual reason may be much more complex. One might not expect β-cell mass to have increased during this time, but precursor duct cells carried along with the islets (61,62) might have been the source of some new islets. Although host vessels grow into transplanted islets in only 7 to 10 days (44), we have no idea how long it takes the vessels to be fully established. In their normal location in the pancreas, islets have a specialized vasculature; arterioles break into capillaries within the core of the β-cells and then exit through the islet mantle that contains glucagon-secreting α-cells (63,64). When transplanted islets are revascularized, the normal relationship between β- and non-β-cells may not be reestablished, possibly leading to altered β-cell function (65). In addition, reinnervation (66) and topographic remodeling of the islet micro-organs may take a long time. There are other reasons for being concerned that transplanted islets may not function as efficiently as normal islets in the pancreas. It has been found that the oxygen tension of islet grafts situated under the kidney capsule of rodents is considerably lower than that for islets in the pancreas (67), a factor that could result in a reduction in glucose-induced insulin secretion.
Although we now realize that increasing the mass of islets transplanted is helpful, this was not clear in the 1990s. Islets from as many as five donors often failed, while islets from a single pancreas were sometimes sufficient. Another complex variable is islet purity; many of the successful transplants have used islet preparations that had a purity of more than 80%, and others had a purity of 50% or less, raising questions about the influence
of non-islet elements such as duct cells. The benefits of immunologic matching of donor and recipient have not been carefully studied, but most successful transplants have been matched only for blood type and not for histocompatibility antigens (45). Measurement of islet autoantibodies prior to transplantation is usually of little help because the titers are typically low, but some have found that the presence of autoantibodies may be associated with a worse outcome (68,69). An approach that may be worth pursuing is the measurement of gene expression of various immune mediators in circulating lymphocytes during the course of transplantation, as this has been found to correlate with kidney allograft rejection (70).
of non-islet elements such as duct cells. The benefits of immunologic matching of donor and recipient have not been carefully studied, but most successful transplants have been matched only for blood type and not for histocompatibility antigens (45). Measurement of islet autoantibodies prior to transplantation is usually of little help because the titers are typically low, but some have found that the presence of autoantibodies may be associated with a worse outcome (68,69). An approach that may be worth pursuing is the measurement of gene expression of various immune mediators in circulating lymphocytes during the course of transplantation, as this has been found to correlate with kidney allograft rejection (70).
The Edmonton Protocol
Frustrated by the mediocre results of earlier trials, a group of investigators in Edmonton, Alberta, Canada, tried a new approach in 1999 (71). They thought that rapamycin (sirolimus) might be beneficial and that glucocorticoids were toxic to islets. They also did everything possible to improve the quality of their islet preparations and reasoned that more islets would be required than was previously considered necessary. They recruited patients with type 1 diabetes who had serious problems with hypoglycemia, which justified the use of potentially dangerous immunosuppressive agents. In contrast to the procedure for earlier transplants, the islets were used immediately after their isolation rather than being maintained in tissue culture. The immunosuppression regimen included rapamycin (sirolimus) and tacrolimus (FK-506) but no prednisone. For induction, antibody to the IL-2 receptor (daclizumab) was used. Islets were introduced into the liver through the portal vein via transhepatic angiography. Islets from more than one cadaver donor were required, with two sufficing for most of the patients. Normoglycemia was never attained after the first transplant but did occur immediately after the second or third transplant. A total of about 11,000 islet equivalents (IE) per kilogram were used. At the time of this writing, 17 patients have been rendered insulin-free with glycohemoglobin levels in the normal or near normal range. Most of the patients have glucose intolerance, and their stimulated C-peptide release is approximately one third of normal, which suggests that their β-cell mass is marginal or that the grafted β-cells are less efficient than pancreatic β-cells. Some of the patients have maintained normal glycohemoglobin levels without insulin for almost 2 years.
The results of the Edmonton group are spectacularly better than any previous results, but this enthusiasm must be tempered by the reality that patients must receive dangerous immunosuppressive therapy and that two or more cadaver pancreases are required, which means that very few patients will be treated. Nonetheless, this is a true advance that should provide a foundation for future improvements with different protocols. To bring other groups up to the same level, the Immune Tolerance Network funded by the National Institutes of Health, with help from the Juvenile Diabetes Foundation, is funding 10 centers to perform 40 transplants to try to reproduce the Edmonton results. As with the Edmonton trial, the multicenter trial will enroll patients without kidney transplants who have threatening episodes of hypoglycemia; however, patients with severe instability of their control and advancing complications will also be considered.
Allografts in the Absence of Autoimmunity
Insight into the possible problems caused by autoimmunity have been provided by cluster operations for abdominal cancer in which the liver, pancreas, and other organs were removed from the patient, who then received a cadaver liver into which islets isolated from the same cadaver donor were placed, providing a pure allograft situation (46). These islet allografts more often produced normoglycemia than did islets given to patients with type 1 diabetes; patients became insulin-independent 60% of the time, and one patient was insulin-free for 5 years. Although transplantation of the liver may have had some beneficial influence on the immune system, the absence of autoimmunity is suspected to be a major reason for the success. In addition, these recipients are typically unhealthy, so they may require less β-cell mass to accommodate their reduced nutritional intake and weight.
Islet Autografts
Transplantation of islets that do not face immune attack provides important lessons about how well islets can perform in the liver. When pancreases are removed because of painful pancreatitis or some other reason, it is often possible to isolate the islets and transplant them into the liver via the portal vein. These digested pancreatic preparations have typically been relatively impure, containing non-islet pancreatic elements, including duct cells that have the capacity for neogenesis. These patients do remarkably well, with about 70% being insulin-independent at 1 year if they receive more than 300,000 IE (46). Sometimes success can be achieved with even fewer than 200,000 islets—considerably fewer than the usual requirement for successful allografts (72,73). The most obvious explanation for success is that there are no problems with either allorejection or autoimmunity; it must also be remembered, however, that the removal of glucagon by the pancreatectomy and the tendency of these patients to be thin could make them relatively insulin-sensitive.
EFFORTS TO CONTROL TRANSPLANT REJECTION AND AUTOIMMUNITY
Current organ transplants are successful because of immunosuppression, which although more effective than ever, continues to be associated with notable risk, the main threats being susceptibility to infection and the development of malignancy (22). There is great reluctance to use such dangerous drugs in people with type 1 diabetes whose prognosis might otherwise be good. Fortunately, considerable progress is being made in the quest for safer and more effective immunosuppressive drugs. As we learn more about the differences between rejection and autoimmunity, we can expect different approaches to be used for each process. Even different drugs are likely to be required for xenograft rejection.
Induction of Tolerance
A major goal for the transplantation field is to be able to induce tolerance, that is, treatment given only at the time of transplantation will somehow trick the recipient’s immune system into accepting transplanted foreign tissue as his or her own. Many different approaches currently being studied could lead to full or operational tolerance (Table 45.1). Tolerance to transplanted tissue has been induced by a variety of techniques in a number of experimental models (74), but many scientific and safety hurdles must be overcome before such approaches can be used in humans. One hopeful example was the islet transplants between different species of nonhuman primates in which anti-CD3 immunotoxin, cyclosporine, and steroids were given only during the peritransplant period, with normoglycemia persisting for more than 100 days in the absence of further immunosuppression (75).
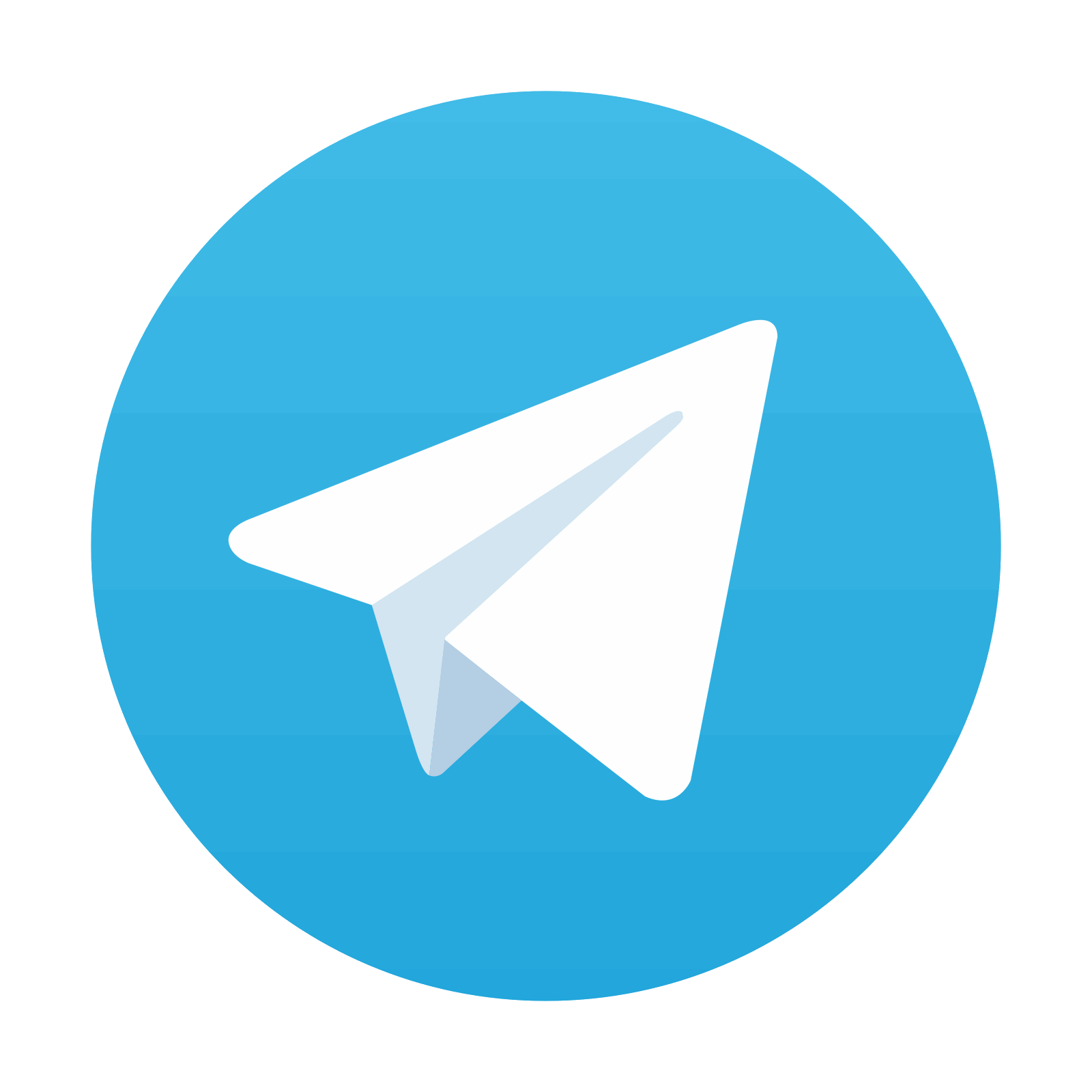
Stay updated, free articles. Join our Telegram channel

Full access? Get Clinical Tree
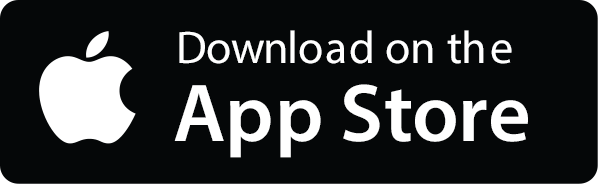
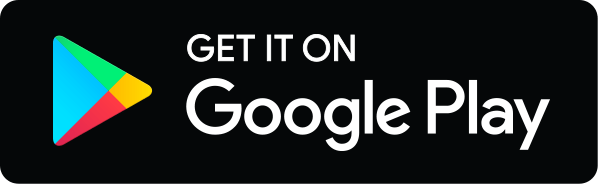