2 University of Southampton, UK
- The immune system may become activated by microbial invasion, as well as by a wide range of stimuli and conditions. The immune response exerts a high metabolic and nutritional cost upon the body.
- Nutrition has a two-way influence on the immune system. The activities of the immune system exert a deleterious influence on nutritional status and alterations in nutrient intake modulate the intensity of the various activities of the immune system.
- Proinflammatory cytokines have far-reaching metabolic effects throughout the body, including changes in protein, fat, vitamin, and trace-element metabolism, alteration of body temperature and appetite, and changes in liver protein synthesis.
- An individual’s nutritional status and intake of specific nutrients can modify cytokine biology in ways which have major implications for health and well-being.
- Antioxidants may suppress inflammatory components of the response to infection and trauma, and enhance components related to cell-mediated immunity.
- The unsaturated fatty acid and cholesterol content of the diet also plays a role in the inflammatory response. While n-6 polyunsaturated fatty acids (PUFAs) and cholesterol exert a proinflammatory influence, n-3 PUFAs and monounsaturated fatty acids exert the opposite effect.
- Single base changes (single-nucleotide polymorphisms, SNPs) in the promotor region of cytokine genes raise, or lower, the amount of cytokines produced during the inflammatory response. This phenomenon has been shown in many studies to increase mortality and morbidity during infection and trauma.
- SNPs interact with nutrient status during the inflammatory response, altering the efficacy of immunonutrients. Further knowledge in this area will pave the way to personalised clinical nutrition.
17.1 Introduction
Humans live in the presence of many types of microorganism, which exert pathological effects if they succeed in penetrating the surface defences of the body. Once entry is gained, rapid multiplication occurs, which, if unchecked, can end in death. However, we possess an immune system that has a great capacity for immobilising invading microbes, creating a hostile environment for them, and bringing about their destruction. Humans and warm-blooded animals have survived because their immune systems have the ability to focus a range of lethal activities upon the invader. This biological property is important because many microbes can multiply at least 50 times faster than the cells of the system. The immune system must therefore become rapidly effective once invasion has occurred. The immune system can also become activated, in a similar way to the response to microbial invasion, by a wide range of stimuli and conditions; these include burns, penetrating and blunt injury, the presence of tumour cells, environmental pollutants, radiation, exposure to allergens, and the presence of chronic inflammatory diseases. This latter group of stimulatory conditions includes such diseases as rheumatoid arthritis, Crohn’s disease, asthma, and psoriasis, as well as more common conditions such as atherosclerotic heart disease, obesity, diabetes, and Alzheimer’s disease. The strength of the response to this disparate range of stimuli may vary, of course, but it will contain many of the hallmarks of the response to invading pathogens. In the normal response to perturbation, the immune system goes from a state of activation to one of deactivation as the body becomes repaired from the effects of the invasion. However, in chronic inflammatory disease the initial activation continues unabated.
As will be seen later, the immune response exerts a high metabolic and nutritional cost upon the body. Inappropriate prolongation of the response will have a deleterious effect upon the nutritional status of the patient.
17.2 The response of the immune system to activation
The immune system is located throughout the body. It consists of clearly recognised structures, such as the spleen, thymus, and lymph nodes, and diffuse populations of cells. Examples of the latter component of the system are lymphocytes, which circulate around the body via the bloodstream and lymphatic circulation. In addition, macrophages (cells capable of engulfing foreign particles, bacteria, viruses, and fungi) populate the linings of the lungs, and together with lymphocytes occupy areas deep in the walls of the small and large intestine. There is also a network of immune cells (dendritic cells) within the skin, which form an important part of the overall immune defence. Thus virtually every part of the body comes under the vigilance of the immune system.
In general terms, the reaction of the immune system to activation can be divided into two components: an innate response, which is unaffected by whether the subject has encountered a particular pathogen before, but is rapid, nonspecific, and the main mediator of the inflammatory response; and a specific immune response, which ‘remembers’ a previous encounter with a pathogen and produces a much-enhanced focused response on each subsequent exposure (Figure 17.1).
The specific immune response is further subdivided into a cell-mediated and a humoral response. The former involves T lymphocytes, which originate in the bone marrow and undergo further development in the thymus. The second involves B lymphocytes, which originate in the bone marrow and, when stimulated with molecules that are foreign to the body (antigens), develop into cells capable of producing antibodies (immunoglobulins, Igs). These are highly specific to the antigen and aid in its destruction.
While potentially thousands of discretely different Igs can be produced (one for each antigenic substance/organism that might be encountered by the body), they fall into five main classes, depending upon their gross structure. The classes are labelled G, M, A, D, and E. For example, IgA has the ability to cross cell-epithelial cell layers and is thus found in tears, saliva, gut secretions, and milk. IgE, on the other hand, has the ability to attach to mast cells, and when activated leads to the release of histamine and other chemicals associated with allergy.
B cells also come under the influence of a type of T cell, called the helper T cell. Both these forms of lymphocytes and macrophages secrete a range of proteins called cytokines, which act as the ‘hormones of the immune system’. Within this group are the interleukins (ILs), tumour necrosis factors (TNFs), and interferons (IFNs). The cytokines act in an apocrine, paracrine, and endocrine manner and modify many activities of the immune system (Table 17.1).
In addition to their direct influence on immune cells, the cytokines IL-1, IL-6, and TNF have widespread metabolic effects upon the body and stimulate the process of inflammation. These cytokines are thus subclassified as proinflammatory cytokines. Key features of the effects of this group of molecules are shown in Figure 17.2.
Nutrition has a two-way influence on the immune system. The activities of the immune system exert a deleterious influence on nutritional status and alterations in nutrient intake modulate the intensity of the various activities of the immune system. Experimental studies and clinical observation have shown that many aspects of the immune response can be modified by alteration in the intake of protein, specific amino acids, lipids, and micronutrients.
Table 17.1 Some examples of cytokines and their effects.
Cytokine | Major effects |
Interleukin 1a | Fever, muscle protein loss, raised blood glucose, changes in blood trace-element concentration |
Tumour necrosis factor-alphaa | Fever, appetite loss, muscle protein loss, raised blood lipids, changes in blood trace-element concentration, stimulates oxidant production |
Interleukin 6a | Stimulates acute-phase protein production by the liver |
Interleukin 2 | Stimulates T-lymphocyte proliferation |
Interleukin 8 | Causes attraction of immune cells: chemotaxis |
Interleukin 10 | Inhibits proinflammatory cytokine production |
a There are varying degrees of overlap between the actions of these proinflammatory cytokines.
17.3 The effects of proinflammatory cytokines
Many of the signs and symptoms experienced after infection has occurred, such as fever, loss of appetite, weight loss, negative nitrogen and mineral balance, and lethargy, are caused directly and indirectly by proinflammatory cytokines (Figure 17.2). The indirect effects of cytokines are mediated by actions upon the adrenal glands and endocrine pancreas, resulting in increased secretions of the catabolic hormones epinephrine (adrenaline), norepinephrine (noradrenaline), glucococorticoids, and glucagon. Insulin insensitivity occurs in addition to a ‘catabolic state’. Reduced insulin sensitivity leads to hyperglycaemia in all but the mildest inflammatory responses. Although increased blood glucose levels do provide greater energy supply to immune cells, fibroblasts, and the brain, which may assist the systemic inflammatory response, hyperglycaemia, particularly if severe, increases morbidity and mortality in the critically ill.
The diverse range of metabolic changes caused by the proinflammatory cytokines can be seen as a coordinated response (Figure 17.3) designed to:
- create a hostile environment for the invading organism;
- provide nutrients, from within the body, to support the actions of the immune system;
- enhance the defence systems of the body to protect healthy tissue from the potent actions of the inflammatory response.
Changes in protein and fat metabolism
The biochemistry of an infected individual is thus fundamentally changed in a way that will ensure that the immune system receives nutrients from within the body to perform its tasks. Muscle protein is catabolised to provide amino acids for the synthesis of new cells and proteins for the immune response. Furthermore, amino acids are converted to glucose (a preferred fuel for the immune system). The extent of this process is highlighted by the major increase in urinary urea excretion, ranging from 9 g/day in mild infection to 20–30 g/day following major burn or severe traumatic injury. Fat is catabolised at an accelerated rate and the fatty acids released help to satisfy the increased energy needs of the infected person (the resting metabolic rate increases by 13% for every 1 °C rise in body temperature).
Alteration of body temperature and appetite
The rise in temperature is part of the body’s attempt to create a hostile environment for the invader. In past centuries, physicians often advocated steam baths for the treatment of infection. It is also interesting to note that when infected, cold-blooded animals, which are unable to raise their body temperature by endogenous means, often move to hotter areas of their immediate environment in an attempt to create hostile conditions for invading organisms.
In mammals, the changes in temperature are mediated by the interaction of proinflammatory cytokines with specialised neurons in the hypothalamus. There is some debate over whether the cytokines gain access directly to the hypothalamus by crossing the blood–brain barrier, or whether circulating cytokines are excluded by the barrier but induce cytokine production within the hypothalamus. The interaction of cytokines with the hypothalamus also brings about a loss of appetite. This may be transient in nature, or prolonged and profound, as is the case in chronic infections such as tuberculosis or during cancer. It is interesting to note that the body-fat regulatory hormone leptin is induced by TNF, but as yet no direct role for leptin in the significant weight loss during infection and cancer has been found.
Changes in trace-element and vitamin metabolism
Major changes occur in plasma cation concentration, such as of iron, copper, zinc, and selenium. These changes in iron and zinc are often misinterpreted as indicating mineral deficiency, but are very likely to be due to a major redistribution of these elements within the body in an attempt to ‘starve’ bloodborne microbes of nutrients or to foster certain beneficial aspects of the systemic inflammatory response in specific organs. However, micronutrient deficiency can be precipitated by the response to infection, as urinary loss of many micronutrients is accelerated following infection and injury. The resultant deficiencies in zinc, iron, and copper have deleterious effects on general immune function and wound healing. The cytokines also stimulate the synthesis of potent oxidant molecules (hydrogen peroxide, nitric oxide, hydroxyl radical, hypochlorous acid, and superoxide anion), which damage the cellular integrity of the invading organism. A similar lowering of certain vitamin or provitamin levels in the serum or plasma, like fat-soluble vitamins A, D, and E and water-soluble vitamins in the B and C series, also occurs in response to systemic inflammation, which is of uncertain significance but does make the diagnosis of deficiency more difficult and uncertain.
Changes in liver protein synthesis
Specialised proteins, called acute-phase proteins, which focus the actions of the immune system on the invader and help to protect healthy tissue from ‘collateral damage’ in the ‘battle’ with pathogens, are synthesised in increased amounts by the liver. Important acute-phase proteins and their respective functions are highlighted in Table 17.2. The liver focuses its activities on acute-phase protein synthesis by slowing the synthesis of its main protein product, serum albumin, and of a number of other secretory proteins, such as prealbumin, retinol-binding protein, and transferrin. This latter group of proteins is termed the ‘negative acute-phase reactants’.
Serum albumin concentration is often regarded as an index of protein nutritional status. Thus, the fall in albumin concentration that occurs during infection and inflammatory disease is often misunderstood as a sign of protein deficiency and complicates the assessment of protein status in patients. In virtually every instance, a low serum albumin concentration clinically reflects the presence, or recent occurrence, of a systemic inflammatory response. The response produces malnutrition by inducing anorexia, decreasing voluntary activity, and reducing the metabolic efficiency of endogenous and exogenous protein utilisation. However, low serum albumin concentration is not specifically a measure of nutritional status, since only an unattainably low protein intake, in conjunction with an adequate energy intake, will even modestly affect serum albumin concentrations. For instance, patients with very severe anorexia nervosa, with losses of body weight in excess of 30%, will have normal concentrations of serum albumin.
Table 17.2 Biological functions of major acute-phase proteins.
Function | Acute-phase proteins |
Inhibition of proteinase released during the inflammatory response | Alpha-1 proteinase inhibitor Alpha-1 antichymotrypsin Alpha-2 macroglobulin |
Removal of antigens from the host | C-reactive protein Serum amyloid A |
Activation of the immune response | C-reactive protein C3 complement |
Suppression of the immune response | Proteinase inhibitors Alpha-1 glycoprotein |
Antioxidant properties | Ceruloplasmin Alpha-1 acid glycoprotein |
Binding and transport of metals and biologically active compounds | Ceruloplasmin Haptoglobin Transferrin Alpha-1 acid glycoprotein |
Many of these complex metabolic changes result in the balance being swung from being in favour of the invading organism to being in favour of the infected individual.
17.4 Control systems for cytokines
Proinflammatory cytokines also induce a cascade of production of other cytokines from lymphocytes. The cascade has modulating actions on lymphocyte function (IL-2 stimulates lymphocyte proliferation, IL-8 attracts immune cells to the site of invasion, IL-4 alters the class of antibodies produced). It is of interest to note that cytokines are also capable of autoregulation: IL-10 with IL-4, produced in response to proinflammatory cytokines, suppresses production of further proinflammatory cytokines.
Conceptually, there is evidence that there can be a poor outcome from an excessive systemic inflammatory response. This is referred to as the systemic inflammatory response syndrome (SIRS) and is manifested by increased TNF concentrations in sepsis or following burn injury. However, there is a compensatory anti-inflammatory response syndrome (CARS), reflected in increased production of IL-4 and IL-10, which, if excessive, can also have a deleterious outcome. It has been hypothesised, from a growing body of research data, that the relative balance between SIRS and CARS has an impact on survival. This is probably why attempts to block proinflammatory cytokine production with monoclonal antibodies have met with limited success in reducing mortality.
Nutritional modulation that affects both sides of the SIRS/CARS equation offers a greater promise of success. A class of proteins called heat-shock proteins (HSPs), which were originally identified in heat-stressed cells, are also produced by cells exposed to proinflammatory cytokines. HSPs have a general anti-inflammatory influence and suppress cytokine production. These autoregulatory mechanisms are very important biological phenomena, because, while cytokines are essential for effective operation of the immune system, they exert a high metabolic cost on the body and can exert damaging and lethal effects (Figure 17.3). Conceptually, the systemic inflammatory response should be viewed as a general benefit in most instances, but as having the potential for harm through excessive or prolonged activation of SIRS or CARS. These adverse impacts can result from excessive inflammation, immunodepression, and/or the development of protein-energy malnutrition.
17.5 Damaging and life-threatening effects of cytokines
Although cytokines are essential for the normal operation of the immune system when produced at the right time and in the right amounts, they play a major damaging role in many inflammatory diseases, such as rheumatoid arthritis, inflammatory bowel disease, asthma, psoriasis, and multiple sclerosis, and in cancer. They are also thought to be important in the development of atheromatous plaques in cardiovascular disease. In conditions such as cerebral malaria, meningitis, and sepsis, they are produced in excessive amounts and are an important factor in increased mortality. In these diseases, the cytokines are being produced in the wrong biological context.
The end stages of many chronic conditions have, as a root cause, an ongoing systemic inflammatory response, which is documented by either elevated plasma cytokines, their soluble receptors, IL-1 receptor antagonist, IL-6, spontaneous or stimulated release of cytokines from peripheral blood mononuclear cells (PBMCs), or elevated acute-phase protein concentrations, particularly C-reactive protein (CRP). These conditions include end-stage liver disease (TNF), end-stage renal disease (IL-6, CRP, soluble cytokine receptors), congestive heart failure (TNF), weight-losing chronic obstructive pulmonary disease (COPD) (IL-6); elevated CRP concentrations are also found in poorly controlled diabetes and obesity. Furthermore, concentrations of this acute-phase protein, when measured by high-sensitivity assay, have been associated with higher risk of coronary or cerebrovascular disease.
Adverse effects of an individual’s genotype
It has recently become apparent that single base changes (single-nucleotide polymorphisms, SNPs), usually in the promoter region of genes responsible for producing the molecules involved in the inflammatory process, exert a modulatory effect on the intensity of inflammation. In vitro production of TNFα by PBMCs from healthy and diseased subjects stimulated with inflammatory agents shows remarkable individual constancy in men and postmenopausal women. This constancy suggests that genetic factors exert a strong influence. A number of studies have shown that SNPs in the promoter regions for the TNFα and lymphotoxin alpha (LTα) genes are associated with differential TNFα production. The TNF2(A) and TNFB2(A) alleles (at −308 and +252 for the TNFα and LTα genes, respectively) are linked to high TNF production, particularly in homozygous individuals.
A large body of research has indicated that SNPs occur in the upstream regulatory (promoter) regions of many cytokine genes (e.g. IL-6 −174, IL-1β −511). Many of these genetic variations influence the level of expression of genes and the outcome from the inflammatory response. Both pro- and anti-inflammatory cytokines are influenced by the differences in genotype.
In a study on inflammatory lung disease, caused by exposure to coal dust, the TNF2 (LTα +252A) allele was almost twice as common in miners with the disease as in those who were healthy. Development of farmer’s lung, from exposure to hay dust, was 80% greater in individuals with the TNF2 allele than in those without the allele. The TNF2 allele was also twice as common in smokers who developed COPD as in those who remained disease-free.
In addition to disease progression, genetic factors have important effects on mortality and morbidity in infectious and inflammatory disease. In a study on malaria, children who were homozygous for TNF2 had a sevenfold increase in the risk of death or serious pathology compared to children who were homozygous for the TNF1 allele. In sepsis, patients possessing the TNF2 allele had a 3.7-fold greater risk of death than those without the allele, and patients who were homozygous for the LTα +252A allele had twice the mortality rate of and higher peak plasma TNFα concentrations than heterozygotic individuals. The TNF2 allele has also been found in increased frequencies in systemic lupus erythromatosus, dermatitis herpetiformis, and insulin-dependent and non-insulin-dependent diabetes mellitus.
Genetic factors also influence the propensity of individuals to produce oxidant molecules and thereby influence nuclear transcription factor kappa B (NFκB) activation. Natural resistance-associated macrophage protein 1 (NRAMP1) has effects on macrophage functions, including TNFα production and activation of inducible nitric oxide synthase (iNOS), which occurs through cooperation between the NRAMP1, TNFα, and LTα genes. There are four variations in the NRAMP1 gene, resulting in different basal levels of activity and differential sensitivity to stimulation by inflammatory agents. Alleles 1, 2, and 4 are poor promoters, while allele 3 causes high gene expression. Hyperactivity of macrophages, associated with allele 3, is linked to autoimmune disease susceptibility and high resistance to infection, while allele 2 increases susceptibility to infection and protects against autoimmune disease.
SNPs also occur in genes for anti-inflammatory cytokines. There are at least three polymorphic sites (−1082, −819, −592) in the IL-10 promoter which influence production. In intensive-care patients, the occurrence of the 1082*G allele, which produces IL-10, was high in those who developed multiorgan failure, with a frequency one-fifth of that of the normal population.
Thus it now appears that each individual possesses combinations of SNPs in their genes, associated with inflammation corresponding to ‘inflammatory drives’ of differing intensities when microbes or tissue injury are encountered. At an individual level, this may express itself as differing degrees of morbidity and mortality.
In general, men are more sensitive to the genomic influences on the strength of the inflammatory process than women. In a study on LTα genotype and mortality from sepsis, it was found that men possessing a TNFB22 (LTα +252AA) genotype had a mortality of 72%, compared with men who were TNFB11 (LTα +252GG), who had a 42% mortality rate. In female patients, the mortalities for the two genotypes were 53 and 33% respectively. In a study on patients undergoing surgery for gastrointestinal cancer, it was found that postoperative CRP and IL-6 concentrations were higher in men than in women. Multivariate analysis showed that men possessing the TNF2 (TNFα −308A) allele had greater responses than men without it. The genomic influence was not seen in women. Furthermore, possession of the IL-1 −511 T allele was associated with a greater length of stay in hospital in old men admitted for geriatric care. Women were unaffected by these genetic influences.
Many studies have shown a clear link between obesity, oxidant stress, and inflammation. The link may lie in the ability of adipose tissue to produce proinflammatory cytokines, particularly TNFα. There is a positive relationship between adiposity and TNF production. Leptin has also been shown to influence proinflammatory cytokine production. Thus plasma triglycerides, body fat mass, and inflammation may be loosely associated because of these endocrine relationships.
In an investigation of cytokine production in healthy men, one of the authors found that, in the study population as a whole, there were no statistically significant relationships between body mass index (BMI), plasma fasting triglycerides, and the ability of PBMCs to produce TNFα. However, individuals with the LTα +252AA genotype (associated with raised TNF production) showed significant relationships between TNF production and BMI and fasting triglycerides. Thus, despite the study population comprising only healthy subjects, within that population were individuals with a genotype that resulted in an ‘aged’ phenotype as far as plasma lipids, BMI, and inflammation were concerned.
Adverse effects of proinflammatory cytokines on body composition
Experience worldwide shows that infections are a potent inhibitor of growth. The actions of IL-1, IL-6, and TNF exert a high metabolic cost on the body. Substrate released from muscle, skin, and bone under their influence will undoubtedly nourish the immune system and help wound healing; however, in a growing child a conflict of interests occurs and nutrients will be diverted away from growth. The metabolic cost of cytokine action also has potentially deleterious effects in adults. In malaria, tuberculosis, sepsis, cancer, human immunodeficiency virus (HIV) infection, and rheumatoid arthritis, the cytokines bring about a loss of lean tissue, which can seriously debilitate an individual. It is a well-known phenomenon that infection is accompanied by a raised body temperature and an increased output of nitrogenous excretion products in the urine. The loss of nitrogen from the body of an adult during a bacterial infection may be equivalent to 60 g of tissue protein, and in a period of persistent malarial infection, over 500 g of protein.
There are four clinical conditions that cause maximal protein catabolism: (i) major third-degree burns of more than 30% of body surface area; (ii) multiple trauma; (ii) closed head injury with a low Glasgow Coma Score; and (iv) severe sepsis. These result in losses of nitrogen of up to 30 g/day, which is equivalent to 200 g tissue protein. The latter translates into losses of 900 g lean tissue/day, at the usual conversion figure of 30 : 1 for lean tissue to grams of nitrogen.
Important endocrine changes occur during the systemic inflammatory response, which impact upon growth and tissue composition. There is a reduction in anabolic stimuli for growth, including a reduction in testosterone and insulin resistance. Despite increases in growth hormone, which enhances fat mobilisation and gluconeogenesis, production of insulin-like growth factor 1 (IGF-1), which is responsible for the growth-promoting actions of growth hormone, is not promoted. Furthermore, insulin resistance in carbohydrate metabolism fosters an increase in hepatic glucose production and a reduced uptake by skeletal muscle.
These changes, which at first sight appear detrimental, may be beneficial during the systemic inflammatory response, primarily because they result in an increase in blood glucose. Glucose is an ideal metabolic fuel because, having no charge and a small size, it is easily diffusible; further, it can be oxidised to easily excreted products (carbon dioxide and water), or it can uniquely be metabolised to produce ATP without a requirement for oxygen by anaerobic glycolysis. This latter process is particularly important in ischaemic tissues and in macrophages and fibroblasts, which are facultative anaerobes.
Other beneficial results of the systemic inflammatory response are the release of glutamine from muscle, which is important for cells of the immune system and other rapidly dividing cells, and the creation of ammonium ions from deamination of glutamine, which assist in acid–base balance.
Potential damage from the interaction of oxidants with cytokine production
There are further characteristics of cytokine biology which can damage an infected individual indirectly. The oxidant molecules produced by the immune system to kill invading organisms can also activate the important cellular control molecule NFκB. This factor is a control switch for biological processes, not all of which are advantageous to the individual. Activated NFκB migrates to the nucleus, where it switches on genes for cytokine, glutathione, and acute-phase protein production. Unfortunately, however, it also increases HIV replication. This sequence of events accounts for the ability of minor infections to speed the progression of individuals who are infected with HIV towards the acquired immune deficiency syndrome (AIDS) (Figure 17.4).
In the last decade it has become increasingly clear that an individual’s nutritional status and intake of specific nutrients can modify cytokine biology in ways that have major implications for health and well-being.
17.6 Influence of malnutrition on key aspects of the cytokine response
Malnutrition has a major effect upon the normal operation of the immune and inflammatory components of the immune system. In a sense, malnutrition was the first-known ‘acquired immune deficiency’ disease; the effects of malnutrition have much in common with the later-recognised AIDS due to HIV infection. Malnutrition influences virtually all of the components of the immune response. The most obvious ones are a reduction in the cell number and function of the lymphocytes associated with cell-mediated immunity, shrinkage or impaired development of the organs of the immune system (thymus, spleen, and gut-associated lymphoid tissue), and suppression of some aspects of the inflammatory response.
Figure 17.4 The effect of inflammatory stimuli on cell biology via activation of transcription factors. AP1, activator protein 1; NFκB, nuclear transcription factor kappa B; IL, interleukin; GSH, reduced form of glutathione; LPS, lipopolysaccharide; HIV, human immunodeficiency virus; IL, interleukin; TNF, tumour necrosis factor.
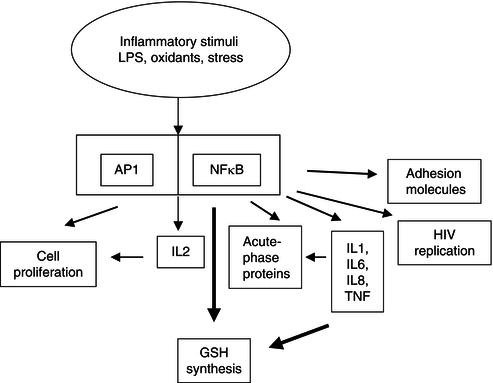
Influence of protein-energy malnutrition
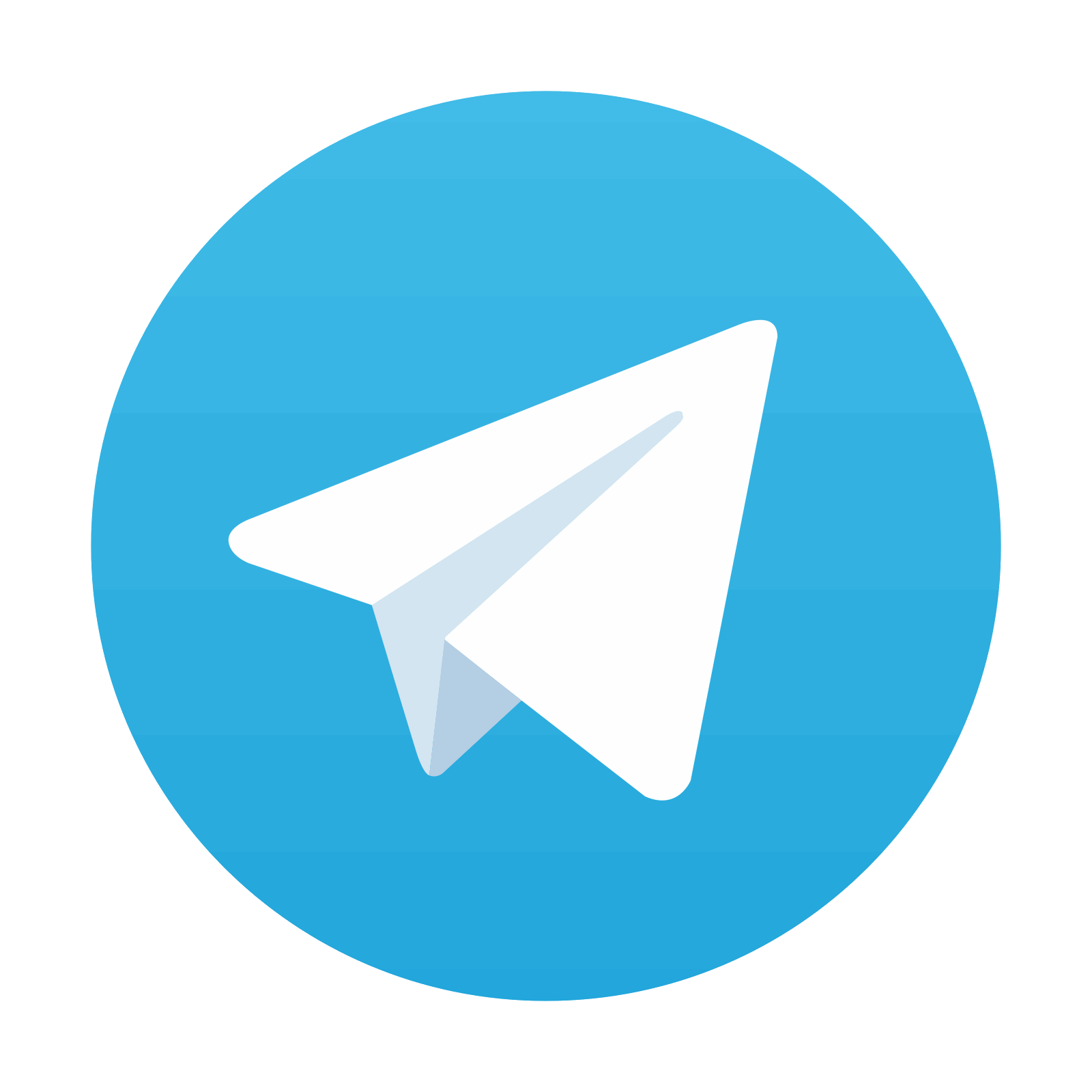
Stay updated, free articles. Join our Telegram channel

Full access? Get Clinical Tree
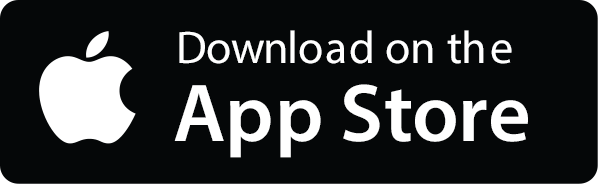
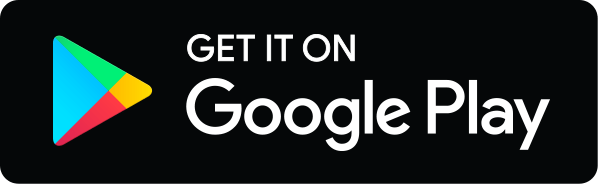