2Touro University, California USA
- The prevalence of noncommunicable diseases such as obesity, diabetes, dyslipidaemia, cardiovascular diseases, nonalcoholic fatty liver disease (NAFLD), and cancer has increased substantially in European and North American populations.
- A similar trend is now being observed in many countries within Africa and South-East Asia, and also in China, which appears to be related to improvements in average income and industrialisation/urbanisation.
- Morbidity is positively correlated with body mass index (BMI), even within the normal range of 20–25 kg/m2. The hypothesis that a low energy intake is associated with an increased lifespan is supported by animal studies.
- Energy restriction is associated with increased lifespan and lower morbidity in animal models and in humans. Proposed mechanisms are decreased oxidative stress, improved DNA repair, and lower lipotoxicity due to sirtuins activation.
- The concomitant occurrence of visceral obesity, impaired glucose tolerance or type 2 diabetes, hypertension, and dyslipidaemia is known as the ‘metabolic syndrome’. Insulin resistance and an excess of visceral fat appear to play a pathogenic role in each of these conditions.
- These metabolic disorders, or diseases of affluence, can be attributed not only to dietary factors but also to decreased physical activity and other environmental factors. It is also likely that the genetic background of individuals plays an important role.
6.1 Introduction
The average life expectancy of European and North American populations increased drastically during the second half of the twentieth century. This is essentially attributable to a marked reduction of the mortality associated with infectious diseases. In contrast, the prevalence and mortality associated with noncommunicable diseases, such as cardiovascular diseases, cancer, diabetes, and obesity, have increased substantially. A similar trend is currently evident in many countries within Africa and South-East Asia, and also in China, which appears to be related to improvements in average income and industrialisation/urbanisation. This epidemiological evolution suggests that the development of these diseases is strongly dependent on the way of life of affluent societies. This may stop or even reverse the trend of increased life expectancy in some countries, because of the years of life lost by individuals as a result of obesity.
The wealth of a nation impacts indisputably on the way of life of its population. As a result of industrialisation, individuals switch from physically active, rural occupations to more sedentary factory and office jobs. The higher income associated with these jobs also promotes the use of automated housework devices, cars, and so on, which leads to a substantial reduction in physical-activity levels. Simultaneously, the mode of feeding and the types of macronutrient consumed are altered as a consequence of urban living. This includes changes in market food availability and increases in consumption of fast food and meals in restaurants. The urban feeding pattern is associated with an increased consumption of processed foods with a higher proportion of fat and sugar when compared with traditional, rural diets containing high-fibre/high-complex carbohydrate foods. These changes in lifestyle are thought to be involved in the pathogenesis of nontransmissible diseases. For example, obesity has dramatically increased over the past century. While rare at the beginning of the twentieth century, it now affects 20–50% of European and North American populations. The marked increase in childhood obesity over the past two or three decades, together with the growing occurrence of type 2 diabetes mellitus in children and young adults, is of particular concern.
The development of obesity stems from an imbalance between energy intake and energy expenditure. There is evidence that energy density, portion size, and the frequency of eating/drinking occasions are contributing to a significant increase in the amount of food calories consumed per capita. From 1977 to 2006, calorie consumption is estimated to have increased by 570 kcal/day in the USA. Other studies clearly indicate that the amount of energy expended through physical activity has dropped during this period. Based on measurements of energy fluxes, it appears that a high energy intake and a low energy expenditure both play a role in the development of obesity.
In addition to nutritional changes and alterations in physical activity, other factors also affect the pattern of diseases in affluent societies. For instance, there is an important increase in the consumption of alcohol and other addictive substances which can be viewed as a consequence of affluence. Chapter 4 deals with over-nutrition; this chapter will essentially focus on the effects of caloric intake on health and its role in the pathogenesis of metabolic disorders (obesity-related metabolic alterations, type 2 diabetes, and hypertension). In addition, the effects of alcohol as a source of calories will be considered.
6.2 Energy intake, health and longevity
In order for individuals to maintain a constant body composition, energy intake must be balanced with energy expenditure. Resting energy expenditure is proportional to body mass (or more specifically, to lean body mass) and hence is increased in obese patients. As a consequence, at similar physical-activity levels, weight-stable obese patients have higher energy requirements and energy intakes in comparison to nonobese individuals. This has to be compensated by an increased food intake in order to maintain a higher body weight over time.
Excessive body weight, together with a high total energy intake, clearly has deleterious effects on health. This is illustrated by the very significant association between body mass index (BMI), an index of adiposity, and morbidity for various diseases. At the other end of the spectrum, an insufficient energy intake will lead to weight loss, which can be associated with muscle and organ wasting, immune dysfunction, and hence adverse health outcomes. Between these two extremes of obesity and underweight, there is however a full range of energy intakes which allow the maintenance of an individual BMI between 19 and 25 kg/m2. This wide variation illustrates that a normal body weight and food intake are not easily defined. It appears, however, that morbidity is positively correlated with BMI even within the normal range of 19–25 kg/m2 and that the Asian population may have an increased risk at a lower BMI threshold.
The hypothesis that a low energy intake (but above the starvation threshold) is associated with an increased lifespan is supported by animal studies. In protozoans, insects, rodents, and non-human primates, energy restriction reduces the incidence of diseases and extends lifespan. This suggests that the level of energy intake and/or metabolic factors modulates the ageing process. Several hypotheses can be proposed to explain these effects of caloric restriction. A low energy intake reduces body weight and the weight of most individual organs (with the exception of the brain). In some studies, energy restriction lowers the rate of oxygen consumption per kilogram of lean body weight, indicating that tissue metabolism is reduced. In relation with this lowering of tissue metabolic rate, energy restriction decreases the synthesis of reactive oxygen species, and hence the oxidative stress imposed on the organism. The reactive oxygen species generated during oxidative stress cause enzyme deactivations, induce DNA mutations, or alter cell membranes (Figure 6.1).
Energy restriction may also result in a reduction in plasma glucose and insulin concentrations, and an improved insulin sensitivity. In this context, it is of interest that a gene associated with longevity in Caenorhabditis elegans belongs to the family of the insulin receptor gene. Energy restriction has also been shown to be associated with the induction of DNA repair systems. Ageing and energy restriction have opposite effects on tissue gene expression: ageing is associated with an increased expression of genes involved in the stress response in the skeletal muscle of mice, including acute-phase proteins, inflammatory mediators, and inducible DNA repair systems; energy restriction has the opposite effect, which suggests that there is induction of a stress response as a result of damaged proteins during ageing. This response may be secondary to a decrease in cellular systems involved in turnover of cellular constituents. Energy restriction, by increasing the expression of genes coding for biosynthetic processes, may attenuate the effects of ageing. It appears that a family of intracellular proteins, sirtuins, is involved in the adaptation of gene expression to calorie restriction. Sirtuins are a class of proteins that possess histone deacetylase or mono-ribosyltransferase activity, and they have been shown to be involved metabolic adaptations to stress, ageing, and energy deficiency. Sirtuin activation induced by energy deficiency leads to an activation of genes involved in lipid oxidation. Mice submitted to a severe energy restriction maintain constant energy expenditure and increase their physical activity, but these effects are abolished in mice lacking SirT1, a member of the sirtuin family. Energy restriction also failed to increase the lifespan of these sirtuin-deficient mice. Resveratrol, a natural plant phenol, activates sirtuins, but its effects on lifespan in animal models are still under investigation.
6.3 The metabolic syndrome
Obesity, impaired glucose tolerance or type 2 diabetes, hypertension, dyslipidaemia, and nonalcoholic fatty liver disease (NAFLD) are diseases of major importance in Western societies (Box 6.1). Nutritional imbalances and, more specifically, excess calorie intake play a recognised role in the pathogenesis of each of these conditions. Furthermore, these metabolic diseases often appear together, with two or more of them being present in the same individual. The concomitant occurrence of these alterations has therefore been renamed the ‘metabolic syndrome’. Additional features of the syndrome are hyperuricaemia, impaired fibrinolysis secondary to high plasminogen-activator inhibitor concentrations in blood, and ectopic lipid deposition in liver cells (NAFLD) and muscle.
The concomitant occurrence of obesity, hypertension, dyslipidaemia, and impaired glucose tolerance/diabetes has led to the search for a common factor of origin. Insulin resistance and hyperinsulinaemia are frequently observed in association with all the above abnormalities, and may represent a common pathogenic link. The presence of excess intra-abdominal fat and mesenteric fat depots is also closely associated with individual features of the metabolic syndrome. Furthermore, for any given body fat content, fat distribution in the visceral rather than subcutaneous compartment is more closely associated with insulin resistance. Central, visceral obesity may therefore be a common feature linking all constituents of the metabolic syndrome. The mechanisms responsible for preferential deposition of visceral fat in some predisposed individuals are not yet fully understood. Males appear to be at higher risk than females, indicating a role of sex hormones; glucocorticoids and exposure to stress are known to favour visceral fat deposition. However, additional genetic or nutritional factors are also likely to be involved.
- type 2 diabetes/impaired glucose tolerance/insulin resistance;
- and at least two of the following:
- visceral obesity;
- dyslipidaemia (high LDL cholesterol, low HDL cholesterol, high triglycerides);
- hypertension;
- hyperuricaemia;
- high fibrinogen microalbuminuria;
- NAFLD.
- visceral obesity;
6.4 Pathophysiology of insulin resistance
Insulin exerts several different actions at the level of various tissues. Regulation of glucose metabolism is a major action of insulin. In skeletal muscle, adipose tissue, fibroblasts, and several other tissues, insulin increases glucose uptake, oxidation, and storage. These effects are largely secondary to the effect of insulin on specific proteins involved in the facilitated diffusion of glucose from the interstitial space into the cells. In tissues where glucose metabolism is not dependent on insulin concentrations, cells constitutively express glucose-transporter protein isoforms (e.g. GLUT1, GLUT3) on the cell surface. The permanent presence of these proteins on the cell membrane ensures a continuous entry of glucose into the cells. Insulin-sensitive tissues (skeletal muscle, adipose tissue, etc.) express a specific isoform of glucose transporter, GLUT4. GLUT4 has the special feature in that it can be essentially sequestrated into the cells when insulin receptors are not occupied by insulin. When the insulin concentration increases (for example, after a carbohydrate meal), stimulation of insulin receptors on the surface of the cells triggers the rapid migration of GLUT4 from intracellular stores to the plasma membrane. This allows a rapid, several-fold increase in the number of glucose transporters present at the cell surface, and hence the entry of glucose into the cell.
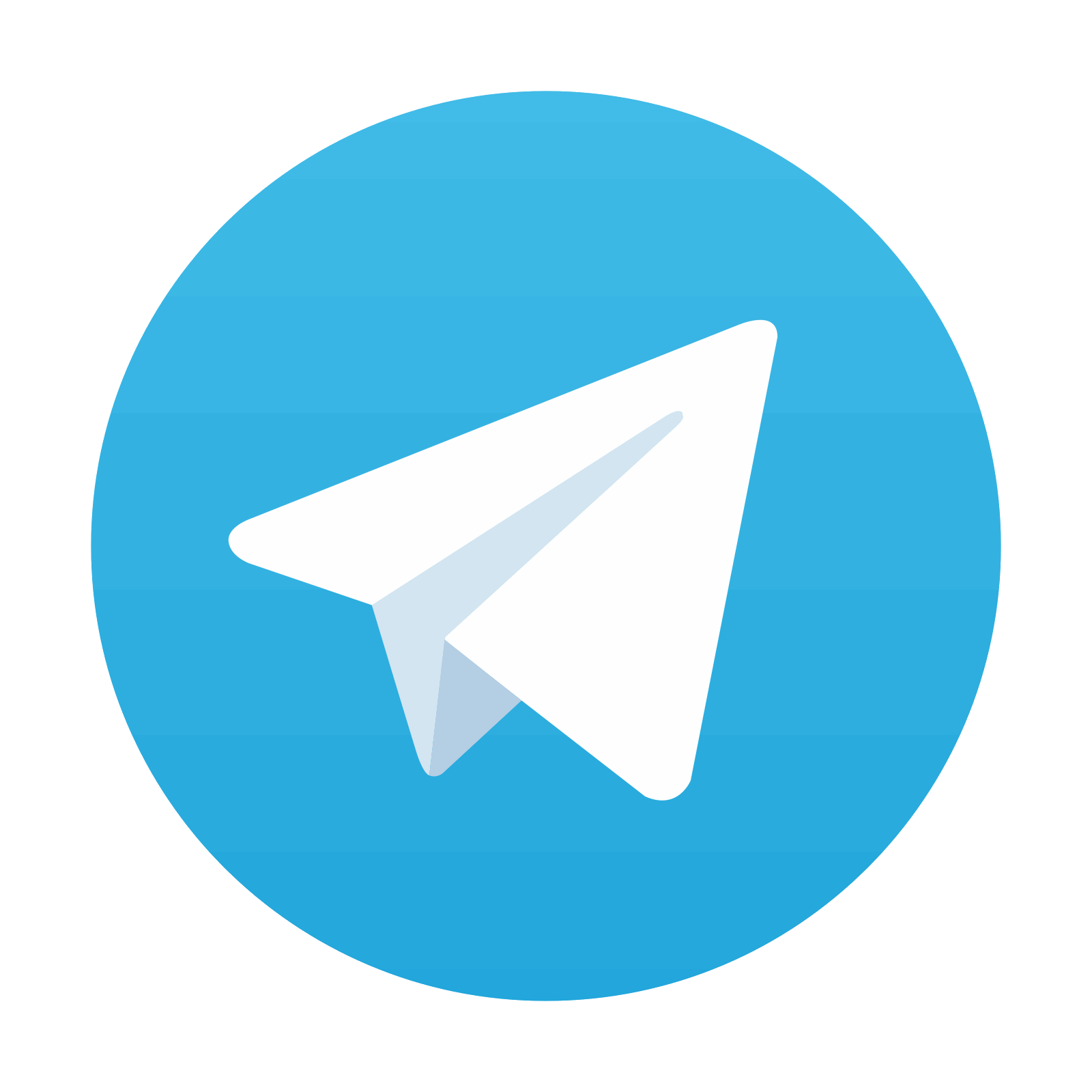
Stay updated, free articles. Join our Telegram channel

Full access? Get Clinical Tree
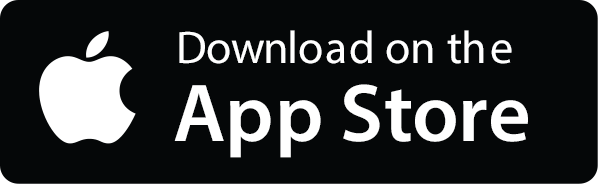
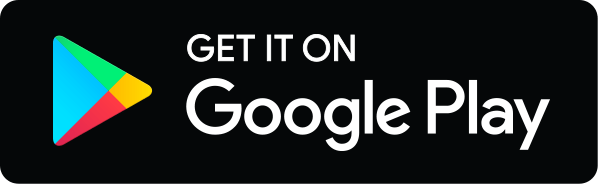