Key points
- •
Radiofrequency ablation is now the standard of care for the treatment of osteoid osteoma.
- •
Surgical treatments carry significant morbidity and recovery times. Improved image guidance techniques drive the increased use of ablation options in treatment of benign bone tumors.
- •
A familiarity with bone biopsy needles, mallets, and drills are needed when performing bone interventions.
- •
Neuroprotective measures including hydrodissection, CO 2 insulation, and nerve monitoring are important adjuncts when ablating near neurovascular bundle or viscera.
Background
Benign bone tumors predominantly affect young patients, and the majority of patients present with symptoms of pain and/or pathologic fracture secondary to the abnormal bone matrix in and around the bone lesion. Surgical resection and stabilization of the bone have served as the time-honored standard of care; however, although complete resection can be achieved in many situations, it is not without morbidity, which can be significant if the tumor to be resected is adjacent to vital structures or requires violation of a joint and the supporting musculotendinous architecture. Furthermore, the integrity of the cortex may be compromised after surgery if hardware for fixation has been inserted or if a prolonged non-weight-bearing status is prescribed. Therefore, balancing the benefits of a complete resection with these risks of the surgical approach is paramount when developing a plan of care for the patient. Over the past two decades, minimally invasive image-guided interventions for benign bone tumors have emerged that can leave the joint and the structural integrity of the underlying bone less disturbed. Thermal ablative techniques have been at the vanguard of these new minimally invasive techniques, , with radiofrequency ablation (RFA) or laser ablation now recognized as the treatments of choice for osteoid osteoma. The application of these techniques to other small, painful, focal bone lesions are emerging ( Table 27-1 ).
Bone Forming Lesions |
Osteoid osteoma |
Osteoblastoma |
Lesions of Cartilaginous Origin |
Enchondroma |
Chondroblastoma |
Chondromyxoid fibroma |
Fibrous, Fibroosseous, and Fibrohistiocytic Lesion |
Fibrous cortical defect |
Fibrous dysplasia |
Desmoplastic fibroma |
Miscellaneous Lesions |
Giant cell tumor |
Unicameral bone cyst |
Aneurysmal bone cyst |
Hemangioma |
Eosinophilic granuloma |
Paget disease |
Oncogenic osteomalacia |
Brown tumor of hyperparathyroidism |
This chapter discusses the use of ablative techniques in the management of osteoid osteoma and other benign bone lesions, presents unique strategies for tackling challenging cases and lesions in difficult locations, and reviews the percutaneous interventions used in the management of unicameral and aneurysmal bone cysts.
Patient selection
The accurate diagnosis of benign bone lesions is important in determining the appropriate management. The diagnosis is mainly based on the patient’s age, clinical presentation, imaging features, and histology. , , For bone lesions, multiplanar, cross-sectional CT imaging is preferred for lesion characterization and procedural planning. The diagnostic imaging will also reveal the presence of an impending fracture, pathologic fracture, or signs of arthritis. Radiography may also be useful as it allows for an accurate determination of the aggressiveness of the lesion and is a better modality for the evaluation of the bony matrix, if present, within a lesion. Additional imaging in the form of radionuclide bone scan and gadolinium-enhanced magnetic resonance imaging (MRI) may be helpful in the workup of equivocal cases.
Although the lesions may be benign, the underlying pathophysiology plays an important role in determining treatment strategies and goals. For example, osteoid osteoma secretes high concentrations of prostaglandins and contains sensory nerve fibers, both of which are felt to be responsible for the characteristic severe pain. However, ablation of the nidus of the osteoid osteoma is sufficient for pain relief and is considered curative treatment. Other tumors, such as hemangioma, manifest rich networks of vascular channels resulting in pain-inducing pressure–volume effects and bone reabsorption. Treatment of these lesions needs to include management of the structural compromise because of the bone reabsorption. Giant cell tumors manifest high concentrations of unopposed osteoclasts at the bone–tumor interface resulting in pain and structural compromise. These lesions can be locally aggressive, infiltrating neurovascular structures and excluding the opportunity for cure by complete surgical resection or thermal ablation. In these cases, palliation and local control become the principal goals, and multimodality therapy involving a combination of medications, embolization, ablation, and structural reinforcement with osteoplasty or surgery is often required. As with all successful outcomes in neoplasm management, a multidisciplinary approach, involving the primary care physician, orthopedic surgeon, neurosurgeon, medical and radiation oncologist, and the interventional radiologist, is the cornerstone of good patient care.
A preprocedural clinical visit is essential and gives the interventional radiologist the opportunity to discuss the procedure, review patient expectations, and define realistic goals for treatment and recovery during the informed consent process. A focused history and physical examination are required. Physical exam findings of neural compromise (either sensory or motor), scoliosis, abnormalities of range of motion or limb length discrepancy should be documented. The findings on physical examination should be correlated with a recent multiplanar, cross-sectional (CT or MRI) imaging study to localize the pain to the lesion site. It is helpful to establish a baseline pain and disability score, objective criteria, which can be monitored following the procedure to determine treatment efficacy. Examples of pain scoring systems that are commonly used include the visual analog scale (VAS) or brief pain inventory (BPI). For lesions in the spine and pelvis causing back pain, the Roland Morris Disability Questionnaire (RMDQ) or Oswestry Disability Index (ODI) can also be used.
The patient’s current medications should be reviewed and anticoagulation medications should be optimized prior to the procedure. Frequently, patients seeking treatment for benign bone lesions are younger and routine preprocedure labs may be unnecessary. In older patients, the Society of Interventional Radiology or American Society of Anesthesia (ASA) guidelines should be followed, and platelet count, prothrombin time, and partial thromboplastin time should be ordered to assess bleeding risk.
Counseling of the patient and family are essential to provide education on expected outcomes, postprocedure pain related to flair response, potential complications, recovery time, and restrictions on activities during the recovery phase. When the lesion involves a weight-bearing long bone, the risk of impeding fracture must be discussed. Although fracture following thermal ablation is uncommon, a few cases have been reported in the literature following radiofrequency ablation (RFA). , In these cases, it may be appropriate to follow the ablation with a cementoplasty procedure to provide structural support; however, the need for possible surgical intervention to stabilize a postablation fracture should always be discussed. When the lesion to be treated abuts an articular surface, the risk of thermal damage to the cartilage, resulting in early-onset arthritis, is a risk that needs to be addressed.
There are a few absolute contraindications to thermal ablation. Lesions less than 1 cm away from a critical structure where hydrodissection, or carbon dioxide insulation cannot be performed (e.g., osteoid osteoma in vertebral body adjacent to spinal cord, lesions adjacent to major motor nerves or the artery of Adamkiewicz) are not suitable for thermal ablation. Patients with skin infection or breakdown in continuity with the bone lesion or those with severe, uncorrectable coagulopathy are not candidates for ablation.
Technique
Ablative modalities
Thermal ablation of tumors either involves heating the tumor tissue to temperatures of 50°C–100°C or freezing the tumor tissue to temperatures below −40°C to induce cell death. , RFA and cryoablation are the most commonly used thermal ablation techniques. Other heat-based thermal ablation modalities include microwave ablation, high-intensity ultrasound (US), and laser photocoagulation.
RFA uses a high-frequency alternating current (460–500 kHz) to deposit thermal energy into tissue, resulting in ionic agitation, frictional heating, and coagulative necrosis. Current RFA generators in the United States are capable of delivering 200–250 W via RF electrodes of varying configurations and sizes (usually 14- to 17-gauge). Three commercially available, FDA-approved, monopolar RF devices are available in the United States: Boston Scientific “LeVeen” (RF 3000, Boston Scientific, Natick, MA); Angiodynamics RITA (Model 1500X, RITA Medical Systems, Mountain View, CA); and, the Covidien Cool-Tip (Boulder, CO) RF ablation systems. The type of RF electrode and the size of the active tip will determine the size and extent of the ablation zone; however, for small, focal bone lesions, a rod electrode is usually sufficient. An initial low wattage between 4 and 10 W depending on lesion size is selected and ablation is carried out based on a manufacturer-prescribed time algorithm or impedance changes in the lesion. For osteoid osteoma treatment, lesions are heated up to 90°C for 6 minutes ( Figure 27-1 ). If there is high impedance during the ablation, the active tip may be buried in the bone, and withdrawing the electrode into the nidus will result in a drop in impedance.
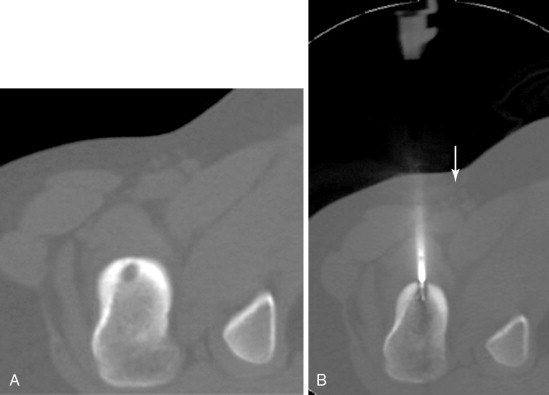
During cryoablation, the delivery of argon gas through a segmentally insulated probe and the rapid expansion of the gas results in rapid cooling (Thompson-Joule effect) with tissue temperatures dropping to below −100°C within seconds. The characteristic properties of the cryoablation zone (“ice ball”), with its changes in intra- and extracellular water composition, allow for visualization using CT imaging and the ice ball appears as an oval or spherical zone of hypoattenuation (see Figure 27-5 ). The outer edge of the ice ball corresponds to 0°C and review of the isotherms of the ice ball is critical to defining the area that falls within the −40°C or below zone. There are two commercially available cryoablation systems available in the United States: Percryo (Healthronics, Austin, TX) and Galil Medical (Galil Medical, Arden Hills, MN). The probe sizes range from 14.7 to 24 mm in diameter. For many authors, cryoablation is the preferred ablation modality for large malignant or benign tumors. The ability to utilize as multiple probes simultaneously allows for the creation of very large ablation zones and nonspherical configurations tailored to the lesion size and location ( Figure 27-2 ). Several published reports have demonstrated the safety and efficacy of cryoablation in benign and malignant tumors.
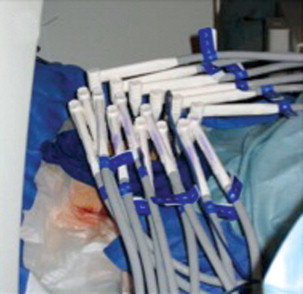
Interstitial laser photocoagulation is widely performed in Europe and is shown to be safe for the treatment of osteoid osteoma. , In this technique, flexible, optical fibers are inserted into the lesion and transmit infrared light energy at low power (typically 2–4 W) to produce heat and coagulative necrosis. The ability of the laser to deliver a fixed energy precisely to a target has made it useful in the treatment of small, focal bone lesions. Other ablative technologies such as microwave, MR-guided high-intensity ultrasonography, and irreversible electroporation are at various stages of development and maturation and have yet to be commonly used in the ablation of benign bone lesion.
Cementoplasty
Cementoplasty, also called osteoplasty or vertebroplasty when performed in the spine, involves the injection of polymethylmethacrylate into the bone to provide structural support and reduce the risk of pathologic fracture. It has been used as a stand-alone or adjunctive procedure in the treatment of benign , and malignant bone tumors. , Because of the direct cytotoxic and thermal effects on tumor cells and sensory nerves, the injection of polymethylmethacrylate has also been shown to provide pain relief. Cementoplasty is a percutaneous technique that is being used increasingly in bone interventions and a familiarity with this procedure is necessary for interventional radiologists who manage bone lesions.
Equipment
Percutaneous ablation procedures can be performed using moderate sedation in adults ; however, for children or adolescents, general, spinal, or epidural anesthesia may be preferable both to eliminate anxiety associated with the procedure and voluntary patient movement during the course of target access. Many authors believe CT imaging for procedural guidance is the best because it provides fast imaging acquisition, superior anatomic resolution of bone tissue, and is compatible with various ablative devices. , The initial preprocedure imaging needs to be adequate to cover the cranial and caudal extent of the lesion for assessment of lesion volume, configuration, and to plan an approach for access. Section thickness of 2–3 mm is recommended for best visualization. As most are pediatric patients, it is important to minimize radiation dose exposure; and, once the lesion has been localized, intraprocedural images acquired during lesion targeting can be obtained with reduced x-ray tube current (often half of even less of the recommended dose by the CT manufacturer).
Performing preprocedural biopsy of the lesion before RFA is controversial with inconclusive biopsy results varying from 27% to 64% in clinically and morphologically unequivocal osteoid osteoma , , ; however, Laredo et al. have argued that histopathologic confirmation of the diagnosis may help, especially in cases of treatment failure or disease recurrence. There is really no disadvantage to performing a biopsy: tissue sampling can be achieved during lesion access and no additional time or complexity is added to the procedure.
Frequently, there is no thinning or weakening of the overlying cortical bone, which can be quite hard in children and young adults, and access becomes the most challenging aspect of the procedure. Ablation probes are not designed to be hammered, screwed, or muscled into the bone and will break relatively easily; therefore, the interventional radiologist must become comfortable with several techniques and tools to gain access to the bone lesion safely. Sturdy bone biopsy needles (14-gauge Bonopty needle [Apriomed, Uppsala, Sweden], Laurane biopsy needle [Laurane Medical, Saint-Arnoult, France], or 13- or 11-gauge Osteosite M2 bone biopsy needle [Cook Medical, Bloomington, IN]) can be used in conjunction with mallets, hand drills, or a Stryker 4200 cordless drill (Stryker Instruments, Kalamazoo, MI) with Steinmann pins to create an osteotomy to gain access to the lesion. Once an osteotomy has been made, a coaxial technique can be used to exchange the access needle for the ablation probe ( Figure 27-3 ).
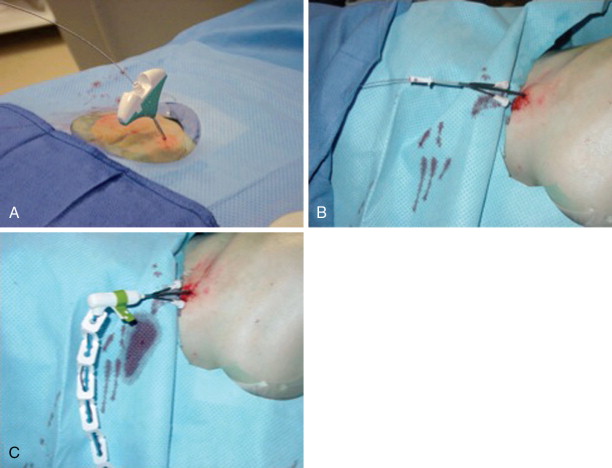
Technical challenges and adjunct maneuvers
A bone or soft tissue lesion will often be in proximity to a critical structure such as a major neurovascular bundle, spinal cord, bladder, or bowel. As a general rule, if the intended ablation zone is to be within 1 cm of a vital structure, additional measures and precautions are necessary to prevent collateral damage. The placement of a thermocouple next to a critical structure can be used for real-time temperature monitoring during the ablation ( Figure 27-4 ). The temperature of the nontarget critical structure should not exceed 45°C in RFA cases or fall below 8°C in cryoablation cases. , Various thermal insulation techniques have been used to protect structures at risk for damage during percutaneous ablation procedures. The instillation of carbon dioxide (CO 2 ) has been widely used as insulation technique in ablation. 20,37,39-41 CO 2 is highly soluble, nonallergenic, and lacks renal or hepatic toxicity. It is easily reabsorbed by vessels, eliminated by the respiratory system, and has low thermal conductivity. It can be injected through a 21-gauge spinal needle that is interposed between the lesion and the structure at risk ( Figure 27-5 ), and volumes range from a few milliliters in the epidural space ( Figure 27-6 ) to 1.5 L in the peritoneal cavity. Because CO 2 can be absorbed, periodic evaluation with imaging is required to monitor the gas volume and determine the need for the injection of additional volume.
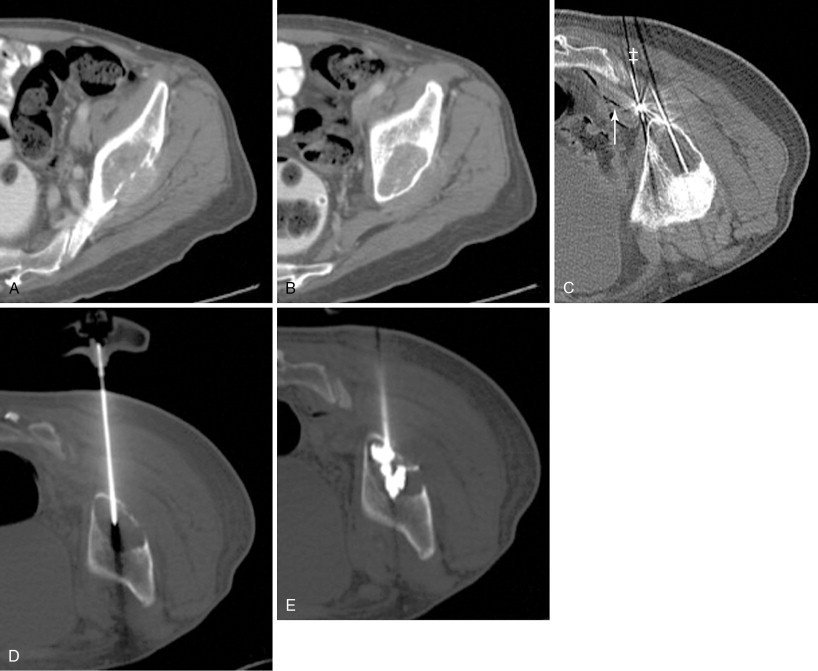
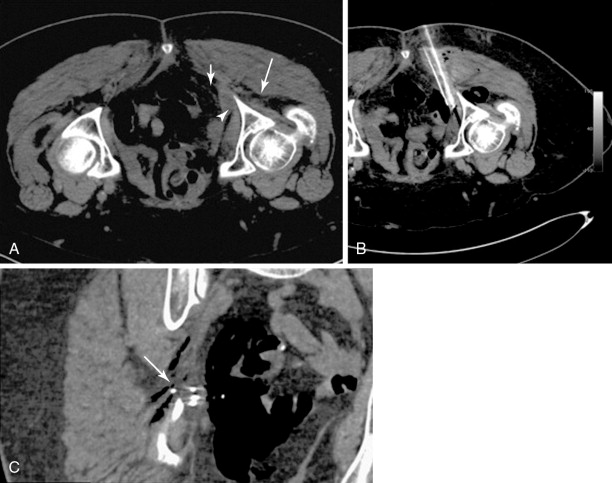
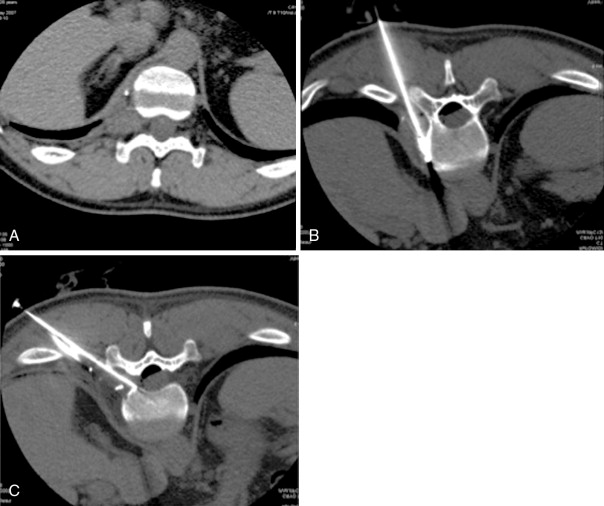
Hydrodissection can be performed to displace and insulate critical structures during ablation. In the abdomen and pelvis, the injection of fluid can be used to displace bowel away from the ablation zone and provide an added margin of safety when ablating liver, renal, or adrenal tumors. Fluid can also be injected into the epidural space for use as a cooling method during thermal ablation of spinal tumors or injected into the joint space to protect the cartilage during thermal ablation of articular or juxtaarticular lesions , , ( Figure 27-7 ). Sterile saline or dextrose (5%) in water (D5W) for RFA procedures can be injected through a 21-gauge needle. The fluid injected can also be cooled or warmed to cool down or warm up the anatomic structures as risk. A dilute amount of contrast (20:1 in strength) can also be added to the fluid to improve visualization. Fluid injection may not be appropriate for use during cryoablation as the fluid may freeze on contact with the ice ball and increase the potential for thermal damage ( Figure 27-8 ).
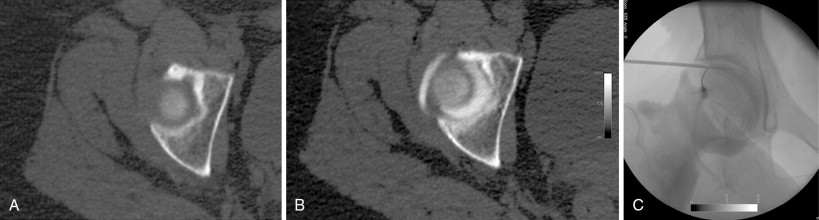
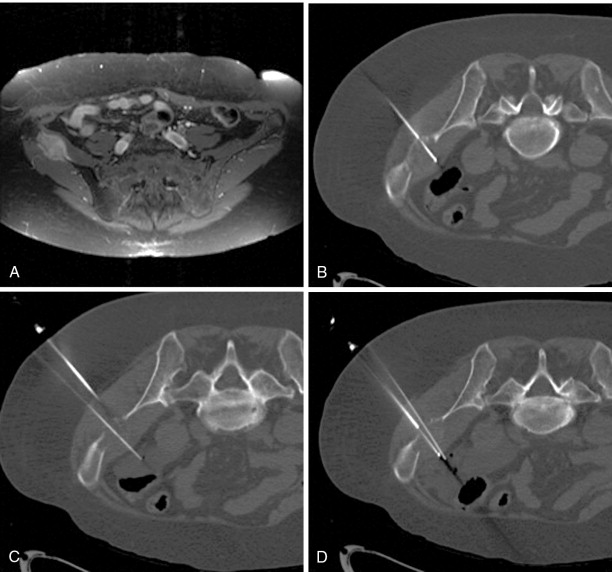
Skin injury in the form of burns or frostbite is a risk with any thermal ablation procedure. Placing a sterile glove filled with either warmed or cooled saline is often sufficient to protect the skin from thermal injury ( Figure 27-9 ). The subdermal injection of sterile saline or lidocaine 1% to create a wheal of fluid under the skin at the site of ablation is commonly used to prevent skin injury during the treatment of superficial lesions ( Figure 27-10 ).
