Molecular Genetics
Although this book is devoted mainly to immunophenotyping of hematologic neoplasms by flow cytometry and immunohistochemistry, molecular genetics is incorporated in the discussion of all our cases, as it has played an increasingly important role in the diagnosis and prognostication of lymphomas and leukemias (1, 2, 3, 4 and 5). The role of molecular genetics, however, may differ from one entity to another and can be divided into three categories:
Mandatory for diagnosis: For the diagnosis of Burkitt lymphoma and chronic myelogenous leukemia (CML), C-MYC oncogene and breakpoint cluster region-Ableson (BCR-ABL), respectively, are the absolute diagnostic markers. There are no substitutes for these markers that are acceptable for a definitive diagnosis. Two subtypes of acute myeloid leukemia (AML) are also defined by cytogenetic karyotypes (6). The first one is t(8;21) (q22;q22) or AML1/eight-twenty-one (ETO), and the second one is inv (16) (p13q22) or t(16;16) (p13;q22) or core binding factor/smooth muscle myosin heavy chain (CBFβ/MYH11). These genetic abnormalities are so characteristic that they supersede the blast count for the diagnosis of AML. Both karyotypes also confer a good prognosis.
Markers for treatment or prognosis: For the diagnosis of acute promyelocytic leukemia (AML-M3) a morphologic diagnosis is possible, but the identification of t(15;17)(q22;q12) or promyelocytic leukemia/retinoic acid receptor alpha (PML/RARα) is not only important for the confirmation of the diagnosis but also for the treatment. If the leukemic cells carry other variants, such as t(11;17), patients will not respond to all-transretinoic acid treatment (6). For anaplastic large cell lymphoma, the anaplastic lymphoma kinase (ALK) gene is not necessarily present in each case, but the presence or absence of this gene will greatly influence the clinical course, the therapeutic strategy, and the prognosis (7).
Confirmation of diagnosis: For mantle cell lymphoma, there are many morphologic and immunologic criteria for the diagnosis, but the results of these tests are frequently equivocal, and the demonstration of t(11;14) (q13;q32) or B-cell lymphoma/leukemia/immunoglobulin H (BCL-1/IgH) is essential for a definitive diagnosis (8). Lymphoplasmacytic lymphoma is usually diagnosed by exclusion of many other lymphomas, but the detection of t(9;14) (p13;q32) or /immunoglobulin H (PAX5/IgH) will confirm the diagnosis in cases of nonsecretory lymphoplasmacytic lymphoma (cases without Waldenström macroglobulinemia) (9).
CYTOGENETICS
Cytogenetics plays multiple roles in relation to hematologic neoplasms (4,10,11). In clinical practice, the most useful occasion for cytogenetics is in determining the nature of a tumor in equivocal cases when immunophenotyping or immunogenotyping demonstrated monoclonality of the tumor and yet morphologic evidence of malignancy is lacking. In these cases, an abnormal karyotype casts a decisive vote for malignancy (10,12). Even when monoclonality is not identified, chromosomal aberration is a strong indication of malignancy or premalignancy (10,11).
Although most lymphomas and leukemias still have no diagnostic chromosomal pattern, karyotyping is the most reliable tool for a definitive diagnosis of many hematologic neoplasms. As mentioned before, it is particularly useful for the diagnosis of CML, Burkitt lymphoma, acute promyelocytic leukemia, mantle cell lymphoma, and anaplastic large cell lymphoma, just to name a few (Fig. 4.1).
Chromosomal aberrations are classified into numeric and structural abnormalities. Structural abnormalities include translocations, deletions, inversions, duplications, and isochromosomes. Among these, reciprocal translocation is most commonly found in hematologic neoplasms. This translocation involves the exchange of segments between two chromosomes; the total number of chromosomes is unchanged.
The numeric abnormalities are subdivided into polyploid and aneuploid. The term polyploid refers to multiplication of the normal haploid number of 23. Thus diploidy refers to 46 chromosomes, triploidy 69, and tetraploidy 92. Aneuploid, in contrast, refers to multiplication of chromosomes by irregular numbers, for instance, monosomy and trisomy. As the result of monosomy in a single chromosome, the total number of chromosome becomes 45. When
trisomy is present in a single chromosome, the number of chromosome becomes 47.
trisomy is present in a single chromosome, the number of chromosome becomes 47.
In addition, cytogenetics is a reliable predictor for prognosis. Generally speaking, neoplasms carrying a normal karyotype have a better prognosis than those with an abnormal one. There are, however, a few exceptions. For instance, cases of anaplastic large cell lymphoma that express t(2:5) have a more favorable prognosis than those without this karyotype (7). In childhood acute lymphoblastic leukemia (ALL), cases with hyperdiploidy carry a better prognosis than do diploidy cases (13). The prediction of prognosis by karyotyping is sometimes associated with the histologic pattern. For instance, a favorable prognosis with t(14;18) is due to its association with follicular lymphoma, whereas the poor prognosis predicted by t(8;14) is due to its association with Burkitt lymphoma (14). Some numeric chromosomal abnormalities, such as +5, +6, or +8, are related to shorter survival in patients with non-Hodgkin lymphoma (15). In AMLs and myelodysplastic syndromes, karyotyping is routinely performed even in entities without a specific karyotype, because the presence or absence of chromosomal aberration and the presence of a particular karyotype are important to predict the prognosis.
Cytogenetic studies are also useful in monitoring clinical course. When the patient has a relapse, karyotyping is able to determine whether the tumor is recurrent or secondary (16). Structural chromosomal abnormalities usually initiate malignant transformation, whereas numeric chromosomal abnormalities represent chromosomal evolution leading to the progression of disease (17). The appearance of new abnormalities in the karyotype, no matter whether the abnormality is structural or numerical, signals transformation of the tumor to a higher grade malignancy (10).
Finally, cytogenetic analysis is also able to detect residual minimal disease. The detection of residual tumor cells is frequently based on the use of molecular genetic techniques, such as polymerase chain reaction (PCR) or the fluorescence in situ hybridization (FISH) technique.
Fluorescence In Situ Hybridization
Conventional karyotyping requires fresh specimens, which are cultured to harvest cells in the metaphase for chromosome analysis. Therefore, it is time consuming and costly, and it frequently obtains unsatisfactory results because of the failure in cell growth or because the normal population overgrows the tumor cells. FISH overcomes all of these deficiencies of karyotyping (11,18), and can identify the cytogenetic abnormality in cells at the interphase. Therefore, it can be applied to dried smears of bone marrow, peripheral blood, body fluids, and paraffin sections. Because cell culture is not required, the failure rate is very low. However, FISH can also be applied to cultured cells in metaphase under special conditions. A large number of cells can be analyzed by FISH so that a percentage of positive cells can be calculated. Therefore, this analysis is particularly useful in therapeutic monitoring. FISH is more sensitive than karyotyping as it can detect cryptic or masked translocations. For instance, 5% of patients with CML have a Philadelphia chromosome from a submicroscopic insertion translocation. Therefore, the aberration is not detectable by conventional karyotyping, but is readily detected by FISH (11).
FISH involves the binding of fluorochrome-labeled DNA probes to target DNA or RNA sequences inside fixed
cells. The basic principle is that a single-stranded DNA can bind to a complementary single-stranded DNA, and this hybridization process can be demonstrated with fluorochrome labels. Normal cells contain two alleles of each chromosome; therefore, two signals for a certain chromosome can be demonstrated by a specific DNA probe in normal cells. When one signal or three signals representing the same chromosome are demonstrated in individual cells, this represents numeric abnormalities (monosomy or trisomy) (Figs. 4.2 and 4.3). Chromosomal translocation can be demonstrated by showing two overlapped signals from two different genes (Fig. 4.4). Chromosomal inversion can be detected in the culture cells at the metaphase.
cells. The basic principle is that a single-stranded DNA can bind to a complementary single-stranded DNA, and this hybridization process can be demonstrated with fluorochrome labels. Normal cells contain two alleles of each chromosome; therefore, two signals for a certain chromosome can be demonstrated by a specific DNA probe in normal cells. When one signal or three signals representing the same chromosome are demonstrated in individual cells, this represents numeric abnormalities (monosomy or trisomy) (Figs. 4.2 and 4.3). Chromosomal translocation can be demonstrated by showing two overlapped signals from two different genes (Fig. 4.4). Chromosomal inversion can be detected in the culture cells at the metaphase.
![]() FIGURE 4.2 Fluorescence in situ hybridization technique demonstrates the deletion of one retinoblastoma (Rb) gene (single red signal). |
Depending on the targeted abnormalities, a certain probe should be selected (11). For the detection of quantitative abnormalities, the centromere-specific probes should be used. For the detection of structural abnormalities, such as reciprocal translocations and inversions, locus-specific probes are most frequently used. Telomere-specific probes are used to detect subtle chromosome abnormalities that involve the ends of chromosomes. Whole chromosome-specific paints with sequence DNA probes are used to identify marker chromosomes and to detect cryptic translocations.
![]() FIGURE 4.3 Fluorescence in situ hybridization technique demonstrates trisomy 12 in a case of chronic lymphocytic leukemia with prolymphocytoid transformation. |
FISH probes can be used with different strategies, including single fusion, double fusion, break-apart, and extra-signal strategy. For technical details, the reader is referred to reference 11.
MOLECULAR BIOLOGY
FISH can be considered a hybrid of molecular biology and cytogenetics. In addition to FISH, the most commonly used molecular biology techniques in clinical laboratories are Southern blotting and PCR. The variants of PCR include reverse transcriptase PCR (RT-PCR) and quantitative PCR (real-time PCR). In hematology, molecular biology techniques are used to detect rearrangements of the antigen receptor genes (immunoglobulin and T-cell receptor [TCR]) and oncogenes, to identify clonalities of the suspected tumor cells and oncogene translocations. The most current techniques are the analysis of the variable region of immunoglobulin heavy-chain gene (VH gene) to determine the developmental stage of a particular B-cell tumor, and gene expression profiling (GEP), which can stratify lymphomas and leukemias by using a large number of gene probes for the purpose of diagnosis and prediction of therapeutic response as well as prognosis.
Immunoglobulin and T-Cell Receptor Gene Rearrangement
Surface immunoglobulin and TCR are the definitive markers of B cells and T cells, respectively. Their production is
controlled by a series of genes (19, 20 and 21). The variable region of the heavy chain of an immunoglobulin molecule is encoded for by three genes, V (variable), D (diversity), and J (joining). The constant region of either heavy chains or light chains is encoded by one gene, C (constant). The heavy-chain (H) gene has about 50 to 100 VH segments, 25 to 30 DH segments, and 6 JH segments (Table 4.1). The κ light-chain gene has about 40 to 80 Vk segments and 1 Jk segment. The λ light chain has about 40 VL segments and 6 JL segments. The light-chain genes have no D segments. All of these segments are arranged in an orderly array on various chromosomes: The heavy-chain gene locus is on 14q32, the κ light-chain locus is on 2p12, and the λ light-chain locus is on 22q11. During the differentiation of the B cell, a series of gene rearrangements takes place (Fig. 4.5).
controlled by a series of genes (19, 20 and 21). The variable region of the heavy chain of an immunoglobulin molecule is encoded for by three genes, V (variable), D (diversity), and J (joining). The constant region of either heavy chains or light chains is encoded by one gene, C (constant). The heavy-chain (H) gene has about 50 to 100 VH segments, 25 to 30 DH segments, and 6 JH segments (Table 4.1). The κ light-chain gene has about 40 to 80 Vk segments and 1 Jk segment. The λ light chain has about 40 VL segments and 6 JL segments. The light-chain genes have no D segments. All of these segments are arranged in an orderly array on various chromosomes: The heavy-chain gene locus is on 14q32, the κ light-chain locus is on 2p12, and the λ light-chain locus is on 22q11. During the differentiation of the B cell, a series of gene rearrangements takes place (Fig. 4.5).
TABLE 4.1 Characteristics of Receptor Genes | |||||||||||||||||||||||||||||||||||||||||||||||||
---|---|---|---|---|---|---|---|---|---|---|---|---|---|---|---|---|---|---|---|---|---|---|---|---|---|---|---|---|---|---|---|---|---|---|---|---|---|---|---|---|---|---|---|---|---|---|---|---|---|
|
The heavy-chain gene rearrangement takes place in the pre-B cell stage when the recombinases encoded by recombination activating gene (RAG1) and RAG2 genes mediate the random rearrangement of one each of the D and J gene segments. This is followed by the rearrangement of one of the V gene segments. After the completion of the VD-J rearrangement, the DNA is transcribed into messenger RNA (mRNA) with splicing of the rearranged segments next to the constant (C) region gene. This RNA transcript is then translated into heavy-chain immunoglobulin. The light-chain genes go through the same process and translate into light-chain immunoglobulins in a later stage. The light-chain and heavy-chain immunoglobulins will then combine and form an intact immunoglobulin molecule. The κ light-chain gene is usually rearranged first. If the κ gene rearrangement fails to produce a κ light-chain protein, the λ chain will then be rearranged (22,23).
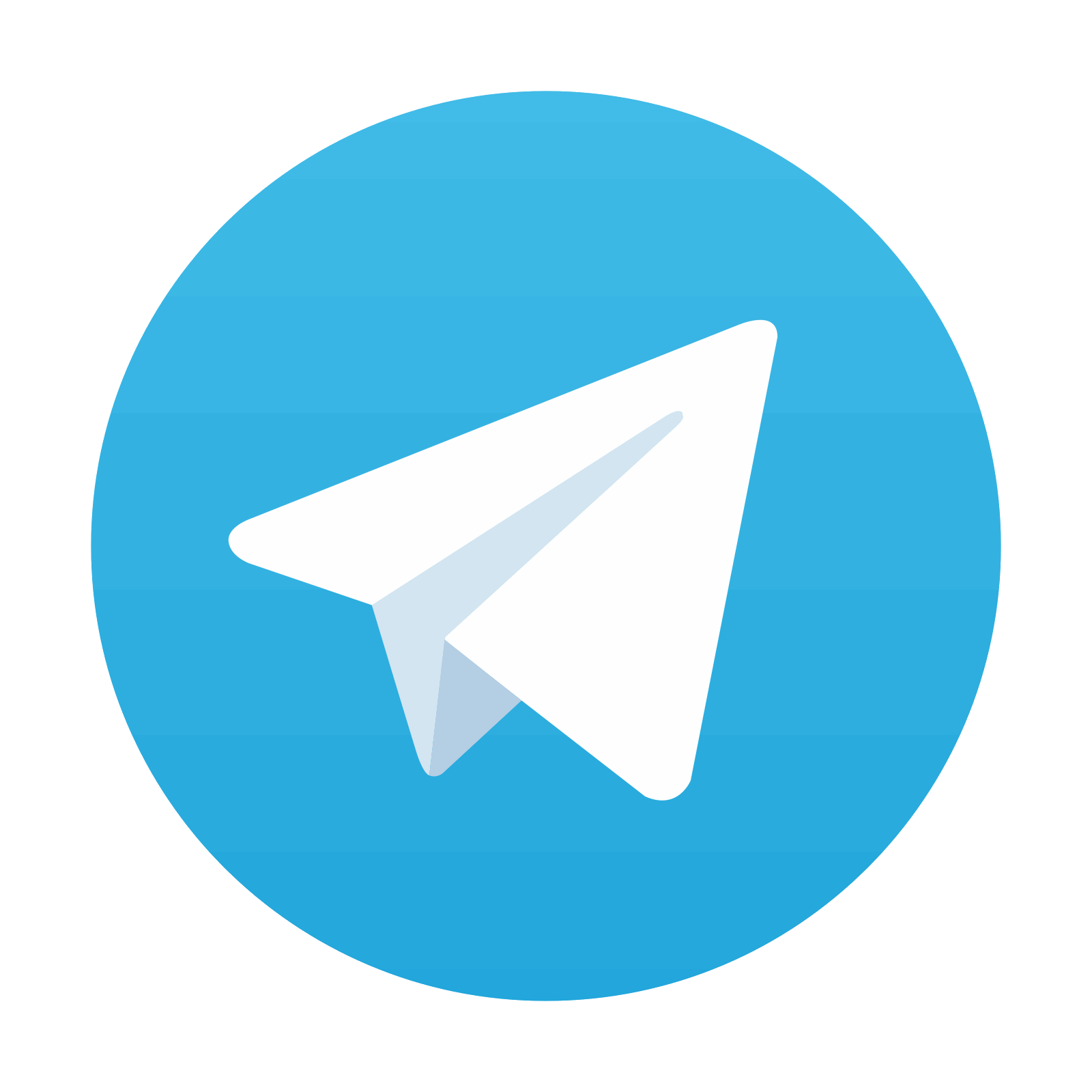
Stay updated, free articles. Join our Telegram channel

Full access? Get Clinical Tree
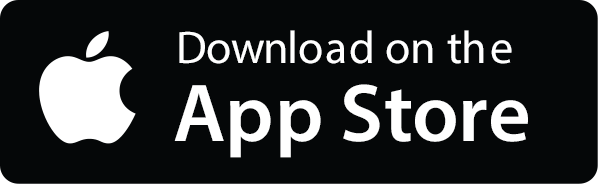
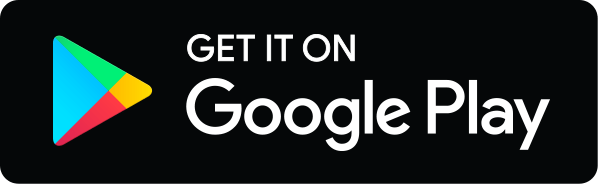