Raphael Dolin
Miscellaneous Antiviral Agents (Interferons, Imiquimod, Pleconaril)
Antiviral agents are discussed here that have activity against a variety of viral infections in addition to those addressed in Chapters 43 to 46. The major clinical use of interferons is in treatment of hepatitis B and C, which is discussed in Chapter 46.
Interferons
Classification
Since their discovery in 1957 as mediators of the phenomenon of viral interference (i.e., inhibition of growth of one virus by another), interferons (IFNs) have become recognized as potent cytokines that are associated with complex antiviral, immunomodulating, and antiproliferative actions.1–3 IFNs are proteins that are synthesized by eukaryotic cells in response to various inducers and that cause biochemical changes leading to a nonselective antiviral state in exposed cells of the same species. Three subfamilies of IFNs are recognized. Type I IFNs are the largest subfamily and include the IFN-αs (13 subtypes in humans) and the IFN-βs. The type II subfamily has only one member, IFN-γ. Type III is the subfamily most recently identified and includes IFN-λ,4 of which there are three subtypes (λ1, λ2, λ3), also known as interleukin (IL)-28, IL-29, and IL-28R.5,6 A fourth interferon λ subtype (λ4) has been recently identified.6a The type I IFNs are clustered on the short arm of chromosome 9 in humans,7 Type II IFN is on chromosome 12, and type III IFNs are encoded on chromosome 19.8 Formerly designated on the basis of the cell types from which they were derived, the IFN-αs, the IFN-β, and IFN-γ are the IFNs currently in clinical use (Table 47-1), whereas IFN-λ is being studied for hepatitis C. Each type is immunologically distinct and has different producer cells, inducers, and biologic effects and unique physicochemical characteristics.2,4,9
TABLE 47-1
Nomenclature and Classification of Human Interferons (IFNs)
SUBFAMILY | TYPE I | TYPE I | TYPE II | TYPE III |
Class* | α | β | γ | λ |
No. of subtypes | 13 | 1 | 1 | 4 |
Receptor | IFNAR1/2 | IFNAR1/2 | IFNGR1/2 | IL10R2, IFNLR1 |
Human chromosome | 9 | 9 | 12 | 19 |
Commercial formulations | rIFN-α2b (Intron A) IFN-α2a (Roferon-A) Le-IFN-αn3 (Alferon N) Ly-IFN-αn1 (Wellferon) rIFNalfacon-1 (Infergen) Peg-IFN-α2a (Pegasys) Peg-IFN-α2b (PEG-Intron) | rIFN-β1b (Betaseron) rIFN-β1a (Avonex, Rebif) | rIFN-γ1b (Actimmune) rIFN-γ (Immuneron) | Peg-IFNλ1 |
* Type I classes in humans also include IFNε and IFNώ.
The IFN-αs and IFN-βs are produced by almost all cells in response to viral infection and various other stimuli, including double-stranded RNA (dsRNA); bacteria; protozoa; mycoplasmas; polyanions; several low-molecular-weight organic compounds; and certain cytokines and growth factors, such as IL-1, IL-2, and tumor necrosis factor (TNF). IFN-γ production is restricted to T lymphocytes and natural killer cells responding to antigenic stimuli, mitogens, and certain cytokines, such as IL-2. The IFN-λs also appear to be produced by multiple cell types.10 The principal antiviral IFNs, IFN-αs and IFN-βs, are approximately 30% homologous at the amino-acid level. The human IFN-αs share a high degree of amino-acid sequence homology (>70%) but have differing in vitro antiviral and biologic effects on human cells.11 Compared with the IFN-αs and IFN-βs, IFN-γ has less antiviral activity but more potent immunoregulatory effects, particularly with respect to macrophage activation, expression of class II major histocompatibility complex (MHC) antigens, and mediation of local inflammatory responses. Most IFNs in clinical use are produced by recombinant DNA techniques (see Table 47-1).
Mechanisms of Action
A wide range of animal viruses are sensitive to the antiviral actions of IFNs, although many DNA viruses are relatively insensitive and considerable differences in potency exist among viruses and assay systems. IFN activity is usually measured in terms of antiviral effects in cell culture. Typically, one unit of IFN activity is the amount present in a sample dilution that causes a 50% reduction in virus replication or expression in certain cell lines; this is generally expressed as international units (IU) relative to National Institutes of Health or World Health Organization reference standards.
IFNs are not directly antiviral but cause elaboration of effector proteins in exposed cells, which contribute to a state of viral resistance.1,12,13 The initial step involves IFN binding to specific cell surface receptors. For the type I IFNs (IFN-αs and IFN-β) the cognate receptor is IFNAR1/2. Type II IFNs (IFN-γ) use the homodimeric receptors, IFNGR1/2. Type III IFNs (IFN-λ) signal through a receptor complex consisting of IL10R2 and IFNLR1.6,14 IFN receptors are linked to the Janus kinase/signal transducer and activator of transcription (JAK-STAT) signaling pathways, which, through a multistep process, activate transcription factors that bind selectively to and upregulate approximately 100 IFN-regulated genes.15–17 The distinct pattern of STAT proteins activated by different IFNs is one mechanism for eliciting different cellular responses. For IFN-α and IFN-β, a three-protein complex known as IFN-stimulated gene factor 3 (ISGF-3) localizes to the nucleus and binds to a cis-acting DNA element (designated IRSE) that activates transcription of the target genes.1
A family of IFN regulatory factors exists, and other pathways may contribute to regulation of the IFN response. Microarray analysis shows that many genes are upregulated by IFN-β but not by IFN-α or IFN-γ in vitro.15 The onset of IFN-induced antiviral action is rapid, and IFN exposure leads to production of more than two dozen cellular proteins. For many viruses, the primary antiviral effect of IFN in vitro is mediated by inhibition of viral protein synthesis. Depending on the virus and cell type, the antiviral actions of IFNs may also include inhibition of viral penetration or uncoating, synthesis or methylation of messenger (m)RNA, or viral assembly and release.
Among the better-characterized IFN-induced proteins are unique 2′-5′-oligoadenylate (2-5[A]) synthetases and protein kinase R (PKR), either of which can inhibit protein synthesis in the presence of dsRNA.1 The 2-5(A) synthetase produces adenylate oligomers that activate a latent cellular endoribonuclease (RNase L) to cleave cellular and viral single-stranded (ss)RNAs, leading to inhibition of protein synthesis. Activated PKR selectively phosphorylates and inactivates eukaryotic initiation factor (eIF)-2 to impede translation. Activated PKR also phosphorylates the transcription factor inhibitor IκB and mediates dsRNA-induced activation of nuclear factor (NF)-κB, which is required for IFN-β synthesis. IFNs may also block mRNA capping by inhibiting transmethylation reactions. IFNs also induce human guanylate binding protein-1, which mediates antiviral activity for several RNA viruses; the soluble form of the low-density lipoprotein receptor inhibitory for rhabdovirus assembly18; the MxA protein (a guanosine triphosphatase with activity against orthomyxoviruses and certain RNA viruses)19; and the RNA-specific adenosine deaminase ADR1, which modifies RNA transcripts after transcription.1
IFNs also inhibit hepatitic C virus (HCV) internal ribosome entry site–dependent RNA translation in vitro.20 Induction of nitric oxide synthase seems to mediate a substantial antiviral effect of IFN-γ.21 Increased levels of 2-5(A) synthetase activity and MxA protein or mRNA in peripheral leukocytes are also used as a marker for IFN exposure or endogenous release.19
Except possibly for the Mx proteins and influenza viruses and for 2-5(A) synthetase/RNase-L and picornaviruses, no consistent correlations exist between induction of a particular protein and resistance to a specific virus across a range of cell types.13 A particular virus may be inhibited at several steps, and the principal inhibitory effect differs among virus families. Many viruses are able to counter IFN effects by blocking signaling and production or activity of selected IFN-inducible proteins.1,13 The NS5A protein of HCV represses the function of the IFN-induced PKR,22 and another hepatitis C virus protein E2 competitively inhibits PKR kinase activity. The NS1 gene of influenza is an IFN antagonist that binds dsRNA to inhibit IFN production and dsRNA-activated pathways. IFN exposure may also reduce the expression of certain cellular genes, including selected oncogenes and genes involved in collagen synthesis.
The viral and immune IFN systems are functionally nonredundant,1,23 and complex interactions exist between IFNs and between IFNs and other parts of the immune system.9,24 IFNs upregulate major histocompatibility complex (MHC) class I expression and promote cytotoxic T-cell responses, regulate the expression of cytokines (IL-12, IL-15, IFN-γ) and chemokines that affect T-cell responses, alter expression of Toll-like receptors (TLRs), enhance natural killer cell cytotoxicity, and promote the differentiation of dendritic cells and T helper type 1 (Th1) lymphocytes.12,24 IFN-α is produced by macrophages and can modify macrophage functions, increasing phagocytosis and cytolytic activity. Consequently, IFNs may ameliorate viral infections by exerting direct antiviral effects and by modifying the immune response to infection. IFN-induced expression of MHC antigens may contribute to the antiviral actions of IFN by enhancing antigen presentation and the lytic effects of cytotoxic T lymphocytes. The IFN-αs, the IFN-βs, and IFN-γ lead to increased expression of class I MHC molecules, but only IFN-γ efficiently induces class II MHC molecules.1 Several viruses, including cytomegalovirus and varicella-zoster virus, antagonize IFN-γ–induced MHC expression. In addition, proapoptotic and antiapoptotic genes are induced by IFNs15 and IFN-α and IFN-β are important mediators of apoptosis, including induction of TP53.1,25
IFN titers generally appear at the sites of viral replication just after peak titers of virus and before humoral antibody responses. IFNs may mediate some of the systemic symptoms associated with viral infections and contribute to immunologically mediated tissue damage in certain viral diseases. High IFN titers are usually followed by a reduction of virus titers, although persistently elevated IFN titers have been recognized in certain chronic and acute viral infections (e.g., hemorrhagic fevers).
Pharmacokinetics
The prolonged biologic effects of IFNs are not easily related to serum concentrations or other conventional pharmacokinetic parameters. After intramuscular or subcutaneous injection of IFN-α, absorption is greater than 80%.26,27 Plasma levels are dose related, peaking at 4 to 10 hours and returning to baseline by 18 to 36 hours. Levels of 2-5(A) synthetase in peripheral blood mononuclear cells, which have been used as an index of biologic responsiveness to IFN, show increases beginning at 6 hours and lasting through 4 days after a single dose. An antiviral state in these cells is detectable at 1 hour, peaks at 24 hours, and slowly decreases to baseline by 6 days after injection. Intramuscular or subcutaneous injections of IFN-β result in negligible plasma levels, although increases in 2-5(A) synthetase may occur. Oral administration does not result in detectable serum IFN levels or increases in 2-5(A) synthetase activity in peripheral blood mononuclear cells.28
After systemic administration, low levels of IFNs are detected in respiratory secretions, cerebrospinal fluid, eye, and brain. After intravenous dosing, cerebrospinal fluid levels average less than 1% of serum concentrations.29 The IFN-αs are stable in most body fluids, whereas the IFN-βs and IFN-γ seem to lose activity readily. It is unknown, however, whether measurable IFN levels at a particular site accurately reflect its antiviral or other biologic activities. The IFN-αs and the IFN-βs are cleared rapidly in a complex fashion. Leukocyte and recombinant IFN-α species have a plasma elimination half-life () of 3 to 8 hours. The clearance of IFN includes inactivation by various body fluids, cellular uptake, and metabolism by body organs, primarily the kidney, although negligible biologically active IFN is excreted in the urine. Clearance of IFN-α2 is reduced by 64% to 79% in hemodialysis patients.30
The attachment of polyethylene glycol to IFN slows absorption, decreases clearance, increases , and results in higher and more sustained serum concentrations, so that once-weekly dosing is effective. Two types of pegylated (peg) IFN-α are currently approved: peg-IFN-α2a has a 40-kDa branched polyethylene glycol moiety attached by a stable amide bond to lysine residues within the IFN protein, and peg-IFN-α2b has a 12-kDa linear moiety attached to histidine residues. Peg-IFN-α2a is more stable and dispensed in solution, whereas peg-IFN-α2b requires reconstitution before use. Peg-IFN-α2a is cleared primarily by the liver, whereas about 30% of peg-IFN-α2b is cleared renally.30 For peg-IFN-α2a (multiple 180-µg doses), a peak serum concentration of 26 ng/mL occurs at about 45 hours after the dosing, and
is 80 to 90 hours. Steady-state serum levels are attained 5 to 8 weeks after initiation of weekly dosing. Moderate renal impairment and presence of cirrhosis do not affect pharmacokinetics, although clearance is reduced by 25% to 45% in patients with renal failure on hemodialysis. For peg-IFN-α2b, dose-related maximal plasma concentrations (1.4 ng/mL with multiple doses of 1.5 µg/kg) occur at 15 to 44 hours after dosing and decline with a
of 30 to 40 hours, or about 10-fold longer than for IFN-α2b.28 Some accumulation occurs with repetitive dosing. Dosage reductions in both peg-IFNs are indicated in end-stage renal disease.
Interactions
IFN and its inducers reduce the metabolism of various drugs by the hepatic cytochrome P-450–dependent mixed-function oxidase system and specifically decrease CYP1A2-mediated clearance of theophylline. IFNs may increase the neurotoxic, hematotoxic, or cardiotoxic effects of other drugs, including increased risk for anemia with ribavirin.
Toxicity
Purified natural and recombinant IFNs are associated with dose-related immediate- and late-onset toxicities.31 Adverse effects are generally mild and reversible at dosages of less than 5 million IU/day.27 Intramuscular and subcutaneous injections of IFN doses of 1 to 2 million IU or more are usually associated with an acute influenza-like syndrome, including fever, chills, headache, malaise, myalgia, arthralgia, nausea, vomiting, and diarrhea, especially during the first week of therapy. Symptoms begin several hours after administration and are most prominent 8 to 24 hours after dosing. Despite more prolonged blood levels, the duration of influenza-like symptoms after peg-IFN is similar to that after conventional IFNs.30 Tolerance develops in most patients within several weeks. Febrile responses can be moderated by pretreatment with various antipyretics. Half of patients receiving intralesional therapy for genital warts experience the influenza-like illness. Intralesional IFN also causes discomfort at the injection site and leukopenia. Local reactions consisting of tenderness and erythema also occur after subcutaneous injection, and intranasal IFN causes local irritation.
Major toxicities that limit dosage and duration of IFN therapy are bone marrow suppression with granulocytopenia and thrombocytopenia; neuropsychiatric disturbance manifested by depression, anxiety, somnolence, confusion, behavioral disturbance, electroencephalographic changes, and, rarely, seizures; reversible neurasthenia with profound fatigue, anorexia, weight loss, and myalgia; thyroid dysfunction and autoimmune thyroiditis; and cardiotoxicity with hypotension, arrhythmias, and reversible cardiomyopathy. Psychiatric disturbance and depression are more common in patients with preexisting disorders but can also occur in otherwise healthy individuals. Elevations in hepatic enzymes and triglycerides and retinopathy are common.32 IFN may lead to the development of or exacerbate various immunologically mediated disorders, including sarcoidosis, systemic lupus erythematosus, psoriasis, vitiligo, lichen planus, and eczematoid skin lesions. Rare pulmonary manifestations include interstitial pneumonia, bronchiolitis obliterans, organizing pneumonia, asthma, and pleural effusion.33 Alopecia, proteinuria, renal insufficiency, interstitial nephritis, autoantibody formation, bacterial infections, and hepatotoxicity occur.34 Acute allergic reactions are rare. Patients with autoimmune chronic hepatitis, who may have false-positive enzyme immunoassay tests for anti-HCV antibodies, can experience worsening of their disease if treated with IFN.35
The adverse effects of peg-IFNs are similar to the adverse effects with conventional IFNs, although dose-related neutropenia and thrombocytopenia and injection site reactions are more common. About 50% of peg-IFN–treated patients with chronic hepatitis C develop fatigue and systemic symptoms after injections; 20% to 30% experience depression or other psychiatric reactions; and approximately 10% to 16% discontinue treatment because of adverse events, most commonly psychiatric disorders.36,37 Peg-IFN-α2a may be associated with a lesser frequency of depression.37
The development of serum neutralizing antibodies to exogenous IFNs varies with the IFN type, dosage, and route of administration but may be more common with IFN-α2a.38 Neutralizing antibodies may be associated infrequently with loss of clinical responsiveness.27 Pegylation may reduce the immunogenicity of IFNs, and anti-PEG antibody seems to be rare.
IFNs may impair fertility and alter hormone levels in women. IFN is an abortifacient in monkeys at high dosages and has been used in small numbers of pregnant women, so safety during pregnancy is not established.39 It is classified as pregnancy category C.
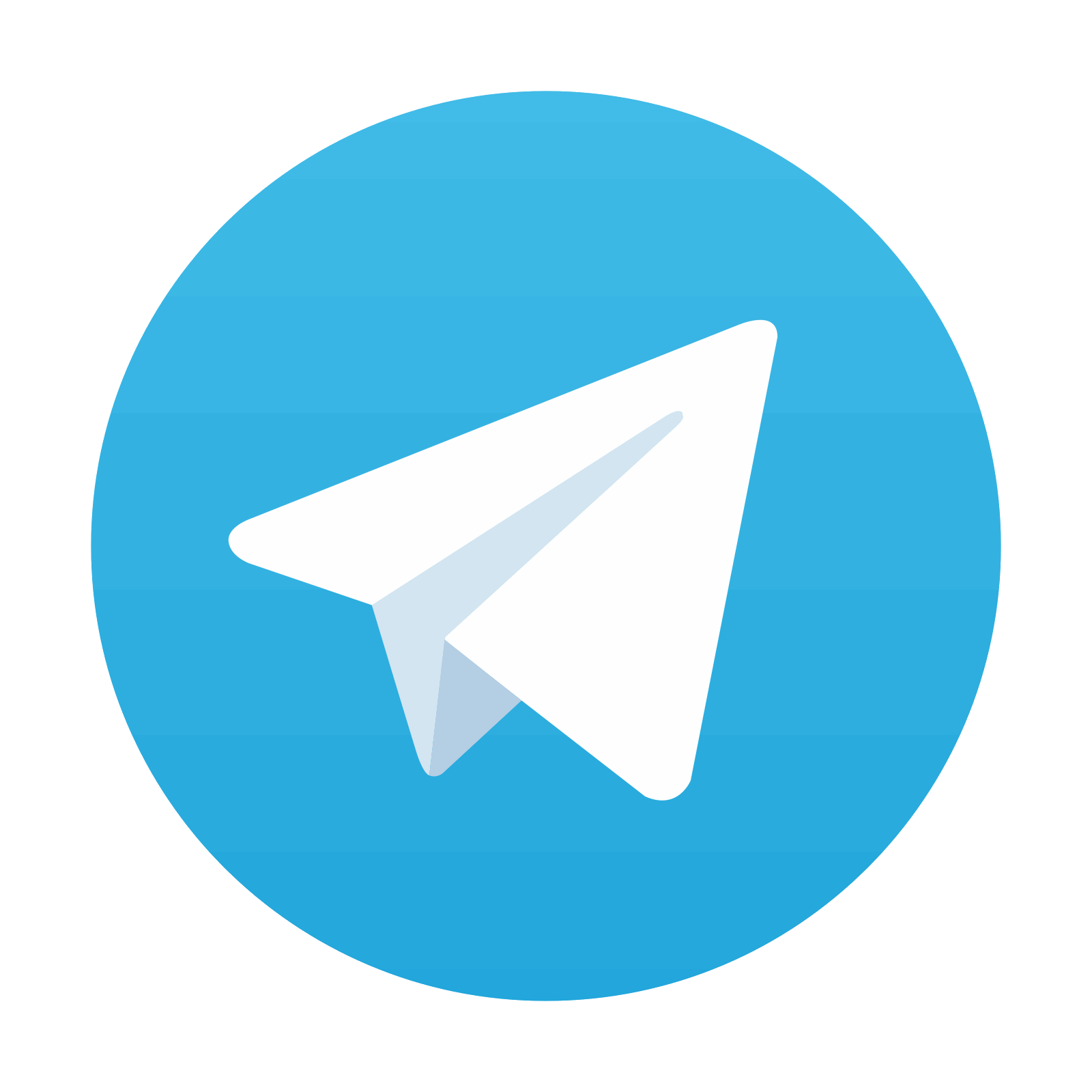
Stay updated, free articles. Join our Telegram channel

Full access? Get Clinical Tree
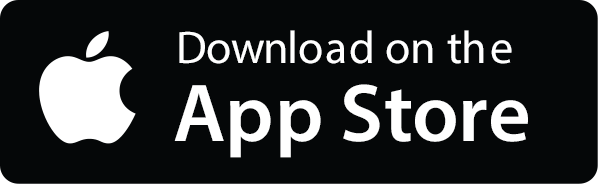
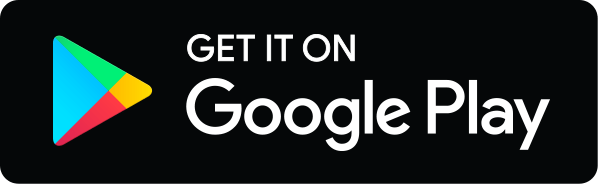