Medical Treatment for Bone Metastases
Stephen V. Liu
Christy A. Russell
MEDICAL TREATMENT FOR BONE METASTASES
Skeletal metastases are a common event in breast cancer, responsible for profound patient morbidity and representing a significant public health burden. While the precise epidemiology of bone metastases in breast cancer is elusive, they affect over 7% of all women diagnosed with breast cancer in the United States (1). Bone is the initial site of metastasis for 47% of women with relapsed breast cancer (2) and the only site for 20% of women with metastases (3). The incidence may vary by subtype: among patients whose initial metastasis is to the bone, 82% will express either estrogen receptor (ER) or progesterone receptor (PR). Nonetheless, these events are common in all subtypes, noted in 69% of women who die from breast cancer (2). Importantly, the development of bone metastases is not a fatal event. Patients with skeletal metastases can have a prolonged survival, particularly when compared to patients with visceral involvement. One study reported a median survival of 24 months for patients with only skeletal metastases compared to 3 months for patients with liver metastases. As our treatment for advanced breast cancer evolves, we are likely to see further improvements in survival, increasing the chances for patients to develop bone metastases during their lifetimes. Because of the high incidence of bone metastases and the potential for extended survival, the cumulative morbidity of skeletal involvement in breast cancer is high and the potential for impaired mobility and loss of independence highlights their clinical relevance.
The morbidity from bone metastases has been difficult to objectively quantify. While helpful, the use of pain scores and analgesic logs is subject to various potential biases. As a result, the impact of bone metastases is often quantified by the incidence of skeletal-related events (SRE). SRE definitions vary by study but operationally represent a composite of pathologic fractures, spinal cord compression with vertebral compression fracture, hypercalcemia of malignancy, requirement for radiation to bone, and necessity for surgical intervention for pathologic fractures or cord compression (4). Unfortunately, SRE are common in breast cancer. In the absence of bone-targeted therapy, the rate of pathologic fracture for patients with bone metastases from breast cancer is 35% (5) and up to 70% of patients with bone metastases will have at least one SRE by 2 years (6). SRE are associated with decreased survival in breast cancer. Patients with a pathologic fracture have a 32% increased risk of death compared to those without a fracture (7). The clinical significance of SRE, however, extends far beyond survival. SRE have been associated with significant declines in physical and functional well-being as well as overall quality of life (8). The resulting economic impact of bone metastases in breast cancer is staggering, with an estimated national cost burden of nearly $4.5 billion annually (1).
Insight into the pathophysiology of bone metastasis has facilitated recent therapeutic advances. The tropism of breast cancer cells to bone has yet to be fully explained. Transcriptome analyses of breast cancer cells predisposed to metastasize to the bone have revealed a gene expression profile that mimics osteoblasts in a process called osteomimetism. These changes may facilitate survival of these cells in the bone microenvironment (9). Under normal physiologic conditions, osteoclast differentiation is directed by osteoblasts via signaling in the receptor activator of NF-κB (RANK) ligand pathway (10). The interactions between these molecules results in normal bone remodeling: a balance between bone formation and resorption. In the setting of bone metastases, normal homeostasis is disrupted. Osteoclast activation is felt to be an early event in the development of bone metastases in breast cancer, often associated with lytic bone lesions. Dysfunction of osteoblasts has been implicated in blastic bone metastases and is relevant in breast cancer, where mixed lesions are frequently encountered.
This dysfunction may be mediated by the RANK ligand pathway, an emerging target in the treatment of bone metastases. An increase in bone resorption can compromise the integrity of the affected bone, leaving it vulnerable to the development of SRE. This process can be mediated by several factors. One contributing factor is an increase in osteoclast precursors in the presence of tumor cells, a shift potentially mediated by tumor necrosis factor α(11). Another mechanism is activation of osteoclasts by breast cancer cells, which can upregulate RANK ligand and inhibit osteoprotegerin, which normally inhibits excessive osteoclast activation. Once activated, bone resorption can elicit a chemotactic response from tumor cells, cyclically propagating the process (12). Agents targeting this pathway, however, have provided inroads in stopping this cycle and preventing some of the morbidity associated with skeletal metastases.
Bisphosphonates are a class of medications commonly used in patients with bone metastases from breast cancer. Early-generation bisphosphonates such as clodronate do not contain nitrogen. These agents are metabolized to adenosine triphosphate (ATP) analogues that cannot be hydrolyzed, interrupting multiple cellular processes including bone remodeling (13). Nitrogen-containing bisphosphonates, including pamidronate, ibandronate, and zoledronic acid, accumulate in the bone microenvironment and are released during bone resorption. They are then internalized by osteoclasts where they affect their activity and survival. One target for nitrogen-containing bisphosphonates is farnesylpyrophosphate synthase (FPPS), an enzyme in the mevalonate pathway that is responsible for the prenylation of small guanosine triphosphatases (GTPases), which are critical for osteoclast survival (14). This indirect effect of nitrogen-containing bisphosphonates is augmented by a direct effect related to production of triphosphoricacid 1-adenosin-50-yl ester 3-(3-methylbut-3-enyl) ester (ApppI), an ATP analogue that induces osteoclast apoptosis (15). The effect of bisphosphonates may extend beyond the osteoclast. Preclinical studies have shown that zoledronic acid inhibits angiogenesis in several in vitro and in vivo models (16). In patients receiving zoledronic acid, there is an early and sustained reduction in circulating levels of vascular endothelial growth factor (VEGF), a cytokine involved in angiogenesis (17). Zoledronic acid also modulates migration and adhesion of endothelial cells by targeting integrins and sensitizing cells to tumor necrosis factor (TNF)-induced programmed cell death (18). In addition, nitrogen-containing bisphosphonates prevent adhesion of cancer cells to bone, which may interfere with tumor seeding and invasion (13). Nitrogen-containing bisphosphonates may also induce cell death by activating cytotoxic T-cells in the host, though the clinical role of this effect awaits further clarification (19).
Agents targeting the RANK ligand pathway have also demonstrated activity, primarily through inhibition of osteoclast function to suppress bone resorption (20). Denosumab is a humanized monoclonal antibody targeting RANK ligand that has recently garnered FDA approval in this setting (21). Similar to zoledronic acid, the activity of denosumab is mediated by several mechanisms, including a potentially similar antiangiogenic effect (22). Multiple randomized studies have shown a decrease in SRE with the use of bisphosphonates and denosumab, which are outlined next.
First-Generation Bisphosphonates
The initial use of bisphosphonates to alter bone metabolism extends back to the 1970s when etidronate, a first-generation bisphosphonate, was used in the treatment of Paget’s disease of the bone (23). Subsequently, reports were published showing the benefit of etidronate in the treatment of both osteoporosis and hypercalcemia of malignancy (HCM). Another first-generation bisphosphonate, clodronate, was evaluated in small phase II studies followed by a randomized, double-blind, placebo-controlled trial comparing daily oral clodronate 1600 mg/day to placebo in patients with metastatic breast cancer with bone involvement (24). This study demonstrated a significant reduction in the development of HCM and vertebral fractures in patients receiving clodronate as well as a 33% reduction in all skeletal events. As a result of these trials, clodronate is approved outside of the United States for women with metastatic breast cancer and bone involvement. Despite this approval, the first-generation bisphosphonates are not optimal for long-term use. Etidronate adequately inhibits bone resorption, but also inhibits bone mineralization. Clodronate is poorly absorbed in the gastrointestinal tract and can cause local irritant toxicity, reducing the likelihood of long-term adherence.
Second-Generation Bisphosphonates
Further drug development led to the design of aminobisphosphonates, which are potent inhibitors of osteoclastic activity that do not alter bone mineralization. Examples of drugs in this class are pamidronate and alendronate. In light of the initial encouraging results of clodronate, multiple clinical trials were initiated with pamidronate for women with breast cancer metastatic to bone. Initial small studies sought to optimize the dose and schedule of intravenous pamidronate and had various endpoints including amelioration of bone pain and sclerosing of lytic bone metastases. Subsequently, two large placebo-controlled, randomized clinical trials (Aredia Protocols 18 and 19) were initiated in the early 1990s (25). Both of the trials’ eligibility criteria included metastatic breast cancer and at least one predominantly lytic bone metastasis measuring ≥ 1 cm in its greatest dimension. Women enrolled in Protocol 18 received a stable endocrine regimen for their metastatic cancer, and those on Protocol 19 received cytotoxic chemotherapy. Patients were randomly assigned to receive either pamidronate 90 mg intravenously over 2 hours or an intravenous placebo, given every 3 to 4 weeks for 24 cycles. Patients were serially evaluated for any evidence of an SRE, defined here as pathologic fracture, spinal cord compression, or hypercalcemia. The primary efficacy endpoint was the skeletal morbidity rate, expressed as the number of events per year. Although the two trials were independently performed, their common time frame and eligibility criteria allowed the investigators to combine their efficacy data.
In this trial, 367 women were randomized to pamidronate and 384 women were assigned to placebo. The addition of pamidronate to systemic therapy for advanced breast cancer resulted in a significant improvement in multiple parameters. The skeletal morbidity rate was 2.4 events per year in the pamidronate group versus 3.7 in the placebo group (p < .001). Overall, skeletal events were seen in 51% and 64% of these same groups, respectively (p < .001). The median time to first skeletal event was 12.7 months with pamidronate and 7 months with placebo (p < .001), and the time to first fracture was 25.2 months and 12.8 months for these groups, respectively (p < .003). Although this was designed as a 24-month trial, only 31.3% of the pamidronate patients and 25.8% of the placebo patients completed 24 months of treatment. Despite the compelling skeletal benefits seen with pamidronate, there was no survival difference between the two arms. As a result of these and the previous smaller trials, the FDA-approved pamidronate for the treatment of women with bone metastases in July 1996.
Third-Generation Bisphosphonates
Following the development of pamidronate, more potent third-generation bisphosphonates entered testing. The potential benefits of increasing potency are to shorten the intravenous infusion times and allow for oral formulations with greater efficacy. Three of these potent heterocyclic, nitrogen-containing bisphosphonates began to enter clinical study: risedronate, ibandronate, and zoledronic acid (or zoledronate). Zoledronate is reported to be the most potent of these third-generation bisphosphonates with respect to bone resorption without affecting bone mineralization.
Ibandronate, in both the intravenous and oral form, has been compared to placebo in the setting of bone metastases. Ibandronate 50 mg orally when compared to placebo (26) significantly reduced the risk of an SRE in 564 randomized patients (p < .001). In a second trial of similar patients, ibandronate either 2 or 6 mg intravenously was compared to placebo (27). The 2-mg dose was ineffective, but the 6 mg dose significantly reduced the skeletal morbidity rate (p < .03). However, ibandronate is not currently approved in the United States for the ancillary treatment of skeletal metastases.
A large international, multicenter, randomized, doubleblind clinical trial was performed comparing pamidronate with zoledronate in patients with bone metastases from not only breast cancer but also multiple myeloma (4). Patients participated as one of three strata: patients with multiple myeloma; patients with breast cancer receiving chemotherapy; and patients with breast cancer receiving hormonal therapy. Patients were randomized to receive pamidronate 90 mg over 2 hours in 250 mL of 0.9% sodium chloride or zoledronic acid either 4 mg or 8 mg administered in 50 mL of hydration over 5 minutes. Because of the observation of creatinine elevation in the zoledronic acid arms, two protocol adjustments were made during the conduct of the trial: the infusion duration was increased from 5 to 15 minutes with the volume of hydration increased to 100 mL; and the 8 mg zoledronic acid dose was reduced to 4 mg. Although the original trial was designed as a 13-month efficacy and safety trial, a 25-month extension phase was subsequently performed and reported. The primary efficacy endpoint of the trial was the percentage of patients in each treatment group who experienced at least one SRE during the 25-month study period. For this study, SRE were defined as pathologic fracture, spinal cord compression, radiation therapy to the bone, or surgery to the bone. Other endpoints included time to first SRE, skeletal morbidity rate, multiple event analysis, and overall survival.
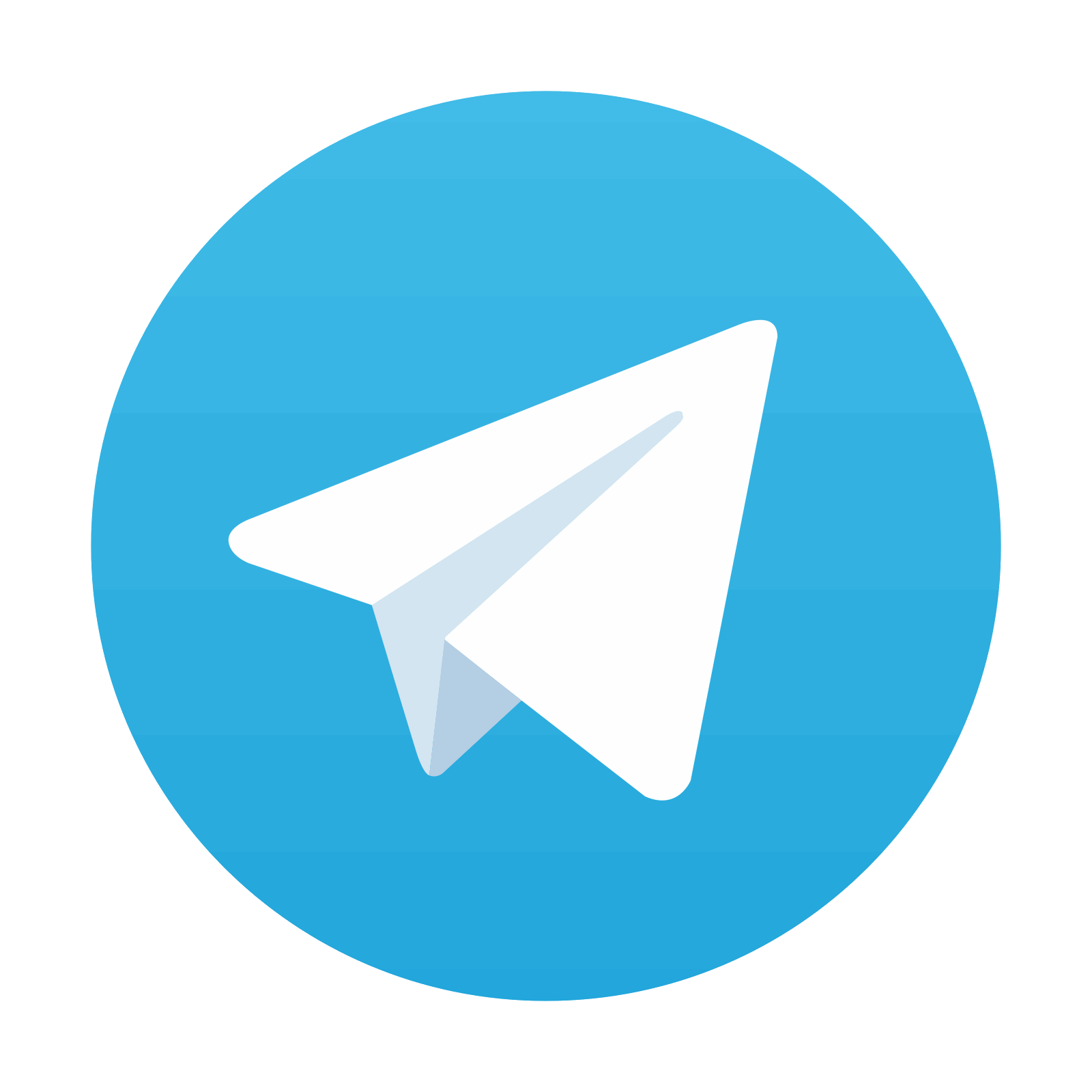
Stay updated, free articles. Join our Telegram channel

Full access? Get Clinical Tree
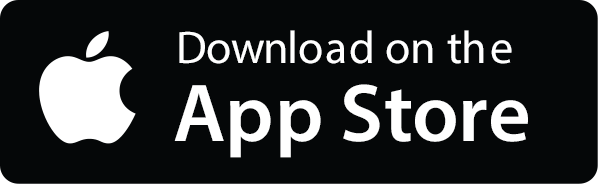
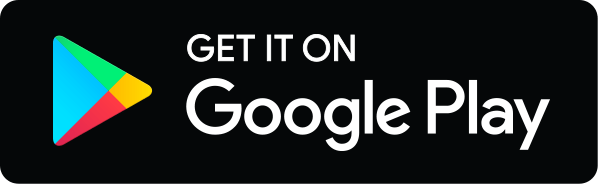