Introduction
Radiotherapy comprises the delivery of ionizing radiation, most commonly in the form of X-rays, for the treatment of malignant or benign neoplasms. Radiotherapy induces DNA damage either through direct ionization or through the generation of intermediary reactive oxygen species. Left unrepaired, this damage can lead to normal and tumor cell death via apoptosis, mitotic catastrophe, autophagy, or terminal growth arrest/senescence. Deficiencies in DNA repair, a hallmark of some cancers, can sensitize these tumors to death after irradiation, in part accounting for the favorable therapeutic ratio of radiotherapy. However, DNA repair within normal cells can be incomplete or can be overwhelmed by the dose of radiotherapy delivered, leading to the development of radiation-induced toxicities.
Types of Radiation-Related Toxicities
- ■
Radiotherapy is a local treatment and therefore adverse effects are generally expected within tissues in closest proximity to the irradiated volume. For example, radiotherapy to the pelvis may result in cystitis, enteritis, proctitis, or bone marrow suppression, but it will not typically cause toxicity in distant organs (e.g., lung). Similarly, in the treatment of pituitary adenomas, cumulative dose to the optic nerves and chiasm will be the major consideration during the treatment planning process to prevent optic neuritis or subsequent blindness. In addition to direct organ damage, there is a risk of secondary malignancy postirradiation. The relationship between the distance from the irradiated volume and risk of secondary malignancy is represented by an inverted U-shape. That is, the risk is lower in the high-dose irradiated volume, increases at intermediate distances from the target volume within a relatively lower dose region, and decreases at greater distances from the treated organ (e.g., increased risk of breast cancer after radiation for mediastinal Hodgkin’s lymphoma).
- ■
Radiation toxicities are generally categorized as acute, occurring during or within a few weeks of radiotherapy, or late, occurring months to years after treatment. Acute toxicities are mainly mediated by the adverse effects of radiotherapy upon the endothelium, leading to vascular permeability, edema, and lymphocyte adhesion and infiltration. Soon after irradiation, endothelial cells have changes in their physiological appearance and exhibit alteration in synthesis and secretion of growth factors, chemoattractants, and injury markers such as interleukin (IL)-1, IL-6, and tumor necrosis factor-alpha. This process produces an inflammatory response resulting in the recruitment and activation of neutrophils and eosinophils, which culminates in endothelial apoptosis mediated by the activation of sphingomyelinases, the generation of ceramide, and the activation of various caspases.
- ■
Late toxicities are primarily attributed to the depletion of tissue-specific stem cells and the generation of fibrosis through the excessive production of fibrocytes. Although the inciting molecular reactions take place shortly after radiation exposure, the cellular events and tissue remodeling processes take place over a period of years. Ionizing radiation also induces premature terminal differentiation of progenitor fibroblasts to fibrocytes through an imbalance of inflammatory regulators, particularly transforming growth factor (TGF)-β. TGF-β overexpression has been linked to late effects throughout many organ sites, attributed to the induction of excess collagen synthesis and the inhibition of matrix metalloproteinases. This excessive fibrosis also leads to late vascular effects including capillary collapse, thickening of basement membrane, telangiectasias, and loss of stem cell clonogenic capacity. Tissue-specific stem cells have been shown to reverse this process by normalizing proinflammatory cytokine levels, promoting revascularization, and upregulating antioxidant enzymes, assuming they remain intact after exposure to radiotherapy.
Genetic Determinants of Radiation Toxicity
Though the likelihood of adverse radiation effects is generally dependent on the cumulative radiation dose, a small number of patients develop toxicity after only mild exposure. Genetic syndromes such as ataxia telangiectasia, Fanconi’s anemia, and Bloom syndrome are known to confer significant radiosensitivity, though the exact mechanisms remain poorly understood. Systemic diseases such as scleroderma, systemic lupus erythematosus, and inflammatory bowel disease are also known to confer sensitivity to radiation, presumably due to a baseline proinflammatory state that exacerbates acute and, relatedly, late toxicities of radiotherapy. However, a majority of patients who exhibit hypersensitivity to irradiation have no identifiable causative mutation or comorbid condition, suggesting that individual sensitivity is contingent on numerous genes with variable penetrance. In addition to normal tissue sensitivity, recent studies indicate a genetic basis for the sensitivity of tumors to irradiation. Biomarkers from these and similar studies could offer an opportunity to personalize radiation doses, which can in turn lead to appropriate deescalation strategies for individual patients.
Technical Advances in Precision Radiotherapy Can Reduce Toxicity
- ■
Advancements in radiation technology have increased safety by reducing radiation doses to normal tissue. Three examples (not exhaustive) of these advances include intensity-modulated radiotherapy (IMRT), image-guided radiotherapy (IGRT), and motion management. IMRT, a highly conformal radiation technique, is characterized by two main features: intensity modulation and reverse planning. The intensity of the radiation beam is modulated with the use of multi-leaf collimators (MLCs) within the head (gantry) of the linear accelerator. Reverse planning utilizes planning software to optimize MLC positioning at each gantry angle. IGRT utilizes imaging, most commonly cone beam computed tomography (CT), at the time of radiation treatment to ensure the patient is aligned correctly and the treatment will be delivered to the desired area. Motion management (i.e., breath hold or 4-dimensional CT scanning) can further reduce the size of radiation volumes by reducing uncertainty in tumor position during treatment.
- ■
Whereas technical advancements aim to control toxicity by increasing the conformality of radiation plans, dose and fractionation are commonly altered as well in an effort to reduce side effects. Fractionation is a critical variable for managing toxicity within the irradiated field. Early radiobiological studies showed that a single fraction of radiotherapy separated into multiple smaller fractions would result in considerably less toxicity due to DNA repair and repopulation between fractions. Hence, radiotherapy has traditionally been delivered as daily treatments lasting multiple weeks. The advancements in radiotherapy detailed above permit the implementation of highly conformal plans. Treatments may now be safely delivered in as few as one to five fractions, for example, stereotactic body radiotherapy (SBRT). However, there remain many clinical scenarios where organs at risk lie in close proximity to the target volume, such that treatments may still require reduced total dose or an increase in the number of fractions in order to safely treat patients.
- ■
Particle therapy is another means used to reduce dose to normal tissue. External beam radiotherapy is most commonly delivered with megavoltage X-rays, which are attenuated as they pass completely through the patient. Charged particles such as electrons and protons possess a dosimetric benefit of delivering the majority of radiation by a certain depth, practically eliminating exit dose. Electrons are commonly used in practice but are limited by their superficial penetration. Proton therapy is becoming increasingly utilized in large centers, and randomized trials through multiple organ sites are ongoing to assess the clinical benefit of protons versus photon therapy.
Medical Therapies Alter Radiation Response
- ■
Various medical therapies have been investigated for their potential to improve the therapeutic index of radiotherapy. Radiosensitizing agents, most commonly cytotoxic chemotherapy, are given concurrently with radiotherapy in the curative treatment of many cancers throughout the body. These agents interact with radiation in additive and synergistic ways, and hence can increase tumor kill but may exacerbate toxicities. Antagonistic interactions between drugs and radiation are characteristics of radiation protectors. Amifostine is a radioprotectant that has been shown to reduce xerostomia and mucositis in head and neck cancers, though some concerns have been raised about a potential to protect tumor cells as well.
- ■
Other systemic agents have been used to treat late toxicities of radiotherapy, though data supporting their efficacy is limited. Pentoxyphylline is an antiinflammatory drug that improves blood flow to irradiated tissues and has been used in combination with the antioxidant vitamin E to treat late radiation fibrosis. Hyperbaric oxygen is thought to counteract the late effects of radiation by promoting reoxygenation and revascularization.
Conclusions
Radiotherapy is the targeted delivery of ionizing radiation for the treatment of benign and malignant neoplasms. Radiotherapy primarily exerts its effect via DNA damage. Acute vascular injury can lead to local inflammation. Excessive fibrosis and loss of tissue-specific stem cells is sine qua non of late radiation effect. Individual sensitivity to radiotherapy is generally unknown prior to radiation, and most likely a polygenic trait. Finally, toxicity can be mitigated with highly conformal treatment delivery, alterations in dose and fractionation, particle therapy, and adjunctive systemic therapies.
References
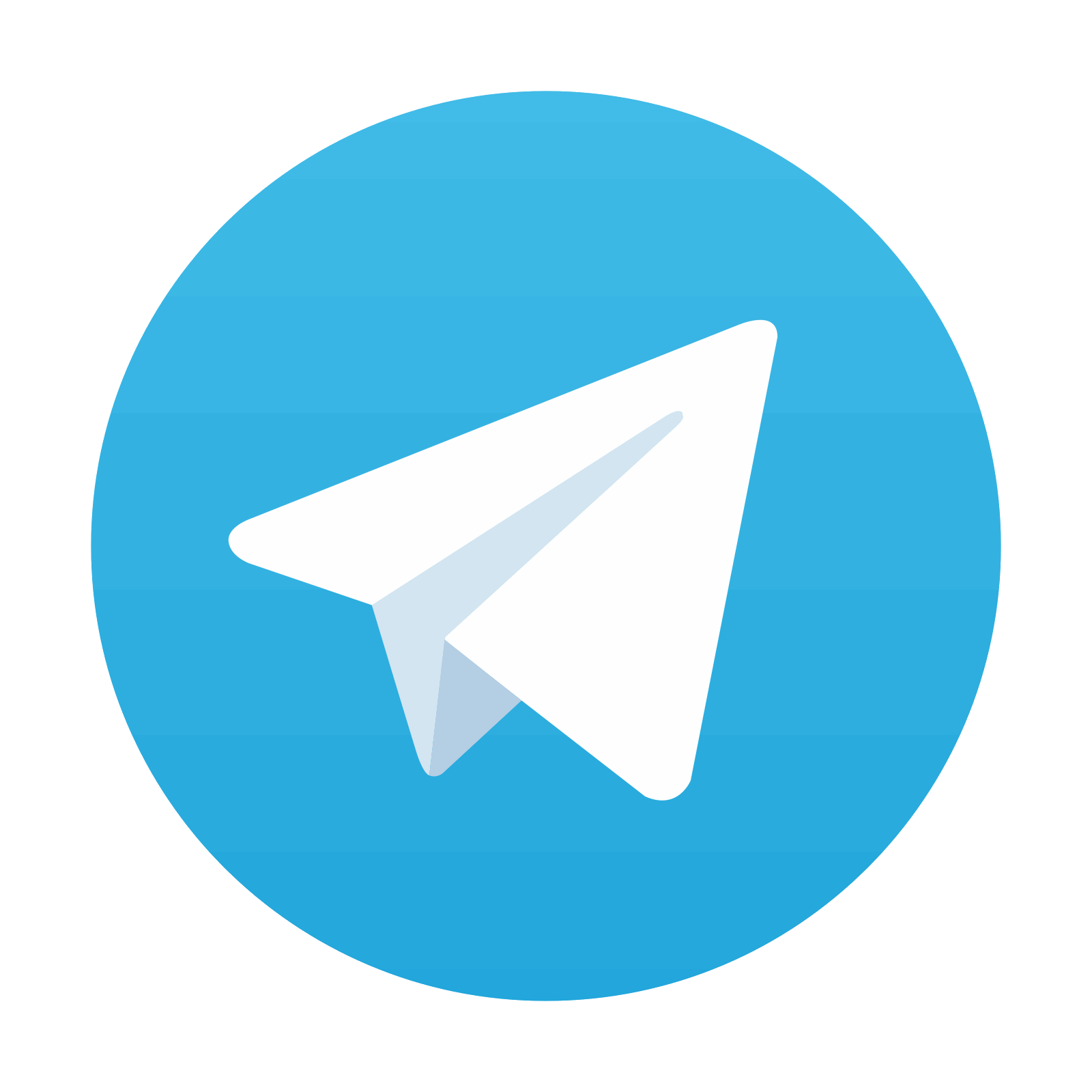
Stay updated, free articles. Join our Telegram channel

Full access? Get Clinical Tree
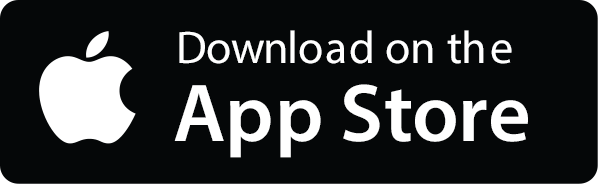
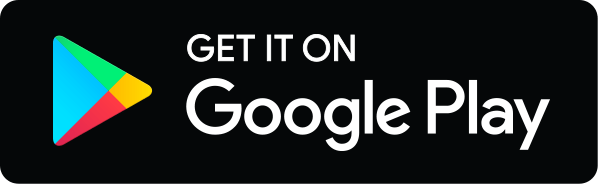
