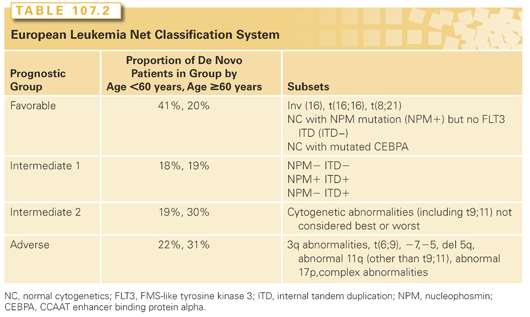
Favorable Group. The core binding factor (CBF)–related AMLs have the most favorable prognosis and constitute 10% to 15% of cases in patients under age 60 years.18 CBFs regulate the transcription of genes involved in the differentiation of normal blasts into mature progeny. CBFs contain a β unit (CBFB, located on the long arm of chromosome 16) and an α unit, one of which is known as RUNX1 (formerly AML1 and located on chromosome 21). The CBF AMLs result from translocations involving RUNX1 or CBFB. Specifically, in t(8;21) RUNX1 is fused with RUNX1T1 (formerly ETO) located on chromosome 8, whereas in inv(16), CBFB is linked with the MYH11 gene located on the short arm of chromosome 16. These abnormal CBFs exert a dominant negative effect over normal CBFs, leading to differentiation block and, in the presence of other genetic aberrations that promote survival of the affected stem cells, to AML. It is important to distinguish deletion of the long arm of chromosome 16(del16q), which does not affect CBFB, from translocation between the two chromosome 16s, t(16;16), which is quite rare but does disrupt CBFB and, unlike del16q, behaves clinically like inv(16). Eighty-five percent of cases of inv(16) or t(8;21) AML are found in those under age 60 years. Although inv(16) is most frequently associated with the FAB subtype M4Eo, 40% of the 145 cases of inv(16) AML seen at MDACC over the past 25 years have had less than 5% eosinophils. Similarly, although t(8;21) AML is most often seen in the FAB subtype M2, 30% of the 124 cases at MDACC were seen in association with other FAB subtypes.
Inv(16) AML and t(8;21) AML differ in several ways. For example, t(8;21) tends to present with lower WBC counts and is frequently accompanied by the loss of a sex chromosome (particularly the Y) or a deletion (del) of the long arm (q) of chromosome 9(del9q), whereas inv(16) is often accompanied by trisomy (+) 22 (+22), +(8), or +(21). Although both inv(16) and t(8;21) are distinguished by CR rates of approximately 90% and long remissions and survival, inv(16) AML is more apt to respond once relapse occurs; as a result, patients with inv(16) tend to live longer than those with t(8;21). The prognosis of CBF AML is quite variable (although not as variable as normal karyotype AML). Thus, long-term remissions occur in only 25% to 30% of patients aged >65 years or with high levels of mutant tyrosine-protein kinase (C-KIT) alleles.19
Worst Group. There is some debate as to the placement of patients with other abnormal karyotypes. Thus, for example, both the Medical Research Council (MRC) and SWOG cytogenetic classification systems include patients with +8,−Y in an intermediate prognostic group together with normal cytogenetic (NC)-AML (see Table 107.1). However, although the MRC considers del20q or t(6;9) AML in its intermediate group, the SWOG places these in its worst group, and other differences exist (see Table 107.1). However, there is very little debate about the great bulk of patients in the worst group: those with monosomy of chromosome 5 (−5), and/or 7 (−7), deletions (del) of the long arms of chromosomes 5 (del 5q) or 7 (del 7q) and with abnormalities of 3q. Particularly poor prognoses have been associated with complex abnormalities involving at least three to four distinct changes. In the last 5 years, some of the prognostic import of complex cytogenetics has been attributed to an association with a monosomal karyotype (MK+) defined by the presence of two or more autosomal monosomies (i.e., not involving the X or Y chromosome) or by the presence of one autosomal monosomy and a structural change, which is a translocation but no addition. MK+ patients are largely incurable with standard therapy, with median survivals of approximately 6 months. Now it appears that alterations in the tumor suppressor gene TP53, located on the short arm(p) of chromosome 17, underlie the prognostic effect of MK.
Intermediate Group. Criteria for intermediate cytogenetics differ (see Table 107.1). However, there is agreement that patients with NC-AML are the lynchpin of this group. NC-AML occurs in 25% to 40% of patients depending on age (less frequent with increasing age) and type of AML (less frequent with therapy related AML [t-AML]). As might be expected from the term intermediate cytogenetics and the frequency of NC-AML, NC-AML is associated with greater variation in outcome than any other single cytogenetic group.
NPM, FLT3 ITD, and CEBPA. Recent years have shown the ability of molecular biology to dissect this heterogeneity. Today, patients with NC-AML should routinely be tested for internal tandem duplications (ITD) in the FLT3 gene, mutations in the nucleophosmin gene (NPM1), and in the CCAAT enhancer-binding protein alpha (CEBPA) gene. Most cost effectively, this would be done once a diagnosis of NC-AML is established (the prognostic relevance of NPM, FLT3, and CEBPA being less in other patients than in those with NC-AML), and CEBPA testing would only be carried out in patients who were negative for FLT3, ITD, and NPM1. NC-AML patients with NPM1 mutations but without FLT3 ITDs and patients with a double CEBPA mutation have a prognosis with standard therapy essentially equivalent to patients with best cytogenetics. In contrast, patients with FLT3 ITDs have a prognosis more closely resembling that of patients with the worst cytogenetics. This has been codified in the widely employed four-group European Leukemia Net (ELN) Classification system (see Table 107.2). The system has been tested in patients with de novo AML that did not contribute to its development; treatment was standard. Each of the four groups were relevant in patients age <60 years, whereas the two intermediate groups were difficult to distinguish prognostically in older patients.
Secondary AML. Although many studies detailing effects of various molecular markers include only patients with de novo AML, there is little doubt that t-AML or AML following a documented abnormality in blood count for 1 to 3 months before the diagnosis of AML, often called an antecedent hematologic disorder (AHD), is associated with resistance. T-AML and AML after an AHD are collectively known as secondary AML. The association between resistance and secondary AML is independent of the tendency of patients with secondary AML to have the worst group cytogenetics (see previously). The relative effects of an AHD and t-AML remain to be established, although it has been suggested that the latter is more important.
Age. Although much of the association between older age and resistance reflects an association with the worst group cytogenetics (see previously) and/or with secondary AML, age per se probably predisposes to resistance.
Mutations Other than NPM, FLT3 ITD, and CEBPA. The ability of cytogenetics, NPM, and FLT3 ITD, along with older age and secondary AML to predict resistance has been studied using areas under receiver operating characteristic curves (AUC). An AUC of 1.0 indicates perfect prediction, an AUC of 0.5 is a coin flip (sensitivity = specificity), and an AUC of 0.6 to 0.69, 0.70 to 79, and 0.80 to 0.89 is often taken as denoting poor, fair, and good predictive ability, respectively. Walter et al.16 defined resistance in several ways: (1) no CR despite no death within 28 days of starting therapy (TRM), (2) as in 1 plus relapse within 3 months, (3) as in 1 plus relapse within 6 months, and (4) as in 1 plus relapse within 1 year. They used data from 4,565 patients treated on trials of the national cooperative groups of the UK (MRC/National Cancer Research Institute [NCRI]) and the Netherlands (HOVON) and on trials of the SWOG and MDACC in the United States. Age, performance status, WBC count, secondary disease, cytogenetic risk, and FLT3-ITD/NPM1 mutation status were each independently associated with failure to achieve CR despite no early death (primary refractoriness). However, the AUC of a bootstrap-corrected multivariate model predicting this outcome was only 0.78, indicating fair predictive ability. Removal of FLT3-ITD and NPM1 information slightly decreased the AUC (0.76). The prediction of therapeutic resistance, defined as primary refractoriness or early treatment failure as indicated by short relapse-free survival (RFS), was more difficult, with AUCs for models predicting primary refractory disease or RFS of ≤3, ≤6, or ≤12 months of 0.75/0.73 (with/without inclusion of FLT3-ITD/NPM1 data), 0.76/0.73, and 0.75/0.71, respectively.16 These data indicate that our ability to forecast resistance based on routinely available clinical covariates is limited and argues for the integration of additional disease characteristics to optimize outcome projection in AML.
Minimal Residual Disease
Although the incorporation of the pretreatment molecular information described previously will likely improve prognostic accuracy, the information obtained posttreatment will almost certainly also be relevant. Of particular note are assessments of minimal residual disease (MRD) in patients in CR by standard criteria. Given that AML relapses in most patients, such patients presumably have residual AML (MRD) even in CR. Quantification of MRD would allow broad recommendations for postremission therapy. In particular, patients with low levels of MRD that remain stable or decrease might continue on their initial therapy. In contrast, therapy might be changed in patients with high or rising MRD levels, in an effort to possibly avert subsequent hematologic relapse. Because relapse can only be diagnosed when more than 5% blasts are present in the marrow, the sensitivity of morphologic examination of the marrow for the detection of relapse is only 1 in 20. In contrast, if 30 metaphases are examined, a cytogenetic examination has a sensitivity of 1 in 30, whereas fluorescence in situ hybridization (FISH) typically has a sensitivity of 1 in 500.
MRD can be determined by polymerase chain reaction (PCR) to detect (1) leukemia fusion genes (such as those characteristic of inv 16, t(8;21), or t(15;17)); (2) mutations (e.g., in NPM1); or (3) overexpression of genes such as Wilms tumor 1 (WT1). PCR techniques generally have sensitivities of 1 in 10 (−4). An MRD can also be examined by multiparameter flow cytometry (MPFC), which relies on the identification of patterns of cell surface antigens characteristic of the patient’s AML. The sensitivity is 1 in 10 (−3)−10 (−4). Buccisano et al.20 have shown that patients with good (inv 16, t8;21) or intermediate karyotypes who have MRD detected by MPFC (MRD+) at the end of postremission therapy have outcomes resembling those in patients with FLT3 ITD+ or unfavorable karyotype AML. In turn, patients who are FLT3 ITD+ but without MRD fare better than those who are FLT3 ITD+ with MRD. In patients where both MPFC and PCR are applicable, data suggest PCR is more sensitive and specific. It remains to be established whether blood can be used rather than bone marrow, whether MRD should be assessed at multiple time points (e.g., CR, immediately after completion of post remission therapy and beyond), what levels of MRD should motivate a change in therapy and whether these levels are reproducible at different centers, and critically, whether a reduction in MRD will lead to better relapse-free or overall survival rather than MRD merely being a surrogate of refractory AML.
Treatment of Newly Diagnosed Acute Myelogenous Leukemia
Treatment of AML begins with induction chemotherapy, with the goal of achieving CR with a resolution of morphologically detectable disease and the restoration of normal blood counts. This is followed by postremission therapy to eradicate MRD. Both chemotherapy and HSCT have been utilized, and each approach has a major role in the treatment of this disease. An AML cure occurs in only a minority of patients with available forms of chemotherapy. HSCT has a greater antileukemia effect but is associated with higher TRM.
Regardless of a patient’s age, the initial goal in treating AML is to produce a CR, defined as a marrow with less than 5% myeloblasts, a neutrophil count greater than 1,000/μL, and a platelet count greater than 100,000/μL.21,22 When successful, induction therapy preferentially targets AML blasts, thus allowing normal blasts to resume control of hematopoiesis. Obtaining CR is critical because, at least in the past, only patients who do so have a chance of potential cure. An operational definition of potential cure is a remission lasting 2 to 3 years, after which the risk of relapse declines sharply to less than 10%.23 Responses less than CR are now recognized: CRp for which the criteria are the same as CR except that the platelet count can be <100,000/μL, although platelet transfusions are not being given,21 and CRi for which no blood count minima are specified, however, the marrow must be reasonably cellular (e.g., least 200 cells must be counted)21 because if such were not the case, a CRi could be produced in any patient by simply rendering the marrow hypoplastic with high doses of cytotoxic chemotherapy. It remains unclear whether CRp or CRi will prolong survival to the same extent as CR.24 It is known that CRp and CRi are more likely to be associated with MRD than CR.25 However, following treatments where CR is perhaps more likely to be associated with MRD (e.g., after use of lower intensity treatments such as azacitidine or decitabine), there may be less value to obtaining a CR.25
Prolongation of the initial response has traditionally entailed further chemotherapy, typically two to four courses of consolidation with or without subsequent prolonged lower dose maintenance. However, several randomized studies suggest that maintenance chemotherapy, although occasionally prolonging remissions, does not lengthen survival.26 These results have spurred interest in development of new approaches. In particular, recent years have seen the advent of targeted therapy. Although, as described previously, successful chemotherapy is itself selectively toxic to AML blasts, targeted therapy is taken to mean therapy that, although perhaps less toxic to AML blasts than chemotherapy, is much less toxic than chemotherapy to normal blasts and to gastrointestinal epithelium, damage to which can lead to sepsis and death. However, as noted previously, the main problem in AML is overcoming resistance, even in patients in their 70s.27,28 It is axiomatic that therapy depends on the prognosis. Accordingly, we will discuss induction and postremission therapy according to the patient’s prognostic group with standard therapy, as determined by the ELN classification system (see Table 107.2) and within each group note modifications that might be made for patients whose prognosis might differ from that of the group as a whole (e.g., older patients). The focus will be on the choice between standard therapy, now including decitabine and azacitidine, which are widely used in the treatment of older patients, and investigational treatment.
European Leukemia Net Favorable Prognosis Patients
Remission induction should typically consist of 3 days (days 1 through 3) of drugs, such as daunorubicin or idarubicin, that interact with the enzyme topoisomerase 2 (topo II) and 7 days (days 1 through 7) of the pyrimidine analog cytarabine (ara-C) at 100 mg/m2 daily; such combinations are called 3+7. A bone marrow aspiration is typically obtained approximately 14 days after the initiation of 3+7. If the marrow shows less than 10% blasts or is hypocellular, marrows are repeated, usually weekly, until it is clear that either a CR has occurred or that there has been a reappearance of AML, often best assessed using MPFC, which can distinguish an excess of normal blasts often seen during marrow recovery from chemotherapy from an excess of AML blasts. Retreatment usually takes the form of either another course of 3+7, the administration of high-dose ara-C, or of an investigational salvage regimen. SWOG data indicate a CR rate of 40% with a second course of 3+7, which competes favorably with previous investigational salvage regimens.29,30 Furthermore, the SWOG analysis could not identify patients more or less likely to respond to a second 3+7 course.29,30
Two randomized trials have shown that a daily daunorubicin dose of 90 mg/m2 in 3+7 is superior to a daily dose of 45 mg/m2 in patients up to age 65 years, particularly in patients with CBF AML.31,32 A French retrospective study suggested that 90 mg/m2 might be superior to 60 mg/m2 in patients with CBF AML.33 The MRC/NCRI in the United Kingdom is currently randomizing patients between these two doses. Although a French trial suggests that idarubicin at 12 mg/m2 daily for 3 days is superior to daunorubicin at 80 mg/m2 daily for 3 days,34 there have often been greater differences between separate randomized trials examining this issue than between daunorubicin and idarubicin in a given study. As a result, there is a consensus that these drugs are interchangeable in 3+7, with idarubicin (12 mg/m2 daily, days 1 through 3) and daunorubicin (60 mg/m2 daily, days 1 through 3) used most frequently.
The ara-C dose to be used during induction has also been the subject of debate.35 The HOVON/SAKK group randomized 431 adults age <60 years to ara-C at a daily dose 200 mg/m2 for 7 days (previously shown equivalent to 100 mg/m2 daily for 7 days36) during cycle 1 of induction therapy and 1 g/m2 twice daily on days 1 through 5 during cycle 2 of induction therapy and 429 similar patients to 1 g/m2 every 12 hours on days 1 through 6 in cycle 1 and 2 g/m2 twice daily on days 1, 2, 4, and 6 in cycle 2. Idarubicin 12 mg/m2 daily for 3 days was given during cycle 1 and amsacrine, analogous to idarubicin/daunorubicin, was given during cycle 2.5 Subsequently, patients with CBF AML in remission received 1 cycle of mitoxantrone + etoposide, whereas patients with other cytogenetic findings received an autologous or allogeneic transplant. With a median follow-up of 5 years, there was no difference between the two regimens in CR rate (80% to 82%) and, at 5 years, the cumulative incidences of relapse, relapse-free survival, event-free survival (34% to 35%), and overall survival (40% to 42%) were similar between the two regimens. Furthermore, there was no suggestion that any cytogenetic group benefited more from the higher dose ara-C regimen; in particular, 5-year survival rates were 64% to 67% in patients with CBF AML. The latter results seem equivalent to those using ara-C doses of 3 g/m2 twice daily on days 1, 3, and 5 during postremission therapy as is often done in the United States. Othus et al. have recently not found an advantage in patients with NC-AML who were NPM+/FLT3 ITD negative according to whether they received during induction standard 3+7 (in SWOG) or idarubicin + ara-C at 1.5 g/m2 daily for 3 to 4 days (at MDACC). Both groups received intermediate (1 g/m2 per dose) and high (2 to 3 g/m2 per dose) doses during postremission therapy.37 The HOVON and SWOG/M. D. Anderson results seem to indicate that there is no benefit for intermediate- or high-dose ara-C during induction. However, as indicated by a randomized Cancer and Leukemia Group B (CALGB) study, there is a benefit for higher doses of ara-C during postremission therapy after 3+7 induction in CBF AML (3 g/m2 twice daily on days 1, 3, and 5 versus 400 mg/m2 or, particularly, 100 mg/m2 daily for 5 days) or in NC-AML (with the 3 g/m2 and 400 mg/m2 doses being equivalent and both superior to the 100 mg/m2,38 although as noted in MRC’s AML 15 trial, the 3 g/m2 dose can be replaced by 1.5 g/m2.39
The duration of postremission therapy is also uncertain, although it seems that between two (based on HOVON data5) and four cycles (based on MRC/NCRI data39) are sufficient. It is likely that determinations of MRD (e.g., using PCR in CBF and NPM+/FLT3 ITD–negative AML40,41) will be important here. For example, in patients with CBF, AML MRC/NCRI randomized data suggest that a fifth cycle might be useful in patients who are MRD negative after the fourth cycle, but not in patients who are MRD positive after the fourth cycle. Patients who are MRD positive after the completion of planned postremission therapy might be candidates for further therapy. Given their low risk of relapse relative to the risk of nonrelapse mortality following allogeneic HSCT, a general consensus is that the average patient with CBF, NPM+/FLT3 ITD–negative AML, or CEBPA+ AML is not a candidate for HSCT in CR1,42 although recent data suggest that HSCT is indicated in patients with double CEBPA mutations.43
There are clearly patients in the best prognosis group who have worse prognoses than the averages reported on trials. These include CBF AML patients age 60 to 65 years,19 and those with high levels of mutant CKIT alleles.19 Reduced-intensity HSCT is an alternative in the former. A randomized American–German trial is examining dasatinib in CBF AML based on the drug’s ability to inhibit CKIT, which is mutated in 20% to 25% of these patients and overexpressed in others.44 Finally, meta-analyses of several trials randomizing patients to 3+7+/− gemtuzumab ozogamicin (GO) 3 to 6 mg/m2 once during remission induction have indicated unequivocal survival benefit due to a lower incidence of relapse in patients with CBF (and many with NC-AML) leading to attempts to reintroduce this drug into clinical practice.45,46 Fludarabine as given in FLAG-ida therapy also appears to be useful as induction and postremission therapy in CBF AML.39
European Leukemia Net Intermediate 2 and Worst Prognosis Groups
Even patients age <60 years in the intermediate 2 group have 5-year survival rates of only 20% to 25% and median survivals of less than 2 years, recalling that the ELN prognostic system does not account for the unfavorable effect of secondary AML.22 Hence, these patients, particularly those in the worst group, are considered prime candidates for clinical trials.22 Although it is not unreasonable to use 3+7 for induction and to begin the trial once in CR, it is noteworthy that differences in induction therapy can affect relapse45–47 and, accordingly, survival rates; furthermore, CR rates in the worst groups are generally <50%.27 Here, we will describe therapies that, although not likely better than standard, are in common clinical use.
Dose Intensification
Generally, intensification of an ara-C dose (e.g., to 3 g/m2 every 12 hours on days 1, 3, and 5) during postremission therapy has not improved outcomes in intermediate 2 or the worst patients,38 or if improving it “statistically,” it has improved it only minimally (e.g., p = 0.02, but from zero to only 13% 5-year survival in the HOVON trial).5
Clofarabine, Cladribine, and Fludarabine
The MRC/NCRI group has conducted two randomized trials involving clofarabine. Older patients considered fit for intensive therapy had similar outcomes whether given clofarabine + daunorubicin or daunorubicin + 10 days of standard dose ara-C.48 Older patients not considered fit for intensive therapy had higher CR rates but similar survival when given clofarabine rather than low-dose ara-C.49 A multicenter trial randomizing patients with relapsed AML also found higher CR rates but similar survival with clofarabine + high-dose ara-C compared with high-dose ara-C alone, largely due to more TRM in the clofarabine arm.50 The Polish Acute Leukemia Group reported longer survival in adults age <60 years with either de novo or secondary AML randomized to daunorubicin, ara-C (DA) + cladribine (DAC) rather than to standard induction DA.4 In contrast, a randomized MRC/NCRI trial in adult patients age <60 years found that FLAG-ida given for induction and one postremission course was associated with longer event-free survival and fewer relapses than daunorubicin + ara-C, although survival was similar due to more deaths in CR with FLAG-ida.39
Azacitidine and Decitabine
The majority of patients in the intermediate 2 and worst prognostic groups are aged >65 years. It is in these groups that azacitidine and decitabine have found greatest use. It is becoming apparent that results with these drugs used alone rival those with more intense therapies, at least in older patients. For example, Quintas-Cardama et al.51 found similar overall survival (OS) among 557 patients aged >65 years given regimens generally containing ara-C at 1 to 2 g/m2 daily and idarubicin or fludarabine +/− other agents and 114 patients given decitabine (n = 67) or azacitidine (n = 57) despite higher CR rates with the more intense therapies.51 An international study randomizing 485 patients age >65 years, one-third of whom had the worst prognosis cytogenetics and one-third of whom had secondary AML, found median survivals of 7.7 months for decitabine at 20 mg/m2 daily for 5 days versus 5.0 months for standard treatment (88% low-dose ara-C, 12% supportive care only).52 A focus on whether the difference is statistically significant (p = 0.037) loses sight of the unsatisfactory results in both arms, with patients losing >90% of their normally remaining life expectancy. An analogous trial involving azacitidine leads to the same conclusion.53 Thus, a fundamental question is whether results with either drug can be improved. One possibility is identifying patients more likely to respond either based on molecular characteristics or less active disease. Examples of the latter are patients in CR, although use of decitabine to delay relapse appears to have been unsuccessful,54 or patients with MRD after HSCT.55 A second possibility is a combination with other active drugs. A combination of decitabine with deoxyguanosine (SGI-110) increases exposure to decitabine but has not obviously improved outcomes to date.56 More fundamentally, it remains unclear whether azacitidine or decitabine, although known as hypomethylating agents, actually exert their effects via hypomethylation.57
Gemtuzumab Ozogamicin
In contrast to its utility in patients with CBF AML, meta-analyses have not indicated a role for this drug in combination in ELN intermediate 2 and the worst prognosis patients.45
Hematopoietic Stem Cell Transplantation
It is generally recommended that patients in ELN intermediate 2 or the worst prognosis groups receive HSCT in CR1 as detailed in the transplant section that follows.
ELN Intermediate 1 Prognosis Group
Here, the decision between the relatively standard approaches discussed for the favorable group and the emphasis on clinical trials stressed in the last section is more difficult. It is perhaps, at times, best to defer this decision to the patient; experience suggests that given the same prognostic data following use of standard therapies, some patients will prefer these, whereas others prefer a trial. Here, the need for new prognostic information is most acute and there might be great use in incorporating posttreatment information into the decision; examples include time or courses to CR,58 achievement of CRp/CRi rather than CR,24 or MRD measured by MPFC20 or PCR for NPM141 or WT1.59 The use of FLAG-ida might be considered because its ability to decrease high relapse rates relative to CBF AML might justify the increased incidence of death in CR associated with its use.39 Transplantation in CR1 is often suggested, although it is possible that, provided CR rates after relapse are in the 50% range (much more likely than in the ELN intermediate 2 and the worst groups), a strategy of delaying HSCT until the second CR may be reasonable.60
Although their prognosis is, on average, worse than others in the ELN intermediate 1 group, patients with FLT3 ITDs are currently included in this group (see Table 107.2). They often present with a high WBC count, usually have NC-AML, although t(6;9) AML is very specific for FLT3 ITDs, and, although having CR rates similar to others with NC-AML, are prone to relapse.61 The abnormal tyrosine kinase activity associated with FLT3 ITDs can be inhibited by various drugs (tyrosine-kinase inhibitors [TKI]). Used alone, FLT3 inhibitors have limited activity in relapsed/refractory AML, with quizartinib being the most effective, producing composite CR rates of about 50%, with >90% of the responses being CRi rather than CR or CRp. However, this response rate has allowed 25% to 35% of patients given quizartinib to subsequently receive HSCT.62 It is uncertain whether the failure of count recovery, and hence, the lack of CRs reflects concomitant quizartinib-induced inhibition of CKIT or the presence of other aberrations in the blasts that are not affected by FLT3 inhibitors. However, the latter possibility has motivated the addition of chemotherapy to quizartinib.63 Sorafenib, a commercially available FLT3 inhibitor, has been combined with 3+7 and compared with 3+7 alone in two randomized studies. In patients aged >60 years the combination produced only increased toxicity,64 but in younger patients, it was associated with longer event-free but not overall survival. In neither study were effects different in patients with or without FLT3 ITDs,65 indicating that sorafenib’s relevant mechanism of action may be independent of FLT3 inhibition and perhaps prefiguring a more general use of chemotherapy–sorafenib combinations. Even quizartinib, presumed to be a much more selective FLT3 inhibitor than sorafenib, may also have activity in patients without FLT3 ITDs.62 As predicted by various genomic studies, resistance to quizartinib is associated with the emergence of new subclones: In the case of quizartinib these contain FLT3 point mutations whose abnormal TK activity might be decreased by crenolanib, another FLT3 inhibitor.66 Although it is generally accepted that patients with FLT3 ITDs should receive HSCT in CR1, FLT3 ITDs predict for relapse even after HSCT. Accordingly, another potential role for quizartinib, and by extension other targeted agents, is the prevention of relapse after HSCT or even in patients who cannot receive HSCT.
Summary of Treatment Recommendations
Patients with favorable prognoses as specified by the ELN22 can be treated with standard therapy emphasizing higher doses of ara-C post-CR (HiDAC), although it remains unknown if the same is true in patients considered at best risk based on NPM1+/ FLT3 ITD–status rather than cytogenetics. Older patients with CBF AML and CBF patients with multiple alleles affected by CKIT mutations have worse prognoses than average CBF and thus might be candidates for (reduced intensity) HSCT or for clinical trials with dasatinib. The choice of 3+7 rather than a clinical trial for induction in the ELN intermediate 1 group depends on whether patients see their prognosis with the former as good enough to be reluctant to undertake a clinical trial, which might produce a worse outcome. FLAG-ida might be useful as induction and postremission therapy in the intermediate 1 and CBF groups and the availability of gemtuzumab ozogamicin (GO) as part of induction therapy would be useful because the drug clearly prolongs survival in CBF and in many intermediate 1 patients.46 In contrast, CR rates are often <50% in the ELN intermediate 2 and adverse groups with standard therapy making a clinical trial particularly important, recalling that the choice of induction therapy can affect the relapse rate. The general consensus is that patients in the intermediate 1, 2, and adverse groups should receive HSCT in the first CR. Results with HSCT are still sufficiently poor that clinical trials involving new transplant preparative regimens or the means to prevent post-HSCT relapse should be considered in these groups, as discussed later in this chapter. Given that many older patients will fall into the intermediate 2 or the worst ELN prognostic categories and have particularly poor outcomes with standard therapy, most such patients are candidates for clinical trials, as suggested by both ELN22 and National Comprehensive Cancer Network (NCCN)67 guidelines. It is important to recall that the major cause of treatment failure in older patients is resistance to therapy, not TRM. Thus, the use of less intense therapy is appropriate only if the chosen therapy is plausibly more effective, and not merely less toxic, than standard therapy. These recommendations are summarized in Table 107.3. Regardless of specific recommendations, any choice of therapy in older patients must refer to the observations of Sekeres et al.68 that 74% of older patients estimated that their chances of cure with 3+7 were at least 50%; in contrast, 85% of their physicians estimated this chance to be less than 10%. Although the most plausible cause of this discrepancy is a patient’s natural tendency to hope for a favorable outcome, there may also be gaps in communication between physicians and patients.
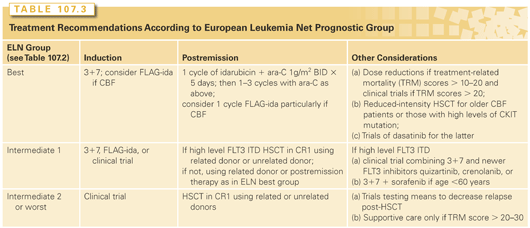
Acute Promyelocytic Leukemia
Acute promyelocytic leukemia (APL) has a unique pathophysiology and requires special considerations for treatment. In more than 95% of cases, APL results from a chromosomal translocation, t(15;17). This translocation results in a fusion protein PML/retinoic acid receptor alpha (RARα), the gene for PML being located on chromosome 15 and the gene for RARα on chromosome 17. The PML-RARα protein, an aberrant form of the normal RAR, recruits corepressor complexes that inhibit transcription of genes involved in promyelocytic differentiation.69 Predictors of APL in patients with AML are younger age, Hispanic ethnicity, and obesity.70 Although it typically has a distinctive morphology characterized by abnormal granules and multiple Auer rods, a microgranular variant exists. The possibility of this variant must be borne in mind in patients who present without morphologically typical APL but with the coagulopathy that is the clinical hallmark of the disease. The diagnosis of APL requires proof of the PML-RARα rearrangement. Although this can be obtained by demonstration of the presence of t(15;17), at least 2 to 3 days are required for test results. Immediate confirmation of the diagnosis can be made by using immunohistochemistry to demonstrate an abnormal pattern of anti-PML antibody nuclear staining consequent to the formation of PML-RARα (the PML oncogenic domains test). If doubt remains about the diagnosis, all-transretinoic acid (ATRA) should be added to 3+7.69
APL is very sensitive to daunorubicin or idarubicin and is uniquely sensitive to ATRA and arsenic trioxide. The responsiveness to the anthracyclines may reflect the absence of multidrug resistance gene (MDR1) in APL cells,71 whereas pharmacologic doses of ATRA release corepressor complexes and lead to degradation of PML-RARα.69 For many years, the initial treatment of APL has consisted of idarubicin 12 mg/m2 daily for 4 to 5 days and ATRA 45 mg/m2 until CR (AIDA regimen).69 Use of transfusions to maintain the platelet count above 30,000/μL, serum fibrinogen above 150 mg/mL, and the international normalized ratio (INR) below 1.5 is mandatory. Ten percent to 25% of patients will develop the APL differentiation syndrome (APLDS) characterized by fever, weight gain/edema, pleural effusions, and pulmonary infiltrates; the WBC is often elevated. Steroids (e.g., methylprednisolone 45 mg intravenous (IV) daily with subsequent tapering) are effective for the treatment of APLDS. The principal prognostic factor in untreated APL is initial WBC. Patients with WBC less than 10,000/μL will have CR rates greater than 90% with idarubicin plus ATRA, whereas patients with higher WBC counts will have CR rates of 80% to 85%. Almost all patients who fail to achieve CR die, which is usually related to bleeding in the brain or lung before treatment begins or in the first few days thereafter. Once in CR, patients typically receive three courses of consolidation with idarubicin 12 mg/m2 daily for 3 days and ATRA 45 mg/m2 daily on a 2-week on, 2-week off basis. With this treatment, at least 90% of patients should test negative using PCR to detect residual PML-RARα transcripts after completion of consolidation therapy; patients who are not have a 50% chance of relapse versus 5% for patients who are PCR negative. The former should receive arsenic trioxide (ATO) with or without GO72 and an allogeneic stem cell transplant if these measures do not produce PCR negativity. Although many protocols omit ara-C, allowing for the administration of higher doses of idarubicin or daunorubicin, a French trial found superior results in patients randomized to receive ara-C in addition to ATRA and daunorubicin.73 It has been suggested, however, that this outcome reflected the use of relatively low doses of daunorubicin.
Recent developments have highlighted the use of ATO for newly diagnosed rather than purely for relapsed APL. The use of ATO during consolidation in patients treated with AIDA has largely obviated the need for maintenance therapy.74 After demonstration by Estey et al.75 that ATRA + ATO could be used without the addition of chemotherapy to cure patients presenting with WBC <10,000/μL, LoCoco et al.75 randomized patients age less than 70 years old with WBC <10,000/μL between ATRA/idarubicin (AIDA) as conventionally given and ATO/ATRA as described by Estey et al.75 With a median follow-up of 34 months, 2-year event-free survival was 87% with AIDA and 96% with ATO + ATRA (p = 0.02 for superiority) and 2-year survival was also better with ATO + ATRA (p = 0.02).76 Fever, prolonged myelosuppression, and early deaths were more common with AIDA and clinically insignificant hepatotoxicity with ATO + ATRA. It is likely that ATO + ATRA will become the new standard for treatment of APL with WBC <10,000/μL. The relapse rate appears so low that there is probably no need to routinely monitor patients with PCR testing. Similarly, Australasian Leukemia Study Group data suggest that the addition of ATO to AIDA induction will improve outcomes in patients presenting with WBC >10,000/μL.77 In these patients, however, PCR monitoring in CR probably remains necessary for 2 years. Attention in APL currently focuses on the discrepancy between results, such as those described previously, in academic centers and the poorer results noted in population-based studies78; it has been proposed that the initiation of ATRA upon suspicion of a diagnosis of APL might reduce this discrepancy.78
Salvage Therapy
Salvage therapy refers to treatment given for relapsed AML or AML that has never gone into CR (primary refractory). As usual, it is critical to assess a patient’s chance for success following the administration of standard salvage therapy, using regimens such as FLAG + ida or mitoxantrone + etoposide + ara-C (2 g/m2 daily for 5 days) (MEC). A principal predictor of response to first salvage therapy is the duration of first remission; this has been true even when the therapy used for salvage contained no drugs used initially. As is often the case, consideration of several covariates to assess prognosis is useful.79 Data presented in the section on transplantation suggest that HSCT should be the first option for such patients, although the possibility of selection bias contributing to results with HSCT needs to be kept in mind. There is also uncertainty as to whether those patients in whom a donor is available should receive investigational chemotherapy before HSCT. If HSCT is unavailable or while a search for a donor is ongoing, patients should receive investigational treatments, including or not including conventional agents.
Hematopoietic Stem Cell Transplantation for Acute Myelogenous Leukemia
Stem cell transplantation provides the possibility of cure for a significant fraction of patients with AML. The approach utilizes a preparative regimen of chemotherapy or radiation with the goal of eradicating the leukemia and providing sufficient immunosuppression of the recipient to prevent rejection of the transplant. There is also an allogeneic graft-versus-leukemia (GVL) effect in which donor T and NK cells act to eradicate malignant cells that survive the preparative regimen.80 Autologous transplants can be done. Patients initially have their own hematopoietic cells collected and cryopreserved; these cells are then reinfused after high-dose therapy to restore hematopoiesis. Improvements in supportive care, histocompatibility, and tissue matching and development of less toxic preparative regimens have all increased the likelihood of success with autologous or allogeneic transplantation. This section reviews the role of HSCT in the treatment of AML in adults.
Prognostic Factors and Indications for Transplant
Outcomes of HSCT are improved if the transplant is performed earlier in the disease course, preferably in CR, due to less chemo-refractoriness and the lower likelihood of infectious or chemotherapy-related side effects. The major prognosticator is disease status at transplant (Fig. 107.1). Prognostics of patients in CR are significantly better than for those with active disease at the time of HSCT. Similarly, most of the covariates discussed in the previous paragraphs retain their influence in the setting of HSCT, such as cytogenetics and FLT3 mutational status (Fig. 107.2).81 Secondary AMLs are considered high risk and the outcome without allogeneic HSCT is generally poor.82 The presence of MRD is also a negative prognosticator prior to HSCT.83–85 A large retrospective registry study investigated outcomes of AML patients transplanted with active disease. The authors found that five adverse pretransplant variables significantly influenced survival: the presence of circulating blasts, the first CR duration less than 6 months, the use of a donor other than a human leukocyte antigen (HLA)-identical sibling, a Karnofsky score less than 90, and poor risk cytogenetics.86 It is generally accepted that fit patients with disease that is primarily refractory to chemotherapy or that has relapsed are eligible for allogeneic or autologous HSCT, as will be discussed. There is, however, controversy surrounding HSCT in first CR. Table 107.4 lists indications for allogeneic HSCT in AML.
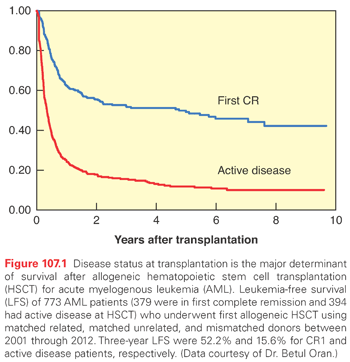
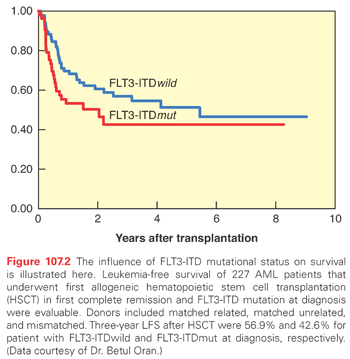
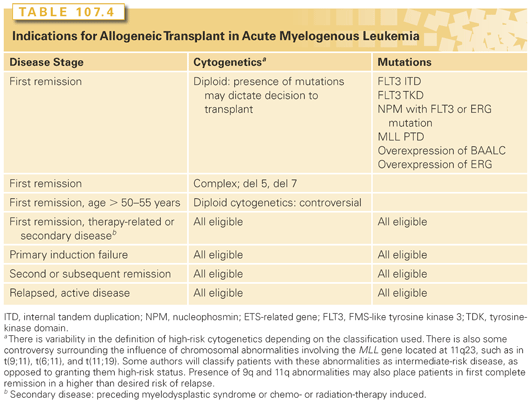
Preparative Regimens and Regimen Intensity
The preparative regimen (chemotherapy with or without radiation therapy that precedes the infusion of hematopoietic progenitor cells) provides treatment for AML and the necessary immunosuppression to prevent graft rejection of an allogeneic transplant. Preparative regimens may be chemotherapy or total body irradiation (TBI) based. A myeloablative regimen generally causes cessation of normal marrow function to a degree that requires autologous or allogeneic hematopoietic cell transplant. Hematopoietic transplantation allows for the use of stem cell toxic agents, which eradicate both normal and leukemic stem cells; hematopoiesis is restored by normal stem cells present in the transplant. Reduced intensity regimens were developed to decrease regimen related toxicity; this approach relies on the immune GVL effect to eradicate residual disease that would survive the preparative regimen. Conditioning utilizes either a lower dose of alkylating agents or low doses of radiation. The advent of these reduced intensity preparative regimens has allowed for the use of hematopoietic transplantation in older patients and in those with comorbidities, which would make them ineligible for myeloablative therapy. This has been an important advance, because the peak incidence of AML is in the 6th and 7th decades of life. Still, there is controversy regarding which patients should receive myeloablative versus reduced-intensity preparative regimens. The safety of myeloablative regimens is improving, and their use for fit patients aged 55 to 65 years is now an attainable goal. Randomized clinical trials will be necessary to resolve this question of regimen choice and intensity. It is recommended that older patients with AML be treated within clinical trials.
Myeloablative Regimens. The most commonly used myeloablative regimens are cyclophosphamide-TBI (Cy-TBI),87 busulfan-cyclophosphamide (BuCy),88 and, more recently, busulfan and fludarabine.89 The development of BuCy as an alternative to TBI-containing regimens led to ongoing debates as to which conditioning regimen is the best for the treatment of myeloid leukemias with HSCT. Two randomized studies were performed. The Nordic group compared Cy-TBI to BuCy in a heterogeneous group of patients with AML, lymphoid malignancies, and CML receiving allogeneic HSCT.90 Results indicated improved Disease free survival (DFS) among advanced stage disease patients treated with Cy-TBI, along with an increased rate of long-term complications for recipients of BuCy. Similarly, Blaise et al.91 studied young patients with AML in first CRs and concluded that Cy-TBI and allogeneic HSCT produced better disease-free and overall survival than BuCy. The major pitfall of these reports is the use of the oral busulfan formulation, which results in unpredictable plasma levels. The Center for International Bone Marrow Transplant Research (CIBMTR) compared the use of oral or intravenous (IV) BuCy and Cy-TBI in 1,230 AML patients who received allogeneic transplantation in first remission. There was less nonrelapse mortality and relapse 1 year after transplant, and improved leukemia free survival (LFS) in recipients of IV busulfan compared to TBI regimens.92 A prospective cohort study tested the hypothesis of noninferiority of survival after IV busulfan ablative regimens, compared to TBI-based regimens for myeloid leukemia patients (n = 1,025 versus n = 458, respectively). Two-year probability of survival was better after IV busulfan treatment (56% versus 48%; p = 0.03).93 Another retrospective registry analysis performed with the European Cooperative Group for Bone Marrow Transplantation (EBMT) database found IV BuCy to lead to similar outcomes to CyTBI for the treatment of AML in first CRs.94 Exchanging cyclophosphamide for the nucleoside analog fludarabine may increase the safety margin of the regimen.95 Fludarabine appears to increase alkylator-induced cell killing by inhibiting DNA-damage repair and is highly immunosuppressive. A preparative regimen using fludarabine and single daily dosing of IV busulfan has been studied in multiple centers and appears to be effective and potentially less toxic than the commonly used BuCy regimen.
Reduced Intensity Conditioning Regimens. Given the relative insensitivity of AML to the GVL effect, chemotherapy or radiation intensity is an important component of HSCT. There is a trade-off between nonrelapse mortality (NRM) and dose intensity, which may negate the decrease in relapse rates associated with higher dose regimens. The CIBMTR collects information on most transplants performed in North America. The most commonly used reduced-intensity regimens (for all indications) as reported to the CIBMTR are fludarabine combined with low-dose TBI,96 cyclophosphamide,97 busulfan,98 melphalan,99 or other drugs. Antithymocyte globulin or alemtuzumab are commonly added to the regimen for in vivo depletion of T cells in order to reduce the risk of graft versus host disease (GVHD). The use of in vitro or in vivo T-cell depletion remains controversial in the setting of myeloablative and reduced-intensity conditioning, but the perceived increased risk of leukemia relapse100 is challenged by reports indicating similar or improved outcomes with ex vivo T-cell depletion.101 The fludarabine–melphalan reduced-intensity regimen has been used at the MDACC for approximately 15 years, and long-term follow-up demonstrates its ability to induce durable disease control in a significant fraction of AML patients.102 Likewise, the Seattle group experience of treating AML in first CRs (median age of 59 years) with a nonmyeloablative, low-dose TBI-based regimen achieved a low TRM rate.103
Transplants for AML in First Complete Remission. Although multiple phase 2 studies have indicated that both allogeneic and autologous transplants benefit subsets of patients with AML in first CRs, the conclusions from randomized trials are less clear.104–107 The majority of studies indicate that allogeneic and autologous transplants are associated with lower relapse rates but also with higher mortality rates in CR (especially allogeneic HSCT). Nonrelapse mortality (death of all causes for patients in CRs) traditionally has reduced the benefit of less relapses after allogeneic HSCT, and most randomized studies did not show statistically significant advantage in survival (Table 107.5). The debate is far from resolved, given that newer preparative regimens (as discussed previously) and improvements in supportive care are reducing nonrelapse mortality significantly. A common feature of these large clinical trials is the fact that, although most patients assigned to chemotherapy will complete the intended treatment, a significant minority of those assigned will not receive an allogeneic or autologous HSCT.
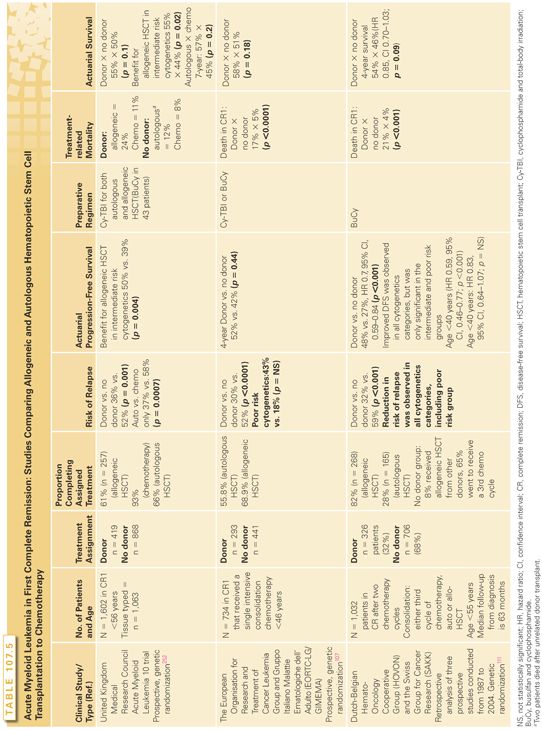
Most studies indicate that the use of consolidation chemotherapy prior to myeloablative or reduced intensity allogeneic HSCT in first remission does not influence survival after transplant.108. In the autologous HSCT setting, however, another retrospective registry analysis concluded that consolidation may improve transplant outcomes. Leukemia-free and overall survival rates were improved for those who received consolidation, but the number of consolidation cycles (one versus two) and the cytarabine dose did not significantly affect transplantation outcomes.109
The European Organization of Research and Treatment of Cancer—Leukemia Group/ Gruppo Italiano Malattie EMatologiche dell’Adulto (EORTC-LG/GIMEMA) AML-10 trial set out to compare autologous and allogeneic HSCT for patients in first CRs.107 After one course of consolidation chemotherapy, patients younger than 46 years with an HLA-identical sibling donor were assigned to undergo allogeneic HSCT, whereas all others were to undergo autologous HSCT. In the donor group, 68.9% received an allogeneic HSCT, whereas in the no-donor subset, an autologous transplant was performed in 55.8% of those eligible. DFS was improved in the former group, whereas the death rate in first CR was decreased in the latter (17.4% versus 5.3%). OS was similar, but DFS was improved for patients with poor prognosis cytogenetics after allogeneic HSCT, especially for patients aged 15 to 35 years. Instead, however, the MRC study indicated an advantage in survival for allogeneic transplanted recipients with intermediate-risk disease.105 In both the EORTC/GIMENA AML-8 and the MRC AML-10 trials, the relapse-free survival was improved with autologous HSCT when compared to chemotherapy alone, without an OS benefit. The likelihood of achieving another remission after relapse was higher in the chemotherapy arms, which led to the similar survival.
Jourdan et al.110 reported the long-term follow-up results of four studies investigating postremission consolidation strategies conducted by the Bordeaux Grenoble Marseille Toulouse (BGMT) cooperative group in Europe. The donor group (i.e., with the HLA-identical sibling) comprised 182 patients (38% of those who had achieved a first CR); allogeneic HSCT was performed in 171 patients (94%). The no-donor group had 290 patients, of which 62% received an autologous HSCT. The intent-to-treat analysis (donor versus no donor) showed a statistically nonsignificant advantage in overall 10-year survival probability of 51% versus 43% for the donor group. Patients were stratified using the covariates WBC count at diagnosis, the FAB subtype, the cytogenetic risk, and the number of induction courses to achieve a CR. An intermediate risk group benefited from allogeneic HSCT, with longer survival, whereas small numbers precluded definitive conclusions in other subgroups.
Cornelissen et al.111 updated the follow-up and consolidated the results of three consecutive studies sponsored by the Dutch-Belgian Hemato-Oncology Cooperative Group (HOVON) and the Swiss Group for Cancer Research (SAKK) between 1987 and 2004. These studies investigated myeloablative allogeneic HSCT for young patients with AML in first CR, comparing results in patients with a transplant donor identified versus those with no donor who received conventional chemotherapy. Subsets of patients in the no-donor subgroup were eligible to receive autologous HSCT. The initial sample size was 2,287 patients. Patients younger than 55 years, without FAB M3 disease, who achieved a first CR after a maximum of two cycles of chemotherapy and then received consolidation treatment were considered eligible for allogeneic HSCT (n = 1,032, 45% of the cohort). The donor group comprised 326 patients (32%), and the no-donor group comprised 599 patients (58%). In the donor group, 82% went on to an allogeneic transplant. Patients in the donor group had fewer relapses and longer DFS. Patients with a donor younger than 40 years of age and with an intermediate- or poor-risk profile had statistically significantly improved DFS.
It is likely that some subsets of patients in first CR will benefit more from HSCT than others. Patients older than 55 years have an extremely poor outcome with conventional chemotherapy. Historically, they have not been eligible for myeloablative allogeneic transplantation because of concern for toxicities, but this group may benefit from nonmyeloablative allogeneic HSCT. A feasibility study demonstrated a marked improvement in relapse-free survival with nonablative allogeneic transplants in elderly patients with AML in first CR compared to patients without a donor who received standard chemotherapy.112 The incorporation of comorbidity indexes to estimate NRM risk have contributed to patient selection as well, and the proportion of patients ultimately receiving allogeneic HSCT is increasing, especially in reference centers.113,114
High-dose ara-C–containing chemotherapy regimens, on the other hand, have improved the outcome of young patients, particularly for patients with good risk cytogenetics (t[8;21] and inv16); the consensus is to not perform allogeneic HSCT in these patients while in first CR. An HLA-compatible family donor (an HLA-identical sibling or a one-antigen mismatch relative) is available in less than 35% of the cases. Unrelated donor transplants that are HLA matched using high resolution methods for the HLA-A, -B, -C, and -DR loci fare comparably with matched sibling donors.115 Interestingly, in the unrelated donor setting, it is possible that donor-derived NK cell reactivity could reduce relapse rates. A study of 1,277 patients with AML that received unrelated donor HSCT indicated that the activating KIR2DS1 gene provided an HLA-C–dependent prevention of relapse, whereas the donor KIR3DS1 gene was correlated with reduced mortality. This information may guide donor choice aiming at relapse prevention.116
Autologous HSCT has been proposed and extensively investigated as an option for first CR consolidation (see Table 107.5). The role of autologous HSCT in first CR, however, remains controversial. Phase 2 and 3 studies demonstrate that some subgroups of AML patients may benefit from autologous HSCT, with a reduction in relapse and improvement in leukemia-free survival. Patients with unfavorable cytogenetics do not appear to benefit, and allogeneic transplant is their preferred option. AML patients, however, are very often poor mobilizers of stem cells, possibly due to AML and treatment-related changes on the normal stem cell pool. Reinfusion of leukemia stem cells contained in the autologous graft is a possibility, and gene marking studies have demonstrated that malignant cells contained in the autograft may contribute to systemic relapse.117 Purging describes the various ex vivo procedures that have been used to eliminate these residual leukemic cells from the graft.118 Preclinical studies have suggested that this strategy significantly reduces the number of clonogenic progenitors. Chemotherapeutic agents have been extensively used for ex vivo purging, but none of the purging techniques have been tested in a randomized fashion, and conclusive evidence of a benefit for purged grafts is lacking.
Most studies of autologous HSCT enrolled patients younger than 50 years old. However, autologous HSCT transplants have also been investigated for patients older than 60 years. The EORTC-GIMEMA AML-13 trial proposed the collection of peripheral blood stem cells after induction therapy with mitoxantrone, cytarabine, and etoposide (MICE) with or without G-CSF, and consolidation chemotherapy with idarubicin, cytarabine, and etoposide (mini-ICE). Patients aged 61 to 70 years with good performance status were eligible. Of 61 patients, 54 (88%) had peripheral blood stem cells harvested, but only 35 patients received autologous HSCT (57%). The 3-year disease-free and overall survival rates were 21% and 32%, respectively. The authors considered this a negative study that exemplified the limitations of dose intensification for patients with AML in this age range.119
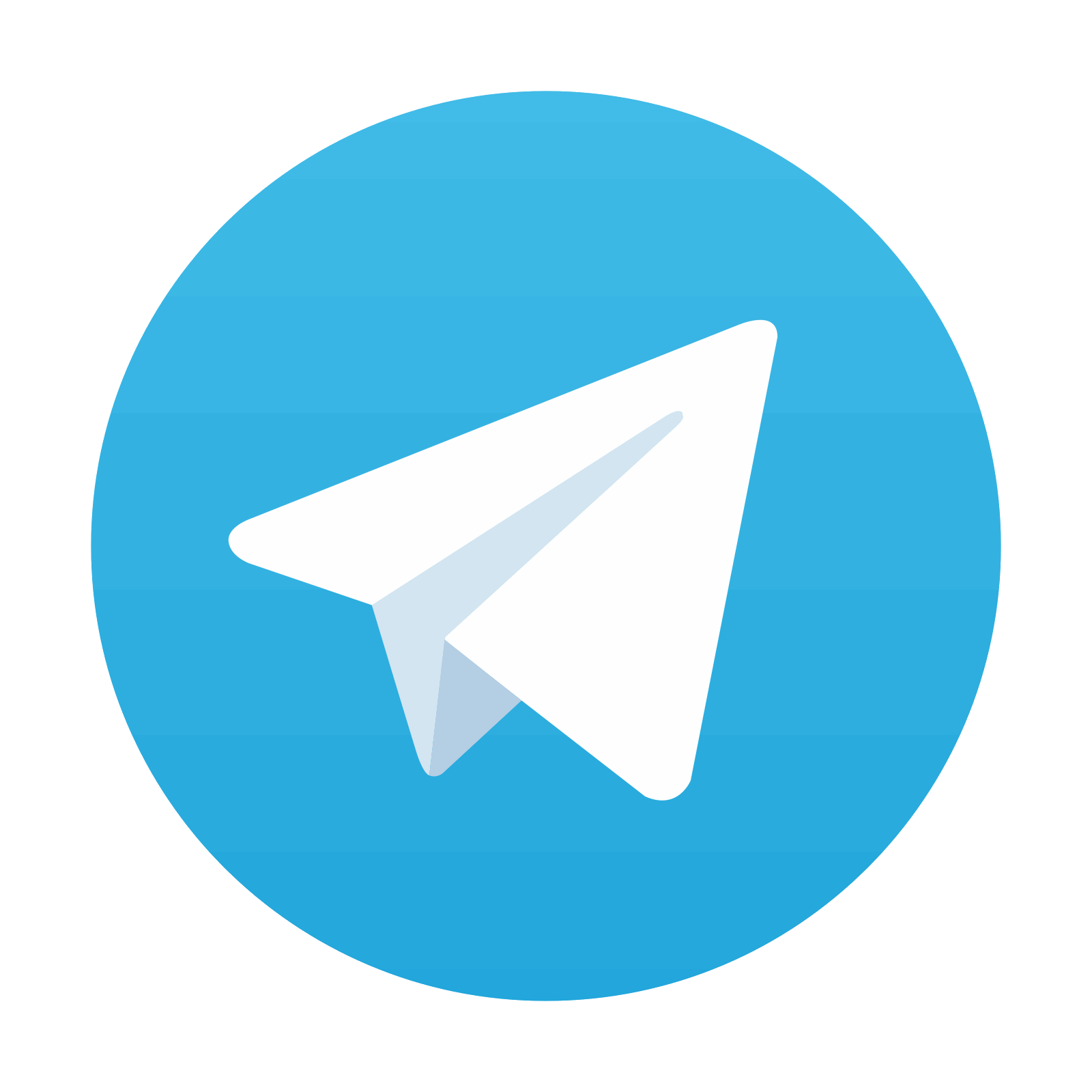
Stay updated, free articles. Join our Telegram channel

Full access? Get Clinical Tree
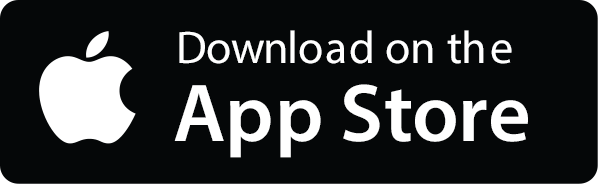
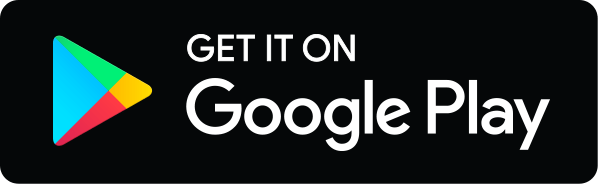