that may be fulfilled by a second chemotherapeutic agent, by radiotherapy, by a disease-related abnormality in metabolism or immune function, or by a somatic mutation or deletion that increases the risk of malignancy. Chemical carcinogens show a diversity of structures but share important metabolic features. Most are inert and require microsomal metabolic activation to positively charged (or electrophilic) intermediates that react with DNA bases. This characteristic of carcinogens is shared by certain antineoplastic agents such as cyclophosphamide, procarbazine, and mitomycin C and is essential in the antineoplastic action of these drugs. Other agents, such as L-phenylalanine mustard and nitrogen mustard, do not require metabolic activation to form alkylating species. Carcinogenicity has also been ascribed to ionizing irradiation, which produces free radicals, such as superoxide or hydroxyl radicals. A number of antitumor drugs have the same ability to promote formation of reactive oxygen intermediates, such agents include those that possess quinone functional groups (doxorubicin hydrochloride and plicamycin [mithramycin]) and those that bind electron-donating heavy metals (such as bleomycin sulfate and hydroxyurea).
TABLE 42.1 Oncogene activation | ||||||||||||
---|---|---|---|---|---|---|---|---|---|---|---|---|
|
TABLE 42.2 Results of testing antineoplastic agents in three systems for carcinogenicity | |||||||||||||||||||||||||||||||||||||||||||||||||||||||||||||||||||||||||||||||||||||||||||||||
---|---|---|---|---|---|---|---|---|---|---|---|---|---|---|---|---|---|---|---|---|---|---|---|---|---|---|---|---|---|---|---|---|---|---|---|---|---|---|---|---|---|---|---|---|---|---|---|---|---|---|---|---|---|---|---|---|---|---|---|---|---|---|---|---|---|---|---|---|---|---|---|---|---|---|---|---|---|---|---|---|---|---|---|---|---|---|---|---|---|---|---|---|---|---|---|
|
time through the latency period before the occurrence of a second malignancy. Such an analysis allows a reasonable estimate of the carcinogenic effects of a single therapy, but its use when comparing two treatments results in bias against the treatment that leads to a longer survival. Many studies compare the risk of cancer in the treated group with that of an age-matched cohort in the normal population to determine an RR. For a tumor that is uncommon in this age-matched population, a 5-fold to 10-fold increase in the RR sounds impressive but may only translate into a problem for fewer than 1% of patients who received therapy. On the other hand, small increases in RR for the more common solid tumors such as lung or breast cancer translate into a much greater problem in terms of absolute risk. One method that is useful in determining the overall impact of a secondary cancer in a population is to describe it in terms of the number of new cancers that occur per 10,000 patients treated.
TABLE 42.3 Categorization of antineoplastic agents according to carcinogenic risk in humans | ||||||||||||||||||||
---|---|---|---|---|---|---|---|---|---|---|---|---|---|---|---|---|---|---|---|---|
|
risk of developing second primary cancers for patients previously treated for childhood malignancy. The SIR in this study was 3.3.35 Further analysis from the childhood cancer survivor study reveals that survivors of childhood cancer are 15.2 times more likely to die of a subsequent cancer than match controls from the general population. The risk factors for death due to subsequent malignancy include previous therapy with alkylating agents, epipodophyllotoxins, or radiotherapy.36
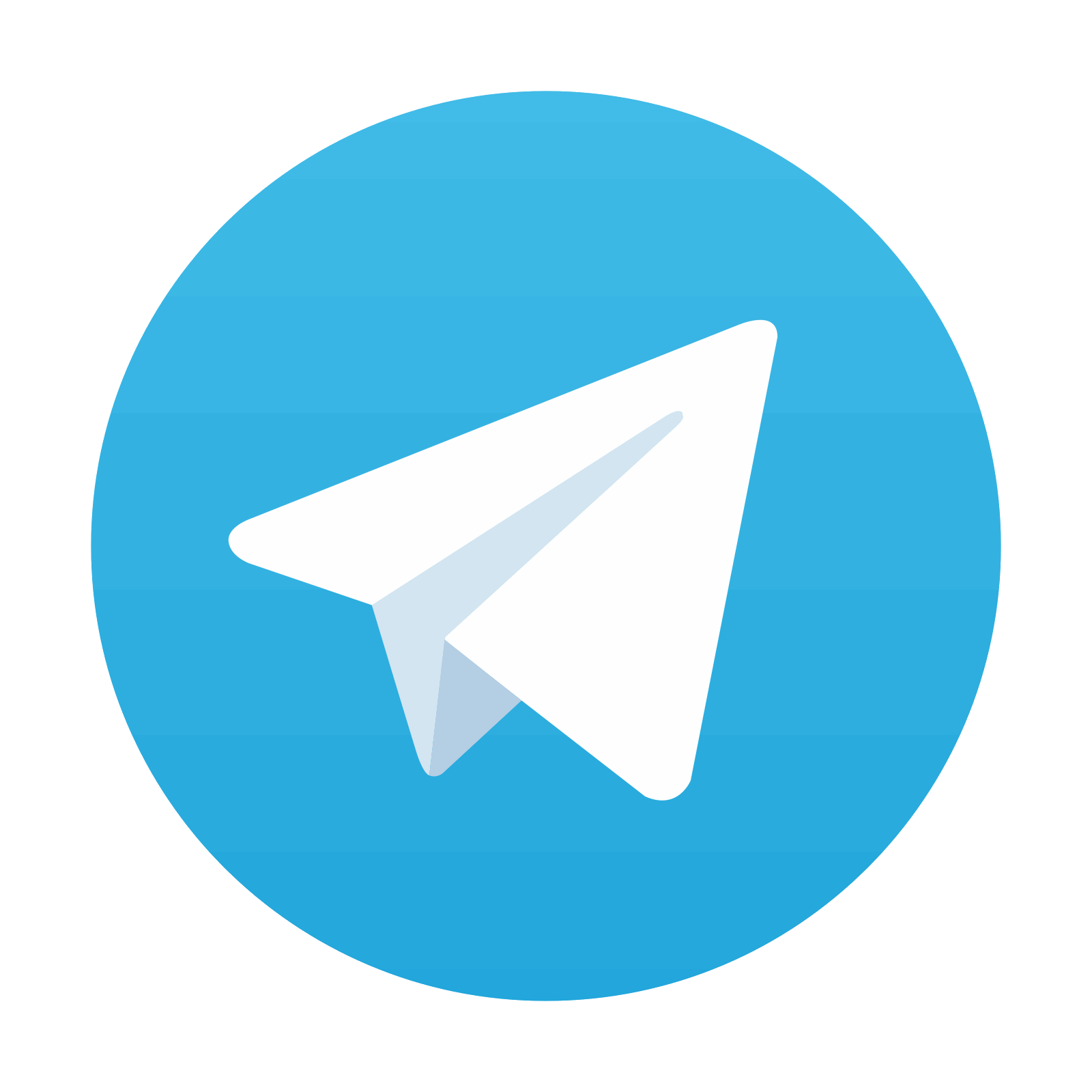
Stay updated, free articles. Join our Telegram channel

Full access? Get Clinical Tree
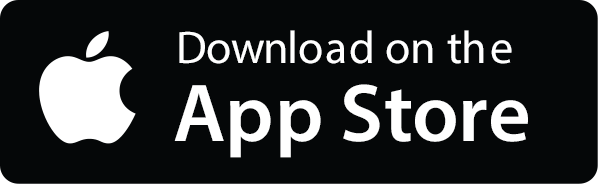
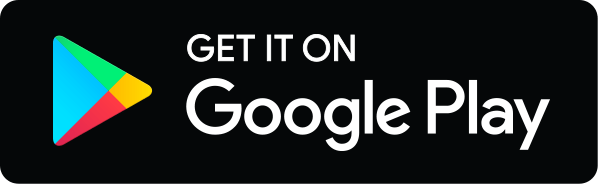
