Laboratory methods in pediatric endocrinology
Donald Walt Chandler, PhD, Dennis J. Chia, MD, Jon Nakamoto, MD, PhD, Kelly Y. Chun, PhD, Samuel H. Pepkowitz, MD and Robert Rapaport, MD
Introduction
Hormones circulate at remarkably low levels, yet relatively small perturbations can distinguish health from disease states. As a frame of reference, the major extracellular cation, sodium, circulates at approximately 140 millimolar (10−3 mol/L), whereas normal glucose levels are in the 4 millimolar range. In contrast, relatively abundant hormones such as cortisol have concentrations in the low micromolar range (10−6 mol/L), and others such as growth hormone and thyroglobulin are only in the low picomolar range (10−12 mol/L) (Figure 4-1). Given this thousand- to billion-fold difference, it is not surprising that assays using chemical analysis are not applicable in the measurement of hormones. To reach an appropriate level of sensitivity and specificity at these concentrations of an analyte, two types of assays are generally employed: immunoassays and tandem mass spectrometry (MS/MS) coupled with chromatography.
FIGURE 4-1 Relative concentrations of selected hormones. Concentrations are rounded for illustration purposes.
Endocrine hormone assays have applied improved technology to evolve from colorimetric tests and radioimmunoassay (RIA) with monoclonal antibodies, sensitive detection techniques, extensive automation, and, increasingly, tandem mass spectrometry. With automation, the sudden availability of platforms with a menu of commonly ordered hormone tests has rapidly broadened access of endocrine testing so that currently more than 3000 laboratories offer a wide range of endocrine tests.1 In most general laboratory settings, one of five common immunoassay analyzers is used for hormone detection (Table 4-1). These analyzers consolidate more than 20 to 30 hormone or other assays on a single platform that can operate in batch or random access mode with unattended capability, timely turnaround, and cost-effective throughput. Despite these practical advantages, there are some challenges to consider. In many laboratories utilizing such high-throughput instruments, appropriate in-depth knowledge of endocrinology and the technical aspects of the analytic systems is limited, such that troubleshooting is often outsourced to device manufacturers whose primary focus may not be on analytic accuracy. Furthermore, platforms of consolidated analyzers may not be appropriate for specimens from pediatric-age patients for several reasons: available normal reference range data may be inadequate; the assay may perform well at a normal adult range but be unsatisfactory in the pediatric range; or the failure to remove cross-reacting contaminants by extraction and chromatography steps may lead to falsely elevated results.2–4 Similar pitfalls may be encountered as mass spectrometry technology is expanded to more laboratories.
This chapter is intended as a reference work for pediatric endocrinologists in training or in practice to assist in the requisition and interpretation of laboratory tests to facilitate optimal clinical diagnosis and management. It will first cover the two main assay formats used to measure hormone levels, namely (1) antibody-based immunoassays and (2) mass spectrometry. Later sections discuss preanalytic variables and then validation techniques, both analytic and clinical. Dispersed throughout the chapter are short clinical vignettes that illustrate how a strong foundation in laboratory methods can impact clinical care. This chapter is not intended to provide a comprehensive review of all laboratory assays. Principles of molecular endocrinology testing are discussed in Chapter 2.
Hormonal assays
Immunoassays
Immunoassay is an important methodology in the endocrine laboratory. Understanding the basic principles involved allows specialists to (1) identify when a particular immunoassay is or is not appropriate for a particular clinical scenario, (2) anticipate potential physiologic and technical issues affecting the interpretation of laboratory results, and (3) understand how to work with the clinical laboratory to investigate unanticipated or clinically discordant test results.
Competitive immunoassay versus immunometric (sandwich) assay
There are two main immunoassay formats relevant to endocrinology laboratory testing, and understanding some of the basic differences between these formats helps both interpretation of results and troubleshooting of unexpected situations.5 The first class of assays is termed competitive, with the classic radioimmunoassay (RIA) as the archetypal example. A primary antibody against the substance (“analyte”) of interest is added to the patient’s sample, together with a radiolabeled version of the analyte (“tracer”) that competes with the endogenous analyte for binding to the primary antibody (Figure 4-2, upper panel). After a sufficiently long incubation time, the primary antibody is precipitated using a second anti-IgG antibody, polyethylene glycol, or (most commonly nowadays) by using primary antibody attached to a solid support such as a bead, which allows a simple centrifugation step to collect the primary antibody. Any unbound tracer or analyte is washed away, and the amount of tracer in the precipitate is then quantified.
FIGURE 4-2 Comparison of competitive versus immunometric immunoassays. In competitive immunoassay (top), the labeled tracer (signal conjugated analyte) and unlabeled (analyte) compete to bind specific antibody sites. In the two-site method (bottom), analyte of interest is sandwich between the capture and signal antibodies.
The second class of immunoassays is termed immunometric, with the most important example being the noncompetitive, so-called sandwich assay. Typically, as shown in Figure 4-2 (lower panel), an antibody attached to a solid support (plate, wall of a tube, or a bead) is used to capture the analyte of interest, followed by the addition of a second, labeled antibody that binds to a different site on the analyte, creating an antibody-analyte-antibody sandwich. After unbound detection antibody is washed away, that which remains generates a radioactive (immunoradiometric [IRMA]), chemiluminescent (immunochemiluminometric [ICMA]), colorimetric (enzyme-linked immunosorbent assay [ELISA]), or fluorescent (immunofluorescence assay [IFMA]) signal, depending on the label chosen.
The immunometric assays offer certain inherent advantages over competitive immunoassays. First of all, the fact that two antibodies are involved, each binding to a separate epitope on the analyte, greatly increases analytic specificity, including the ability to select very specific isoforms of an analyte.6 For example, one antibody can be directed at the N-terminus of a peptide, whereas the other antibody can be directed at the C-terminus, ensuring that only full-length peptide is detected. A second advantage is that immunometric assays tend to be more analytically sensitive than their competitive assay counterparts.7 This arises from the fact that the sensitivity of a competitive immunoassay largely depends on the affinity of the antibody used in the assay—and developing very high affinity antibodies is not an easy or predictable task. In contrast, the sensitivity of an immunometric assay can be improved by use of a higher activity signal, which is more easily detected even when analyte concentrations are very low. As signal detection technology continues to improve, increasingly sensitive immunometric assays can be developed. A third advantage of immunometric assays arises from the ability to use monoclonal antibodies, which can be more easily produced in quantity and have more predictable characteristics than polyclonal antibodies.
Potential confounders in immunoassays
Sandwich immunometric assays are potentially vulnerable to what has been termed the “hook” or “prozone” effect that can lead to falsely normal or low values in the presence of large amounts of the analyte8 (Figure 4-3). Generally, as analyte concentration increases, more antibody-analyte-antibody sandwiches are formed, leading to more signal detected. At extremely high analyte concentrations, however, it is possible for both capture and detection antibodies to be saturated with analyte prior to formation of a sandwich complex, leading to the detection antibody being washed away and the low signal being misinterpreted as a low concentration of analyte. This phenomenon is not seen with competitive immunoassays. To minimize the chances of a hook effect, most immunometric assays have now modified their methods as follows: analyte is allowed to bind to capture antibody, a thorough wash step removes excess unbound analyte, and only then is the detection antibody added. In the now extremely rare situation where a hook effect is still suspected, serial dilutions of the sample may be performed to see if the apparent concentration of the analyte actually rises with increasing sample dilution.
FIGURE 4-3 Hook (prozone) effect leading to falsely low results. Extremely high analyte concentration leads to occupancy of all binding sites without creating the assay “sandwich” configuration. Signal antibody is washed away and the low signal is interpreted as a low concentration of analyte (dotted line). Dilution of sample may lead to increasing signal and an apparent increase in the measured analyte concentration.
There is some inconsistency in the literature around the terms heterophilic antibody and human antianimal antibody (HAAA) or human antimouse antibody (HAMA). Some authors use these terms interchangeably; others reserve the term heterophilic for low-affinity antibodies that spontaneously arise against multiple, poorly defined antigens and use HAMA or HAAA only for those patients who develop specific high-affinity antibodies due to treatment with a murine monoclonal antibody or due to recurrent exposure to a particular animal species (e.g., scratches endured by a rabbit handler). Regardless of the term used, any given patient may have antibodies present that bind to and interfere with animal (mouse monoclonal; rabbit, goat, or donkey polyclonal) antibodies used in a particular immunoassay,9 even without previous exposure to that particular animal. These antibodies can bridge the capture and detection antibodies in an immunometric assay, as shown in Figure 4-4 (left panel), creating a sandwich and a high signal (false elevation) even in the absence of analyte. Alternatively, they could attach at the binding site of the capture antibody and prevent binding of the analyte (Figure 4-4, right panel), eliminating formation of the sandwich, and causing a falsely low value. Competitive immunoassays are less often affected, although they may show falsely high values if the interfering antibody outcompetes the assay antibody for tracer, or falsely low values if they bind preferentially to the analyte over the tracer.
FIGURE 4-4 Mechanism of immunometric assay interference by heterophilic or human antianimal antibodies. See text for details.
Specific autoantibody interferences appear to be rare compared to the heterophilic/HAAA/HAMA interferences described in the previous section, with the notable exception of TgAb in patients with thyroid cancer. TgAb is present in about 20% to 25% of thyroid cancer patients and leads to false depression of thyroglobulin levels by immunometric assay (e.g., Tg-ICMA). In addition, different thyroglobulin antibody assays may report discordant (negative versus positive) results in some cases. Although a competitive assay such as Tg-RIA is less often affected by TgAb interference than is the Tg-ICMA, if the autoantibody is of sufficiently high affinity to outcompete the assay antibody, a Tg-RIA may show false elevation in the presence of such TgAb.10 Overall, any Tg immunoassay result, whether performed by Tg-ICMA or Tg-RIA, must be viewed as potentially affected in a patient with TgAb pressent (and even possibly in patients whose TgAb is reportedly negative). Helpful proxies for Tg include following TgAb titer, which should fall over time in patients without cancer recurrence.11 Imaging studies may also help assess the probability of recurrence. Although rare, autoantibodies may be of sufficiently high affinity to affect both competitive and immunometric assays alike. Consequently, they would be less likely to show differences when assayed on different platforms and are not affected by heterophilic antibody blocking agents. In these scenarios, diagnosis may require measurement of specific autoantibody (e.g., a parathyroid hormone [PTH] antibody assay in someone with a discordantly high or low PTH level).
Limitations of immunoassays
The ability of an antibody to bind a specific target with high affinity is remarkable, yet there are limitations. The small size and relatively poor immunogenicity of steroid molecules make it difficult to obtain an antibody that can clearly distinguish a specific steroid from other similar steroids. For example, an antibody raised against testosterone may show significant cross-reactivity with a conjugated form such as testosterone glucuronide, or with the structurally similar dihydrotestosterone molecule. A solvent extraction step to remove water-soluble conjugates such as glucuronides, coupled with a chromatographic step to separate out structurally similar molecules, will greatly improve the analytic specificity of the assay12 (Figure 4-5A). The extraction step also separates testosterone from binding proteins that can interfere with accurate measurement.
FIGURE 4-5 A, Increasing specificity of analysis via extraction and chromatography. After organic solvent extraction, water-soluble conjugates remain in the aqueous phase while steroid molecules generally remain in the organic phase. Similar steroid molecules can be separated by an additional chromatography step. B, Correlation of testosterone assays (extraction/chromatography/RIA versus nonextraction immunoassay or tandem mass spectrometry). Left panel: Steroid immunoassays without additional preparation steps prior to assay tend to give higher values than immunoassays after extraction and chromatography, likely because of steroid conjugates and compounds of similar structure (occasional lower values may reflect issues with incompletely removed steroid binding proteins). Right panel: Measurement of steroids by HPLC-mass spectrometry (which also involves extraction and chromatography) produces testosterone results very similar to those obtained with immunoassay after extraction and chromatography.
Testosterone immunoassays that do not involve such extraction and chromatography steps, including the majority of assays found on most automated laboratory platforms, may perform adequately at the higher levels found in adult males (> 300 ng/dL) but will frequently measure inappropriately high values in the range most pertinent to women and prepubertal children (Figure 4-5B). Because in practice the lower values are more concordant with the clinical picture, endocrinologists caring for women and children have traditionally relied on immunoassays that involve extraction and chromatography steps, or assays involving chromatography and tandem mass spectrometry.
Free and bioavailable hormone tests
The free hormone hypothesis states that free, or unbound, steroids or thyronines, vitamins, or peptides are available to enter cells or interact with cellular proteins by virtue of their small size and sometimes their hydrophobic nature.13 Free hormone in this section means not liganded to a binding protein. As free and bound hormone pass through a tissue bed, the free hormone may be taken up by the tissues, additional free hormone may become available from the bound fraction, or the complexed hormone may be taken up by cells.14 The systems may be further complicated by multiple binding proteins. Clearly, the activities of several steroids, thyronines, and even protein hormones are reduced by binding to specific high–affinity binding proteins and albumin. Conversely, the absence of binding proteins can reduce the total hormone level, but the free hormone level may be unaffected. For example, the majority of thyroid hormone in circulation is carried by thyroxine-binding globulin (TBG). TBG deficiency is characterized by low total thyroid hormone levels, normal free thyroid hormone levels, normal TSH (in equilibrium with the free T4), and clinical euthyroidism.
Testosterone binds to sex hormone binding globulin (SHBG) and serum albumin. Testosterone binds SHBG with much higher relative affinity, but SHBG is present in much lower concentrations than albumin. Free testosterone may be measured by equilibrium dialysis, which is accepted as the best indication of the physiologic availability of testosterone (Figure 4-6A). The free fraction may also be estimated mathematically from the testosterone, SHBG, and albumin concentration; however, not all assumptions may be valid in calculated free testosterone.15, 16 Analog methods measure free testosterone directly using a competitive test in automated assay instruments (Figure 4-6B), but the analog methods may at best weakly reflect free hormone activity. Similarly, free T4 measured by analog methods can perform poorly in some settings.
FIGURE 4-6 A, Free steroids may enter cells by passive diffusion through plasma membranes. Bound steroids may not diffuse through membranes. Once in the cell, steroids may interact with enzymes, or nuclear receptors. B, Free thyroxine (FT4) measurements, routinely made by analog immunoassay largely reflect total thyroxine. (Fritz KS, Wilcox RB, Nelson JC [2007]. A direct free thyroxine [T4] immunoassay with the characteristics of a total T4 immunoassay. Clin Chem 53:911-915.) Dialysis with HPLC and tandem mass spectrometry or dialysis and immunoassay methods provide theoretic accuracy. Dialysis methods should be used where binding proteins vary from the norm. T4 interacts with the antibody used in the FT4 test and competes with the analog; however, the analog does not bind thyroxine-binding globulin (TBG).
Bioanalytic testosterone refers to the fractions of testosterone bound to serum albumin plus the free hormone; for this reason this fraction is also called free and weakly bound testosterone. The dissociation rate of albumin bound is very fast relative to that of the SHBG bound, and for that reason it is thought to be available to the tissues for actions. This idea, supported by empirical evidence, is in contrast to the free hormone hypothesis, as free and protein-associated hormone may be available to the tissues.14 In practicality, measurement of free or bioavailable hormones provides the same information to the clinical encounter.
Mass spectrometry
Mass spectrometry is a qualitative and quantitative analytic technique that separates molecular ions based on their mass and charge, or more exactly according to the mass/charge ratio (Figure 4-7). In clinical laboratories, mass spectrometry is invariably preceded by chromatographic assays that separate hormones. Separation techniques include high-pressure liquid chromatography (HPLC), column chromatography, gas chromatography, thin-layer chromatography, electrophoresis, and other variations limited only by the imaginations of the inventors. In all chromatography assays, the hormone is attracted to both a mobile phase and a stationary phase, and separation occurs by variations in attraction of hormone to the two phases. Table 4-2 highlights some of the chromatographic techniques used in endocrinology.
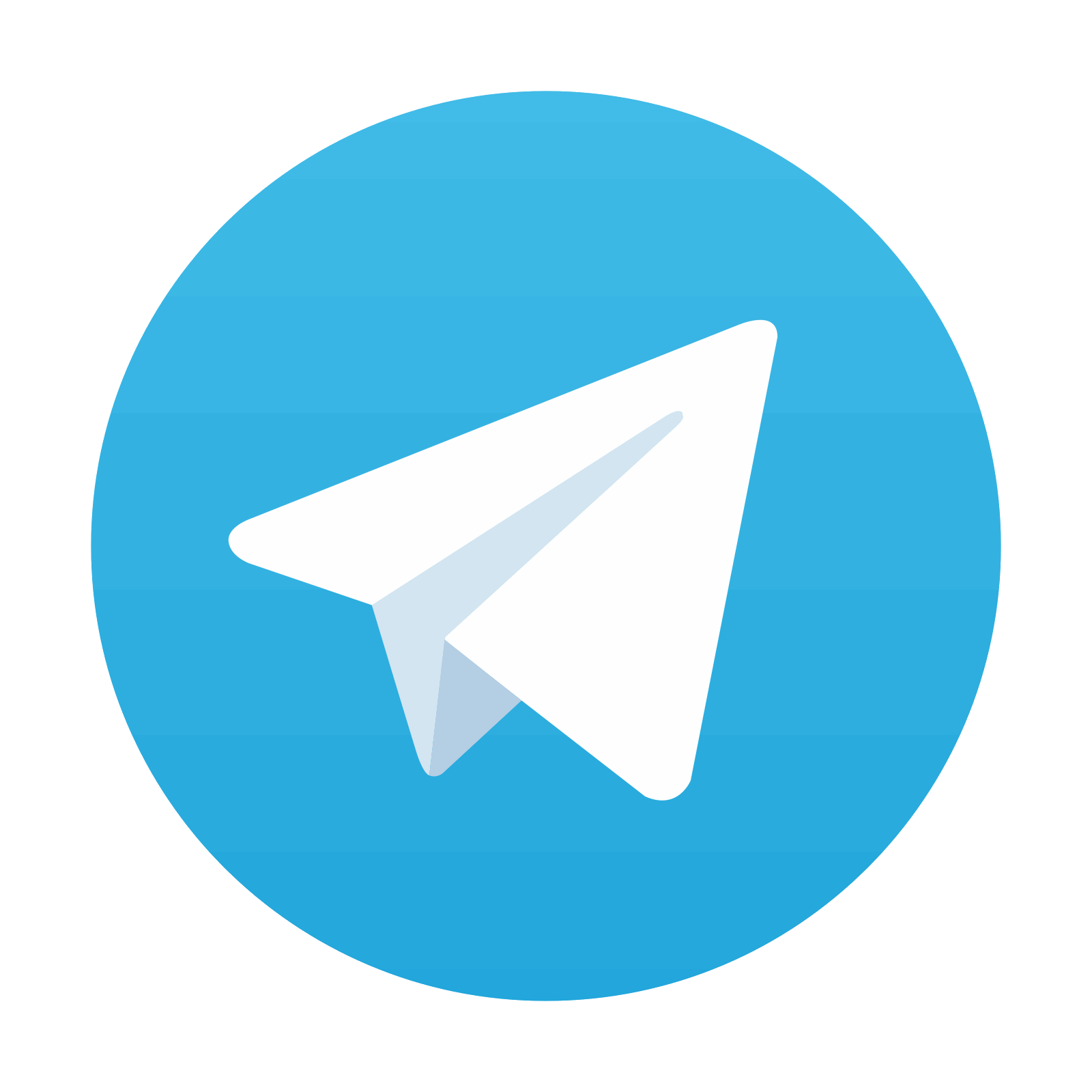
Stay updated, free articles. Join our Telegram channel

Full access? Get Clinical Tree
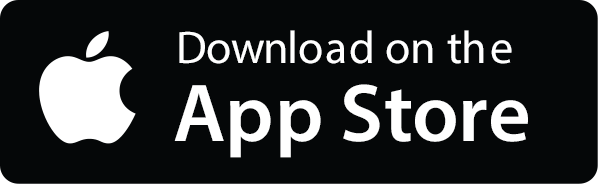
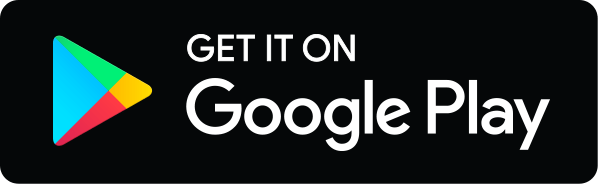