- Insulin is a potent anabolic hormone essential for life. Insulin has a circulating concentration of 15–20 mU/L in the fasting state, and 60–80 mU/L post-prandially.
- Early insulins were extracted from the pancreata of pigs and cows but good glycemic control was difficult to achieve because of residual impurities after the purification process. The newer and purer animal insulins are better tolerated and can potentially achieve a level of glycemic control similar to synthetic human insulins. Clinically significant hypoglycemia rates between the human and animal insulins also appear to be similar.
- The challenge of insulin replacement therapy is to reproduce a normal physiologic insulin profile without incurring significant hypoglycemia. A range of insulin preparations with differing durations of actions are available to achieve this: rapid-acting insulin analogs (about 3 hours), soluble insulin (about 6–8 hours), neutral protamine Hagedorn (NPH) insulin (12–18 hours), lente insulins (about 12–24 hours), long-acting insulin analogs (about 24 hours).
- Current recommendations for the injection of insulin are that the skin is pinched to reduce the risk of injecting into muscle, and to inject at a right angle to the skin after which the fold should be held for 5–10 seconds before removing the needle. Injection needle lengths of 5–12 mm are available to ensure that the insulin is delivered deep enough into the subcutaneous tissue to prevent back-leakage, but not so deep that it is intramuscular. Alternative modes of insulin administration such as continuous subcutaneous insulin infusion can also be highly effective.
- Multiple-dose insulin therapy is an appropriate initial approach to reproducing the physiologic insulin profile in patients with absolute insulin deficiency such as those with type 1 diabetes. This consists of a long-acting insulin preparation administered once or twice a day to meet the basal insulin requirement, with the injection of a short-acting insulin preparation with each meal.
- A number of different insulin injection regimens are available for patients with type 2 diabetes who may already be treated with non-insulin-based therapies. These include a once daily injection of a long-acting insulin, a once daily injection of a long-acting insulin with an injection of a short-acting insulin with the main meal, twice a day injections of insulin mixtures, and multiple dose injections.
Life (and death) before insulin
Insulin is a potent anabolic hormone, and its absence induces a profound catabolic state that affects fat, carbohydrate and protein stores. Absolute insulin deficiency, such as that characterized by type 1 diabetes (T1DM), will result in death if left untreated (Figure 27.1). What the study of patients with diabetes in the pre-insulin era teaches us is the surprisingly long period that can be survived without insulin. Leonard Thompson was the first patient to have effective insulin treatment. He was 12 years old when he was diagnosed with diabetes in 1919 and had survived over 2 years when at 11AM on January 22, 1922 he received his first injection of insulin. This said, it was a miserable existence without insulin. Other than the weight loss, constant tiredness, thirst, urination and frequent infections, there was the certain knowledge that a death sentence had been passed, and that death would be an agonizing and slow process. The most effective therapy available at that time appeared to be severe nutritional restriction, perhaps most popularly expounded by Allen [1] from the Rockefeller Institute in New York. However, this was a difficult regimen which did not appear to prolong life expectancy significantly, and when death came it was not clear whether it was the result of diabetes or starvation.
The discovery of insulin
Although there had been previous attempts to identify a pancreatic agent that could control blood sugar, most notably by the Romanian physiologist Nicolas Paulesco, the story of insulin may be considered to start in the autumn of 1920 when Frederick Grant Banting who, while preparing a lecture on carbohydrate metabolism, considered a way to isolate the internal secretion of the pancreas. By the summer of the next year he had arranged with John Macleod, Professor of Physiology at the University of Toronto, for facilities and the support of a science student Charles Best to start experimentation (Figure 27.2). They showed that tying off the pancreatic ducts induced atrophy of the exocrine glands of the pancreas, thus allowing the “internal” secretions to be isolated. They tested the effect of this internal secretion on blood sugar by injecting it into dogs rendered diabetic through pancreatectomy. With the help of a biochemist, James Collip, they were able to purify this abstract sufficiently for the early experiments on patients. For this work, Banting and Macleod shared the Nobel Prize for Medicine in 1923.
Figure 27.2 Charles Best and Frederick Banting on the roof of the medical building at the University of Toronto, summer 1921.
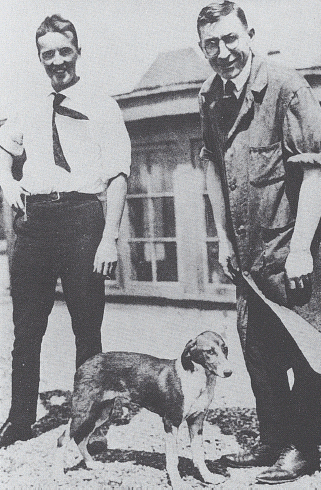
By no means was this discovery a straightforward process, and according to one particularly harsh commentator, “the production of insulin … originated in wrongly conceived, wrongly conducted and wrongly interpreted series of experiments” [2]. The discovery of insulin nevertheless was a defining moment in the history of diabetes and the molecule has gone on to be well studied, with work contributing to three further Nobel Prizes: Frederick Sanger in 1958 for determining its primary amino acid sequence; Dorothy Hodgkin in 1964 for X-crystallographic studies; and Rosalyn Yalow et al. in 1977 for contributing to the development of radioimmunoassays.
The first insulins
Commercially available insulin was initially extracted from porcine and bovine pancreata. The organs would be freshly collected from abattoirs and frozen immediately to prevent the enzymatic degradation of the insulin protein. The tissue would undergo acid-ethanol treatment to solubilize insulin, which would then be salted out with sodium chloride, precipitated and then crystallized [3]. This procedure resulted in insulin with a purity of 80–90%, the contaminants largely being pancreatic polypeptides and glucagon. Although this insulin was effective, it was often complicated by immune-mediated side effects, in particular lipoatrophy and antibody-mediated insulin resistance [4], both of which that can profoundly influence the kinetics of insulin action. These impurities also contributed to an allergic reaction which sometimes resulted in swelling and pain at the site of injection.
Animal and human insulins
Animal insulin continues to be porcine and bovine derived. The amino acid structure of bovine insulin differs from that of human by three amino acids (position 30 of the B chain, and 8 and 10 of the A chain) and that of porcine from human by just one amino acid (position 30 of the B chain) [5,6]. These differences aside, it was the purity of the insulin preparation that appeared to influence its immunogenicity.
In the 1970s, additional purification steps using gel filtration and ion exchange chromatography resulted in an insulin, called mono-component or single component insulin, with fewer side effects and which was better tolerated (Table 27.1). The incidence of lipoatrophy and allergy decreased as these purer insulins became more widely available. It was a logical step therefore to move on to treatment with human insulin in an effort to further reduce immune-mediated complications and improve glucose control.
Table 27.1 Insulin therapy milestones.
1922 | Isolation of insulin and treatment of the first patient |
1936 | Protamine insulin |
1946 | NPH isophane insulin |
1951 | Zinc lente insulin |
1959 | Biphasic insulin |
1977 | Continuous subcutaneous insulin infusion |
1980 | rDNA human insulin in humans |
1981 | Insulin pens |
1987 | Monomeric short-acting insulins |
1987 | Soluble prolonged-action insulin |
1996 | Rapid-acting insulin analog |
2001 | Long-acting insulin analogs |
Human insulin has been obtained using three techniques. The earliest attempts were to isolate and purify it directly from human cadaveric pancreata. The supply of human tissue, however, was never sufficient to enable this technique to provide sufficient quantities. The technique of “semi-synthesis” chemically converts porcine insulin to the human sequence through the substitution of the one amino acid difference in the primary sequence. It was only in 1980 with the introduction of recombinant DNA technology that human insulin therapy became widely available. The technique involves the insertion of the human DNA sequence into a host cell, usually bakers’ yeast or the bacteria Escherichia coli, allowing it to synthesize the insulin molecule [7]. The protein would then be purified usually via chromatography columns to achieve a 99.5–99.9% insulin purity. The quality of the human insulin preparation achieved with this technique, as indeed with the purified porcine insulin, has virtually eliminated problems associated with immune-mediated side effects.
Counter to expectations, rigorous and well-designed studies comparing purified animal insulin with recombinant human insulin have yet to demonstrate a significant benefit in glycemic control. A recent meta-analysis of 45 randomized controlled trials involving a total of 2156 participants comparing animal and human insulin failed to show a significant difference between these two therapies [8]. Most (36 of the 45) studies used highly purified porcine insulin, which many believe to be less immunogenic than bovine insulin and this may explain the favorable results for animal insulins. Overall, there is a marked absence of good studies demonstrating a superiority of human over animal insulin with regard to glycemic control. Bovine insulin was associated with a higher titer of anti-insulin antibodies, but these titers did not appear to influence the dose of insulin required, or the level of glycemic control achieved.
The move from animal to human insulin was associated in some patients with reports of greater hypoglycemia, anecdotally brought about by changes in hypoglycemia warnings [9,10], but systematic reviews have failed to substantiate this finding in population studies [8,11].
There has been a large-scale uptake of recombinant human insulin such that less than 7% of global insulin sales are now animal insulin. This shift has probably resulted at least in part, because of the phasing out of animal insulins by the leading insulin manufacturers. Several companies continue to provide this alternate source, and both bovine and porcine-derived insulin remain available. Although cheaper to produce, the price of animal insulins in the UK is structured at a cost comparable to that of recombinant human insulin (£17–18 per 10 mL vial; BNF 2009). This pricing strategy is not the same worldwide; in the developing world for example, the cost of animal insulin is about half that of recombinant human insulin, a factor that may influence the choice of insulin preparation [12].
Soluble and long-acting insulin preparations
The biologic action of soluble insulin lasts about 5–6 hours and the early preparations were often also associated with pain and swelling at the site of injection. Attempts were therefore made in the 1920s and 1930s to provide the patient’s daily insulin requirement in just one injection. To this end, modifying agents such as lecithin, oil and cholesterol were used [13]; however, their duration of action varied significantly from injection to injection which made their clinical use very difficult.
In 1936 a method for incorporating insulin into a poorly soluble complex, thus slowing its absorption, was reported [14]. This technique involved the addition of a highly basic protein, protamine, derived from the sperm of salmon or trout. The complex was further stabilized by the addition of a small amount of zinc such that it lasted about 24 hours, and this insulin was called protamine-zinc insulin. It was difficult to make consistent batches and absorption again tended to be erratic. In 1946, the technique was further refined such that protamine and zinc were added in stoichiometric proportions (so that there was no free protamine or zinc), which resulted in a preparation that was absorbed at a more consistent rate and lasted 12–24 hours. This insulin was called isophane or neutral protamine Hagedorn (NPH), and became available clinically in 1940.
In 1951, a development that prolonged the action of insulin without the need for protamine was reported; this required zinc to be added in excess and in acetate buffer, resulting in crystals of relatively insoluble zinc-insulin complexes, called the lente insulins [15]. The size of the crystals could be adjusted by changing the pH such that larger crystals, which were more slowly absorbed (ultralente), and smaller crystals (semilente) could be produced. A preparation containing a 70:30 ratio of the ultralente and semilente insulins (lente insulin) was the most popular form of the zinc insulins and was used widely in clinical practice.
Rapid and long-acting insulin analogs
Insulin circulates as single molecules in the blood at concentrations of approximately 10–9 mol/L. At higher concentrations, such as in commercial preparations, insulin molecules tend to associate non-covalently into dimers, tetramers and hexamers [16]. The presence of zinc further stabilizes the hexamer association. Following injection, fluid is drawn into the injected insulin depot through osmosis. This leads to dilution of the insulin and dissociation of the insulin molecules, a spontaneous but gradual process that must occur before insulin can cross the capillary walls as monomers into the blood circulation [17,18]. Patients are therefore advised to inject their soluble insulin 15–20 minutes before a meal so that circulating insulin levels are optimal at the time their meal is being absorbed (Figure 27.3). A significant proportion of patients find it hard to follow this advice because of the planning required. Even when they do, the calculated doses may be inaccurate, particularly when the preparation of the meal is not under the patient’s control.
The association between insulin molecules can be reduced by specific changes to the amino acid sequence of insulin [19]. These changes result in faster absorption of the insulin into the bloodstream, and allow it to be injected just before, or even immediately after eating a meal [20]. To date, three such rapid-acting analogs (RAIs) have become available: insulin lispro (Humalog®, Lilly), insulin aspart (Novorapid®, Novo Nordisk) and insulin glulisine (Apidra®, Sanofi-Aventis). These analogs act more quickly (within 10–20 minutes) and have a shorter duration of action (3–5 hours) than soluble insulin (30–60 minutes, and 6–8 hours, respectively) (Figure 27.3) [21]. A number of studies have now demonstrated the safety of RAIs in both T1DM and type 2 diabetes (T2DM), as part of a “basal bolus” insulin injection regimen combined with intermediate-acting insulins, and in continuous subcutaneous insulin infusion (CSII) [22]. The time–action profile of RAIs is well suited to mimicking the requirement at meal times, and therefore they probably control post-prandial hyperglycemia more effectively than soluble insulin. As a result, patients can achieve better glycemic control with fewer episodes of hypoglycemia with RAIs than with soluble insulin. The benefits of RAI over soluble insulin appears to be clearer in studies involving patients with T1DM than T2DM, and using CSII than for multiple dose injections [21].
Although delayed-action insulin such as insulin lente and isophane can cover the 12–24-hour period, their absorption from subcutaneous tissue can vary significantly [23]. Therefore, attempts at stringent glucose control can be associated with an increased risk of hypoglycemia. The modification of the primary amino acid sequence of the insulin molecule can result in a shift in the isoelectric point towards neutrality (the isoelectric point is that at which the molecule is least soluble). This shift in the isoelectric point therefore encourages insulin to precipitate following injection into the subcutaneous tissue (which is at neutral pH), and results in a slower and, more importantly, a more reproducible absorption [24]. Early studies of the long-acting analog insulins, designed to demonstrate equivalence rather than superiority over the isophane NPH insulins, have failed to show a clear benefit in glycemic control [25]. However, what the studies do appear to show is that achieving glycemic control with the long-acting insulin analogs is associated with less symptomatic and nocturnal hypoglycemia [26].
Reproducing physiologic insulin delivery – the size of the problem
In health, the background insulin secretion is low and stable between meals, with significant prandial surges (Figure 27.4). The stimulus and pattern of insulin secretion is reviewed in Chapter 6, but suffice it to say that the background as well as the prandial insulin secretion can vary significantly within the same individual; the former according to the level of counter-regulatory hormones such as growth hormone and catecholamines, and the latter according to the carbohydrate and protein content of the meal [27].
Figure 27.4 Pancreatic insulin secretion in a healthy non-obese individual. Meals were consumed at 0900, 1300 and 1800. Statistically significant pulses of secretion are shown by the arrows. Reproduced from Polonsky et al. [27], with permission from the American Society for Clinical Investigation.
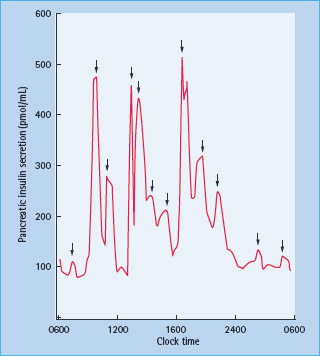
Furthermore, the insulin is secreted into the portal circulation, with about 50% of the insulin removed in the first pass through the liver [28]. The continuous low levels (15–20 mU/L) of insulin secretion act to suppress hepatic glucose output. Peripheral tissues such as muscle also take up glucose but this is at higher insulin concentrations (60–80 mU/L) and tends to occur predominantly post-prandially.
The challenge of insulin replacement therapy is to reproduce the physiologic insulin profile without incurring significant risk of hypoglycemia.
Routes of insulin administration other than subcutaneous
The early experiments of Frederick Banting quickly revealed that the oral route of insulin administration was not an effective means of delivery, and subsequently that the insulin molecule was functionally degraded by gut peptides. Subcutaneous injection of insulin has become the most popular route of insulin delivery because of its relatively reproducible kinetics of absorption, and the relative ease with which it can be administered, but it is worth visiting some of the other routes.
Intramuscular injection is more painful and absorption more rapid than the subcutaneous route [29] but is useful, for example, in the emergency situation when intravenous access is difficult. Inadvertent intramuscular administration should be considered in the slim patient with diabetes who complains of pain on insulin injection, and who may have erratic glucose control and hypoglycemia. The correct choice of insulin needles can help address this problem.
Intraperitoneal insulin administration is absorbed more quickly than the subcutaneous route and has gained some interest recently for use with implantable insulin pump therapy [30]. An advantage is that the major part of absorbed insulin is into the portal circulation [31], thus reducing the risks of high levels of peripheral insulin that may conceivably contribute to the vascular complications of diabetes and development of obesity [32,33]. Certainly, the early studies of implantable intraperitoneal pumps appear to achieve better glucose control and less hypoglycemia than the subcutaneous route. A longer experience of intraperitoneal insulin delivery has been obtained in patients requiring renal replacement therapy using continuous ambulatory peritoneal dialysis where the insulin is administered along with the dialysis fluid. There are some reports that this route is associated with more consistent absorption and lower peripheral insulin levels; however, there are no robust trials to show that any one route is superior to another [34,35].
The oral administration of insulin is a route by which this drug can enter the portal circulation, thus avoiding high levels of peripheral insulin. There are emerging reports that such an approach may be possible. Techniques such as insulin modification to engender resistance to degradation [36,37] have been reported to increase circulating insulin concentrations effectively and decrease blood glucose in early phase clinical trials.
Subcutaneous route of insulin administration
Subcutaneous insulin administration is the most popular route of insulin therapy and suggested sites for injection are the abdomen, thigh and deltoid. Despite a scarcity of clinical trials on this subject, the current recommendations are that the skin is pinched to reduce the risk of injecting into muscle, and to inject at right angles to the skin, after which the fold should be held for 5–10 seconds before removing the needle (Figure 27.5) [38].
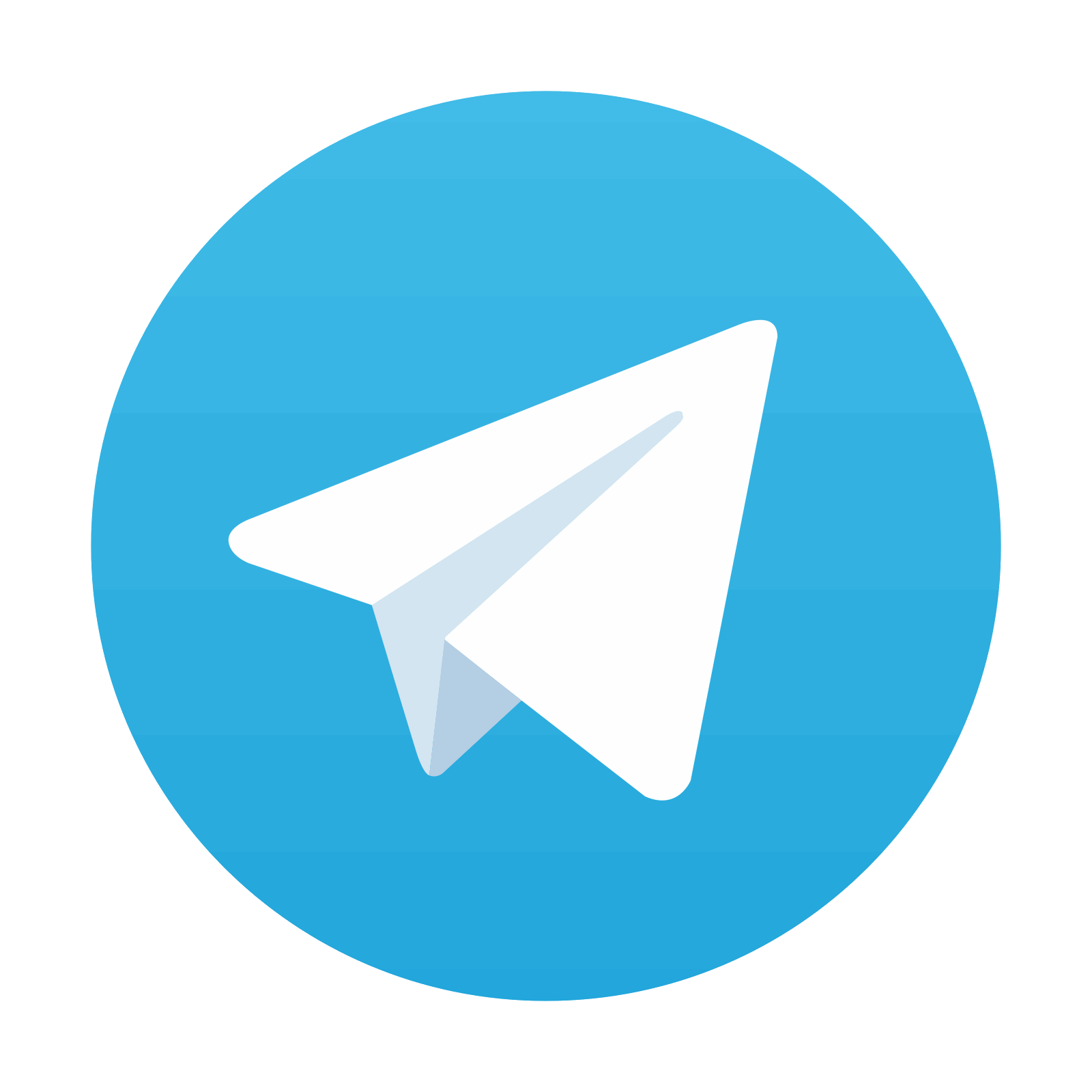
Stay updated, free articles. Join our Telegram channel

Full access? Get Clinical Tree
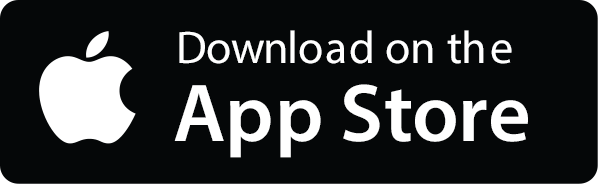
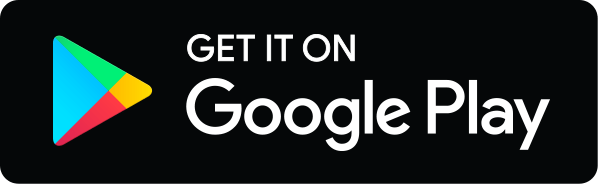