Inflammatory Breast Cancer
Beth Overmoyer
Lori J. Pierce
DEFINITION AND DIAGNOSTIC CRITERIA
Historical Perspective
The term “inflammatory breast cancer” (IBC) was first introduced by Lee and Tannenbaum in their sentinel 1924 manuscript, to describe a rare, rapidly progressing breast cancer with characteristic features of inflammation seen among 28 cases at Memorial Hospital (1). They discussed the grave prognosis of breast cancer when associated with inflammatory changes, as first described by Sir Charles Bell in 1812 (1a); “When a purple color is on the skin over the tumor accompanied by shooting pains, it is a very unpropitious beginning.” Throughout time, this form of breast cancer was referred to as “mastitis carcinomatosa” (1b), “carcinoma mastitoides” (1c, 1d), and “acute carcinoma of the breast” (1e, 1f).
The historical identification of IBC is important as a reminder of the evaluation and acceptance of classic clinical criteria that differentiates it from other forms of locally advanced breast cancer (LABC). More recently, there has been increased awareness concerning the distinctive clinical and pathologic nature of IBC, which has resulted in earlier identification of the disease, enhanced understanding of its unique biologic elements, and utilization of these features to develop improved therapies resulting in a superior disease response. It is anticipated that a greater understanding of IBC will also lead to advances in the treatment of non-IBC, because successful treatment of a virulent form of disease in general, often results in therapeutic progress for less aggressive disease.
Clinical-Pathologic Characteristics
Inflammatory breast cancer is a rare subtype of breast cancer, accounting for 1%-5% of breast cancer in the United States (2, 3). Between 1988 and 1990 and 1997 and 1999, the incidence of IBC increased from 2.0/100,000 women-years, to 2.5/100,000 women-years (p < .001), which translates into a rate of 1.23% per year (4). IBC can also rarely present in male patients, and all pathologic subtypes of invasive adenocarcinoma of the breast can be associated with IBC, though invasive ductal carcinoma is the most common type (5).
“Primary” IBC presents as LABC in a previously unaffected breast, characterized by a rapid onset of symptoms within approximately 3 months. This rapid onset of symptoms and signs distinguishes primary IBC from LABC with inflammatory changes. The clinical features of IBC consist of enlargement of the breast with associated tenderness and warmth, often without a palpable mass (Fig. 59-1). Extensive nodal involvement is often clinically assessable, involving fixed ipsilateral axillary, supraclavicular, and internal mammary nodal groups, as well as frequent extension to contralateral axillary lymph nodes (6, 7). Erythema of the skin,
involving approximately one-third or more of the breast can vary in character from faint pink, to red or purple; either diffuse or developing in a serpiginous fashion (8). The extent of erythema may eventually extend into the contralateral breast via dermal lymphatic extension. Edema or induration of the skin, with pitting of the hair follicles, is often described as peau d’orange (orange peel-like in consistency) (6, 9). Ulceration of the skin and/or nipple is quite rare. This classic appearance of the breast is not due to inflammatory infiltrates, but rather to lymphatic occlusion from tumor emboli present in the papillary and reticular dermis of the skin. Pathologic confirmation of dermal lymphatic involvement is not required for the diagnosis of IBC, and because of skip regions within the breast, dermal lymphatic invasion can be confirmed in only 75% of IBC skin biopsies. The irregularity of the dermal lymphatic involvement contributes to the characteristic feel of “ridges” within the breast.
involving approximately one-third or more of the breast can vary in character from faint pink, to red or purple; either diffuse or developing in a serpiginous fashion (8). The extent of erythema may eventually extend into the contralateral breast via dermal lymphatic extension. Edema or induration of the skin, with pitting of the hair follicles, is often described as peau d’orange (orange peel-like in consistency) (6, 9). Ulceration of the skin and/or nipple is quite rare. This classic appearance of the breast is not due to inflammatory infiltrates, but rather to lymphatic occlusion from tumor emboli present in the papillary and reticular dermis of the skin. Pathologic confirmation of dermal lymphatic involvement is not required for the diagnosis of IBC, and because of skip regions within the breast, dermal lymphatic invasion can be confirmed in only 75% of IBC skin biopsies. The irregularity of the dermal lymphatic involvement contributes to the characteristic feel of “ridges” within the breast.
![]() FIGURE 59-1 Classic clinical features of inflammatory breast cancer. (A) Erythema, edema (peau d’orange). (B) Enlargement of the breast. |
Primary IBC is differentiated from “secondary” IBC which is clinically and biologically similar; however, secondary IBC arises as a breast cancer recurrence in the breast or chest wall of a patient with a previous history of treated non-IBC. In the past, there have been inconsistencies in the definition of IBC and this has complicated the analysis of epidemiologic studies and clinical trials. However, recently there has been a consensus agreement on defining the clinical characteristics of IBC which has permitted the differentiation from non-IBC LABC, and has improved the interpretation of clinical outcomes of IBC (10). Acceptable diagnostic criteria for IBC are based upon both clinical and pathologic features and include the International Classification of Disease for Oncology (ICD-0-2, 8530/3 for IBC) and the American Joint Committee on Cancer (AJCC) staging criteria (3, 11). According to the AJCC staging criteria, the tumor in IBC is classified as T4d, and staged as IIIB, IIIC, or IV based upon extent of nodal involvement and evidence for distant metastasis.
Epidemiology
Based upon data from the Surveillance, Epidemiology, and End Results (SEER) Program, patients with IBC usually present at an earlier age compared with non-IBC LABC; approximate mean age at diagnosis 58 years and 66 years, respectively (3). The incidence of IBC increases to the age of 50 years, then plateaus, regardless of hormone receptor (HR) status, whereas the incidence of non-IBC continues to increase after age 50 years, especially among estrogen receptor (ER) positive disease (2, 4). The incidence of IBC is higher among African American women compared with Caucasian women (3.91/100,000 women-years compared with 2.5/100,000 women-years, p <.001), and has geographic variation within the United States and globally (12). For example, SEER data from 1992-2002 demonstrated the frequency of IBC documented in the Los Angeles Tumor Registry as 2.45% whereas the frequency of IBC in the Connecticut Tumor Registry during the same period was 1.49% (4). Internationally, there is an increased frequency in the Middle East and Northern Africa, e.g., 11% occurrence in Egypt, 5%-7% in Tunisia (decreased from 50% using stringent diagnostic criteria), 10% in Pakistan, and 17.5% in Nigeria; versus 2.9% in Spain, 5.4% in France, and 0.6%-2% incidence in Italy (4, 7, 13, 14 and 15). Other epidemiologic features that have been associated with a higher incidence of IBC include living in a high poverty county within the United States, having a high BMI (regardless of menopausal status, though there is a propensity of developing IBC while premenopausal), an earlier age of menarche, and earlier age of first full-term pregnancy (16, 17 and 18). A familial or genetic linkage for IBC has not been borne out.
Approximately 30% of patients with IBC present with distant metastasis at the time of initial diagnosis (stage IV), based upon SEER data examined from 2004-2007 (19). Among individual institutions, the incidence ranges from 26% (City Hospital, Nottingham, UK) to 40% (MD Anderson Cancer Center); however, all data supports a significantly higher occurrence of stage IV disease at presentation with IBC than with non-IBC (20, 21). Compared with non-IBC LABC, the 2-year breast cancer specific survival (BCSS) for IBC was 84% vs. 91% (p < .0001) (22). This translated into a hazard ratio of 1.43 risk of dying of breast cancer among patients with IBC vs. non-IBC LABC (p = .008). Among the SEER cases from 1990 to 2008, the median BCSS for non-IBC LABC was 13.4 years versus 4.75 years among patients with IBC (23).
These data support the importance of accurate diagnosis for IBC which carries a significantly poorer prognosis compared with non-IBC LABC.
Imaging
The imaging employed during the evaluation of IBC serves to identify the extent of disease within the breast and regional lymph nodes, determine spread to the ipsilateral pectoralis muscle or contralateral breast, to detect distant metastasis, and assess disease response to treatment. As in non-IBC, mammography is utilized as an initial diagnostic procedure, wherein the affected breast demonstrates skin thickening (from dermal lymphatic involvement), diffuse increased breast density, and trabecular distortion due to edema. Although most patients with IBC do not present with a palpable breast mass, in a large retrospective study of 80 patients with IBC, 80% of the patients displayed mammographic abnormalities in the affected breast, such as architectural distortion, asymmetry, multi-focal densities (masses), or suspicious calcifications (41%) (24). Microcalcifications often become more evident with effective treatment that results in a decrease in the density of the breast parenchyma (25). Occasionally, the mammographic findings are subtle and can only be detected by direct comparison with the contralateral breast. IBC appears to be a predominately unilateral breast disease, with previous reports of bilateral cancers now being attributed to non-IBC LABC.
Mammographic differentiation between IBC and nonmalignant inflammation of the breast can be complicated by the patient’s inability to undergo adequate compression of the breast due to pain. For this reason, ultrasonography is often utilized to assess skin thickening and regional lymph node involvement as well as identify areas within the breast that can successfully undergo biopsy via ultrasound guidance. In contrast to mammography, these abnormalities can be identified in 95% of patients with IBC (24). Ultrasound is more sensitive at detecting abnormal anatomic changes within the axillary lymph nodes, and color Doppler sonography can be used to assess intratumoral vascularity, however, microcalcifications cannot be detected via ultrasound.
A review of 4 studies evaluating the efficacy of magnetic resonance imaging (MRI) in IBC described skin thickening and enhancement (98% and 94%, respectively), and more commonly than a discrete mass, the parenchyma contained an infiltrative mass associated with a reticular/dendritic pattern of enhancement in 78% of patients (25). The retrospective study by Yang et al. found MRI detection of multiple breast masses with heterogeneous internal enhancement associated with a washout in 97% of their 80 patients (24). Overall, MRI was found to be the most accurate imaging modality for IBC, not only useful in detecting the primary cancer (98% vs. 68% with mammography), but also in imaging axillary lymph nodes and determining cancer involvement of the pectoralis muscle (26). In addition, MRI imaging posttreatment has the highest correlation with pathologic disease response compared with other modalities, such as mammography and ultrasonography (25).
The use of PET/CT (fused positron emission tomography and computed tomography) in breast cancer remains controversial; however, pretreatment imaging with PET/CT among patients with IBC has resulted in the detection of new areas of distant metastasis in 44% of 62 patients with IBC evaluated in one retrospective analysis (27). The extent of pretreatment nodal involvement detected by PET/CT imaging resulted in changes in the posttreatment radiation therapy fields in 18% and 14% of IBC patients in two independent retrospective analyses, though the clinical impact of these therapeutic modifications remains unknown. These data suggest that PET/CT may be considered as an imaging modality in the initial evaluation of IBC; however, confirmatory data is needed prior to recommending it as a standard approach (27, 28).
The current armamentarium of breast imaging is associated with some ambiguity in the evaluation of IBC, both in terms of diagnostic information as well as confirming disease response. Novel molecular targeting agents are currently being explored in IBC with the goal of demonstrating more sensitive and specific non-invasive evidence of disease response to systemic treatment (29).
BIOLOGIC CHARACTERISTICS
Breast cancer is a heterogeneous disease characterized by expression of specific molecular markers that control the biologic phenotype of the disease. IBC has been shown to express specific molecular characteristics that are more uniformly associated with this disease, and have thus permitted the characterization of IBC based upon biologic criteria rather than from purely histologic or clinical classification. A greater understanding of these distinctive mechanisms that coordinate the progression of IBC will allow the development of more successful targeting agents designed specifically for this disease.
Gene Expression Profiling
High-throughput molecular technologies, such as DNA microarray-based expression profiling, have revealed the full complement of breast cancer cell-of-origin subtypes (i.e., Luminal A, Luminal B, basal-like, HER2-overexpressing) within IBC; however, IBC has a propensity for segregating into the more proliferative molecular subtypes, such as the basal-like (triple negative, i.e., ER-negative, PR-negative and HER2-negative) and HER2-overexpressing subtypes. Bertucci et al. first demonstrated the same heterogeneity of cell-of-origin subtypes within 37 IBC tumor samples and 1 IBC cell-line (SUM-149) (30). Compared with 44 non-IBC LABC tumor samples, 24% of IBC samples were HER2-overexpressing and 22% were basallike, versus 14% HER2-overexpressing and 19% basal-like. These findings were confirmed in a subsequent analysis from Van Laere et al., wherein 16 pretreatment IBC tissue specimens exhibited all of the different cell-of-origin subtypes, yet 50% of the IBC samples segregated into the combined basal-like and HER2-overexpressing clusters compared with only 17% of the non-IBC samples (31). A principal component analysis of variability of gene expression in the entire sample set revealed that approximately 30% of the variation between IBC and non-IBC samples was related to the cell-of-origin subtype, suggesting the presence of other genetic factors that significantly contribute to the IBC phenotype.
Several investigators have attempted to discover a specific IBC gene signature; however, the results have been inconclusive. A RT-PCR analysis of mRNA expression among 36 pretreatment IBC tumor specimens identified 48 genes involved in cellular proliferation, signal transduction, apoptosis, and angiogenesis (32). Interestingly, the investigators found no difference in the expression of inflammatory cytokines, except IL-6, supporting the conclusion that the inflammatory characteristics of IBC were due to dermal lymphatic occlusion by tumor emboli and not by classical inflammatory mechanisms. They also identified a 3-gene expression signature that was associated with a poor prognosis in IBC. Van Laere et al. expanded their original work and identified an IBC signature that was associated with NF-κB activation, although the association of NF-κB target genes appeared to be linked to ER negative breast cancer as well as with IBC
(33, 34). Within their IBC gene signature, several genes were identified that were linked to insulin-like growth factor (IGF) signaling, which is supported by several molecular analyses described later in this chapter.
(33, 34). Within their IBC gene signature, several genes were identified that were linked to insulin-like growth factor (IGF) signaling, which is supported by several molecular analyses described later in this chapter.
The Baylor group identified a hyperproliferative gene profile involving fatty acid and lipid metabolism, the Bcl2-BAX apoptotic pathway, and genes associated with increased cell turnover (35). When their signature was compared with the Van Laere IBC signature, only 5 genes overlapped, and when the signature was compared with the Bertucci signature, only 1 gene was similarly over-expressed (30, 33). Other studies examining specific IBC gene signatures have identified clusters of oncogenes that encode proteins involved in protein translation and transport, adaptation to hypoxia, and activation of mammalian target of rapamycin (mTOR) signaling (36, 37). These heterogeneous gene profiles examined the tumor epithelium, whereas investigators from the National Cancer Institute (NCI) explored gene expression signatures among the tumor stroma of 52 IBC tissue specimens and found an enrichment of genes involved in intracellular protein transport and localization, protein secretion, mRNA translation, and GTPase signaling (38). The investigators proposed that the IBC phenotype was more easily distinguished by its stromal signature than the tumor epithelial signature. All of these studies demonstrate a significant heterogeneity within IBC, and although a specific IBC gene expression signature has not been established, these investigations have revealed certain molecular markers and signaling pathways that have consistently been linked to the pathophysiology of IBC.
Traditional Molecular Features: HER2, Hormone Receptor, TP53
Although there is a considerable focus on elucidating the unique biologic profile of IBC, the traditional molecular features of breast cancer play an important role in understanding the pathophysiology, prognosis, and treatment options associated with IBC. Clinical evaluation of patients with IBC supports the findings seen with gene expression profiles, i.e., IBC is more frequently found to be triple negative and HER2 positive (39, 40). One-hundred and eight patients with LABC from the Leuven University Hospital in Belgium were identified and classified as IBC (49) or non-IBC (59), and compared with non-IBC LABC, IBC patients were more likely to have HER2 positive disease; 35% versus 19% (p = .07) (41). In a larger registry dataset from the California Cancer Registry (1999-2007), the receptor status and clinical outcome was assessed among 2,014 IBC patients, 1,268 non-IBC LABC patients, 3,059 patients with metastatic breast cancer (MBC) and 73,758 non-T4 breast cancer patients (42). Patients with IBC were more likely to have HR negative disease (both ER and PR negative) compared with the other groups of breast cancer patients: 40% (IBC), 31% (non-IBC LABC), 25% (MBC), 18% (non-T4); whereas IBC patients were more likely to have HER2 positive breast cancer: 40% (IBC), 35% (non-IBC LABC), 35% (MBC), 22% (non-T4). Patients with HR negative IBC had an inferior overall survival (OS) and BCSS compared with HR positive IBC, whereas HER2 status was not found to be prognostic for a worse OS among patients with IBC, but was prognostic for a more favorable BCSS (hazard ratio [HR] = 0.82). These associations, i.e., a more favorable prognosis with HR positive IBC and absence of an adverse prognosis with HER2 positive disease, are also supported by retrospective data from MD Anderson Cancer Center, the Institut Gustave Roussy, and the Aga Khan University Medical Center (7, 43, 44). The more favorable disease outcome associated with HER2 positive disease is attributed to the effective therapy with trastuzumab discussed later in this chapter.
In addition to an increased incidence of HER2 overexpression, IBC is often associated with a higher frequency of mutations in the tumor suppressor gene p53 (TP53) compared with non-IBC (7). Wild-type TP53 is intimately involved in maintaining genomic stability by functioning as a transcription factor for genes involved in cell-cycle progression, DNA repair, and apoptosis. Common TP53 mutations are missense or nonsense mutations, and often result in the accumulation of p53 protein in the cytoplasm or nucleus demonstrated by immunohistochemistry (IHC); however, direct complimentary DNA (cDNA) sequencing can also detect up to 20% of TP53 mutations that are not identified by IHC (45, 46). Using these methods, Turpin et al. examined 161 patients from the Hospital Saint Louis and found 57% of the 63 IBC tumors exhibited TP53 mutations compared with 37% of the 27 non-IBC LABC (40). Another evaluation of the immunohistochemical profile of IBC using tissue microarray techniques found 45% of 86 IBC tumor specimens contained TP53 mutations versus 23% of the 552 non-IBC samples (39). This study also confirmed the increased incidence of triple negative IBC and HER2 positive IBC compared with non-IBC (54% vs. 24% and 40% vs. 12%, respectively). Some, but not all of the studies which demonstrate a prevalence of TP53 mutations in IBC have correlated this finding with an adverse prognosis (46, 47 and 48). A single institution retrospective analysis from the MD Anderson Cancer Center found that among 59 patients with IBC, 58% had TP53 positive tumors which corresponded to a shorter 5-year progression-free survival (PFS) (35% vs. 55%) and OS (44% vs. 54%); neither were statistically significant (49). A similar retrospective examination from the Japanese Foundation for Cancer Research did not find such a correlation (50).
Distinctive Molecular Features: RhoC GTPase, WISP3
Recent investigations into the unique molecular properties of IBC have elucidated several genetic features that are critical to the development of its highly metastatic phenotype. RhoC GTPase is a member of the RAS superfamily of G proteins that are involved in several aspects of cellular function including cell cycle control, cell migration and epithelial cell polarity, cell survival, and angiogenesis (51). Dysregulation of these processes is associated with increased metastatic potential in pancreatic cancer, melanoma, and breast cancer. Original exploration of differentially expressed genes in IBC was performed on the triple negative IBC cell-line, SUM149 and validated among 29 IBC and 19 non-IBC tumor samples under the direction of van Golen et al (52). This search led to the demonstration of overexpression of RhoC GTPase in 90% of the IBC specimens compared with 38% of the non-IBC tumors (p = .0095). The overexpression of RhoC GTPase was 91% concordant with a decrease in expression of WISP3 (Wnt-1 inducible signaling pathway protein 3), a member of the CCN (Cyr61, CTGF, Nov) family of tumor suppressor genes that function to modulate insulin-like growth factor-1 (IGF-1) mediated cellular growth, and proliferation (53). WISP3 was originally named LIBC or “Lost in Inflammatory Breast Cancer” because it was expressed in only 20% of the IBC specimens examined compared with 79% of the non-IBC tumors (p = .0013) (52).
Overexpression of RhoC GTPase in transfected cell lines resulted in a phenotype that mimics IBC similar to the IBC cell-line SUM149, including its tumorigenic ability (54). These experiments led to a greater understanding of the potential role of RhoC as an IBC oncogene, in addition to its known
ability to stimulate angiogenesis, aid in the formation of stress fibers and focal adhesion, and to stimulate actomyosinmediated cell contraction leading to increased cellular mobility. Recent data from the van Golen laboratory have also shown an increase in several PI3K/Akt signaling pathways due to a downstream effect of activated RhoC on PI3K/Akt-1 associated genes (55). These signaling pathways control cytoskeletal polarity and cellular mobility. C-Met activation may also contribute to enhance PI3K pathway signaling in IBC due to increased expression of c-Met expression in IBC (56).
ability to stimulate angiogenesis, aid in the formation of stress fibers and focal adhesion, and to stimulate actomyosinmediated cell contraction leading to increased cellular mobility. Recent data from the van Golen laboratory have also shown an increase in several PI3K/Akt signaling pathways due to a downstream effect of activated RhoC on PI3K/Akt-1 associated genes (55). These signaling pathways control cytoskeletal polarity and cellular mobility. C-Met activation may also contribute to enhance PI3K pathway signaling in IBC due to increased expression of c-Met expression in IBC (56).
Given the greater than 90% rate of concordance of WISP3 suppression with RhoC expression observed in human IBC, Kleer et al. transfected immortalized human mammary epithelial (HME) cells with antisense WISP3 constructs resulting in the inhibition of WISP3 and subsequent overexpression of RhoC (57). This molecular pattern of WISP3 modulating RhoC expression produced a statistically significant increase in cellular proliferation and in VEGF production. Transfection of the IBC cell-line SUM149 with WISP3 resulted in suppression of angiogenesis by reducing levels of VEGF, FGF2, and IL-6 (58). Restoration of WISP3 function also caused a decreased rate of cellular proliferation, anchorage-independent growth, and invasive capabilities manifested by reduced tumor formation when injected into athymic nude mice. One potential mechanism of WISP3 protein function is directly related to its secretion into extracellular spaces, thus functioning to regulate IGF-1 signaling leading to diminished growth of IBC cells (59). This constellation of function attributed to WISP3 supports its classification as a tumor suppressor gene of IBC.
The WISP3 negative/RhoC positive phenotype detected by both IHC and RT-PCR has consistently been found to be associated with IBC compared with non-IBC human tumor samples evaluated in Tunisia (26/41 = 66% of IBC vs. 10/86 = 12% non-IBC) (60). RhoC overexpression has also been demonstrated in 40/46 (87%) of Egyptian IBC tumor samples versus 11/64 (17%) of non-IBC tumors (61). This reliable molecular arrangement, seen in IBC, supports investigation into modifying the expression/function of RhoC or WISP3 as a therapeutic target (62).
The Pathophysiology of Tumor Emboli: E-cadherin, MUC-1, eIF4GI
The establishment of an IBC xenograft model (MARY-X) from a patient with IBC has been instrumental in elucidating some of the principal features of the pathophysiology of IBC. MARY-X was developed in 1999 and exhibited classical characteristics of erythema of the murine skin and tumor emboli exclusively involving lymphovascular spaces in nude/SCID mice (63). The carcinoma is triple negative but is EGFR (epidermal growth factor receptor) and TP53 positive. MARY-X overexpresses the membrane bound adhesion molecule E-cadherin and cell-surface MUC-1 glycoprotein up to 10-20 times greater than in non-IBC xenograft models. When further examined, the carcinoma within the lymphovascular spaces were spheroids, exhibiting significant homotypic tumor cell adhesion due to overexpression of E-cadherin along the entire membrane surface of the carcinoma (64). Although MARY-X overexpressed MUC-1, it was a dysfunctional protein, with decreased cell-surface sialyl-Lewis X/A residues which bind to E-cadherin. This contributes to the absence of tumor cell heterotypic adhesion to the endothelial cells causing visible retraction of the tumor emboli from the endothelial wall lining of the lymphovascular structures. The pattern of these effector molecules results in the ability of IBC to form tight adhesive tumor emboli that lack the ability to adhere to the endothelial wall of vasculature, thus enabling a more metastatic phenotype. Alpaugh et al. confirmed the overexpression of E-cadherin and MUC-1 in human IBC specimens and also found that the tumor emboli were retracted from the endothelial lining of the vasculature in these human samples (63, 64 and 65).
The E-cadherin adhesion complex remains structurally and functionally intact in IBC, with overexpression of its associated α-catenin and β-catenin membrane-bound proteins as part of its E-cadherin/α,β-catenin functional axis (65). In MARY-X, when this axis is disrupted, the spheroids disassociate and apoptosis occurs suggesting that the intact E-cadherin/α,β-catenin functional axis is necessary for the survival of IBC cells. These characteristic spheroids are also present and functional in the pulmonary metastasis that develops in the MARY-X xenograft, supporting the hypothesis that this structural integrity is necessary and maintained throughout the progression to metastasis that occurs in IBC.
Gene amplification results in increased MUC-1 expression whereas alterations in other factors involved in mRNA transcription and translation contribute to the higher E-cadherin levels. eIF4GI is a translation initiation factor which, when combined with eIF4E and eIF4A, forms a complex (eIF4F— eukaryotic initiation factor 4E) that contributes to the initiation of cap-dependent mRNA translation (66). Silvera et al. examined 77 breast cancer samples and found overexpression of eIF4GI in 80% of the 37 IBC specimens (67). Through a series of studies performed on the SUM149 IBC cell line, suppression or silencing of eIF4GI resulted in a reduction of E-cadherin attached to the IBC surface membrane due to increased E-cadherin degradation. Increased stability of E-cadherin occurs with membrane association through its interaction with the protein p120 catenin. Silencing of eIF4GI also results in a reduction in the translation of p120 mRNA by more than 60%. Overexpression of eIF4GI appears to be essential for the development and survival of IBC tumor emboli and supports its invasive properties by promoting increased translation of mRNAs with internal ribosome entry sites (IRESs), such as the p120 mRNA.
The Stem-cell Phenotype of Inflammatory Breast Cancer
The MARY-X spheroids and tumor emboli have been likened to the human embryonal blastocyst which overexpresses the E-cadherin axis and is the source of embryonal stem cells (ES). This similarity in physiology, i.e., the presence of cellular anchorage independence and enhanced ability to migrate and metastasize, prompted several studies that demonstrated a strong association between IBC and breast cancer stem cells. MARY-X expresses the stem-cell marker phenotype which includes CD44+/CD24-/low (100% of cells), strong CD133 surface expression (>90% of cells), high activity of aldehyde dehydrogenase (ALDH) (23% of cells) (68). Molecular pathways which sustain the stem cell state, such as Notch signaling through the Notch 3 receptor, are also increased in MARY-X compared with non-IBC carcinomas that primarily utilize Notch 1 and Notch 4 expression to enhance Notch signaling (69). Increased tumorigenicity and self-renewal was primarily evidenced in the subpopulation of MARY-X which exhibited both high ALDH expression and enhanced Notch 3 signaling.
Xiao et al. also confirmed a higher level of CD133 membrane expression and Notch 3 nuclear expression in human IBC tumor samples compared with non-IBC samples (88% vs. 8%, and 76% vs. 8%, respectively), regardless of molecular subtype of IBC. The high prevalence of CD44+/CD24-/low phenotype among human IBC was confirmed by Polyak’s laboratory where they examined tumor specimens from all
subtypes of IBC and found that >80% expressed the stem-cell phenotype of CD44+/CD24-/low, regardless of whether they were triple negative or of luminal subtype (70). In addition to the Notch signaling pathway, both the NF-κB/RAS/MAPK and the IL6/JAK2/STAT3 pathways have been found to play an important role in mammary stem cell survival and have enhanced activation in IBC, making them potential therapeutic targets for this disease (34, 70, 71, 72 and 73).
subtypes of IBC and found that >80% expressed the stem-cell phenotype of CD44+/CD24-/low, regardless of whether they were triple negative or of luminal subtype (70). In addition to the Notch signaling pathway, both the NF-κB/RAS/MAPK and the IL6/JAK2/STAT3 pathways have been found to play an important role in mammary stem cell survival and have enhanced activation in IBC, making them potential therapeutic targets for this disease (34, 70, 71, 72 and 73).
Angiogenesis and Lymphangiogenesis in Inflammatory Breast Cancer
Early investigation of IBC determined this subtype of breast cancer to have significantly greater angiogenic and lymphangiogenic properties compared with non-IBC. Histologically, Colpaert et al. found a significant increase in microvessel density as determined by the Chalkley method (p < .0001), and a greater percentage of proliferating endothelial cells (EPC) among the 35 human IBC tumor specimens compared with 104 non-IBC specimens (19% vs. 11%, respectively, p = .014) (74). A correlation was found between EPC and expression of the hypoxia marker carbonic anhydrase IX (CA IX) on tumor emboli, whose transcription is regulated by hypoxia inducible factor 1 (HIF-1). This evidence suggests an adaptation to hypoxia within the IBC tumor emboli, supported by intense angiogenesis. However, hypoxic signals are not the sole stimulant of the intense angiogenesis seen in IBC. RhoC, which is overexpressed in IBC, is a potent activator of angiogenesis, and the overexpression of eIF4GI in IBC results in the increased translation of specific IRES containing mRNA that encode VEGF, regardless of hypoxic signals (75). IBC tumor cells express a genetic profile that is associated with survival during hypoxia even when it does not exist.
RT-PCR analysis of 16 IBC and 20 non-IBC tumor specimens revealed a significantly higher expression of the mRNA involved in the Ang1/Tie2 pathway among the IBC samples (Ang-1, Tie-1, Tie-2) (76). This pathway supports the survival of endothelial cells and vascular expansion. In addition, the mRNA expression of KDR (the receptor for vascular endothelial factor A [VEGF-A]) and FGF-2 was also significantly higher among the IBC specimens, supporting a more active angiogenic process among IBC compared with non-IBC. Other molecular profiling studies of IBC supported an increased expression of genes involved in angiogenesis (32). IBC is also associated with a higher expression of all of the lymphangiogenesis mRNAs (VEGF-C, VEGF-D, Flt-4, Prox-1, Lyve-1), supporting activation of the Flt-4 signal transduction pathway that induces lymphangiogenesis. This was confirmed by the demonstration of a greater percentage of proliferating lymphatic endothelial cells among the IBC specimens compared with the non-IBC specimens. Significant investigation is ongoing in determining whether the presence of IBC tumor emboli in lymphovascular spaces occurs by classic lymphovascular invasion, or whether the IBC tumor cells stimulate the formation of encircling vascular channels from adjacent myoepithelial cells or stromal stem cells; a process known as lymphovasculogenesis. Regardless of pathophysiology, the highly angiogenic presence in IBC lends itself as an excellent therapeutic target, as described later.
TREATMENT OPTIONS FOR INFLAMMATORY BREAST CANCER
The poor prognosis associated with IBC stimulated intensive research focusing on unraveling the unique molecular biology of this disease in an attempt to develop more specific and effective therapies. These novel therapies will be discussed later in this chapter. The current therapeutic armamentarium does not differ significantly from that utilized for non-IBC LABC and the next sections will review the current tri-modality approach to treatment.
LOCOREGIONAL THERAPY FOR INFLAMMATORY BREAST CANCER
Locoregional Treatment in Inflammatory Breast Cancer: An Historical Perspective
Early surgical series of patients presenting with clinical findings consistent with IBC in the pre-chemotherapy era demonstrated consistently poor outcomes despite aggressive surgical resection. Haagensen reported his initial experience with IBC in a series of 20 patients treated with radical mastectomy (77). Due to difficulty in achieving negative margins, the rate of locoregional failure (LRF) was high at 50%. Rates of systemic failure were higher, thus survival was poor with a median survival 15.5 months after surgery and no 5-year survivors. Similar results were reported by others as summarized by Treves in an editorial where a table of 6 radical mastectomy series revealed 4 of 262 patients survived 5 years when treated by radical mastectomy alone, translating into a cumulative 5-year OS of 1.5% (78). These results supported the classification of IBC as a categorically inoperable disease because, as noted by Haagensen, “when no cures can be expected, and no definite evidence of prolongation of life can be shown, it seems entirely unreasonable to treat these patents by radical mastectomy” (77).
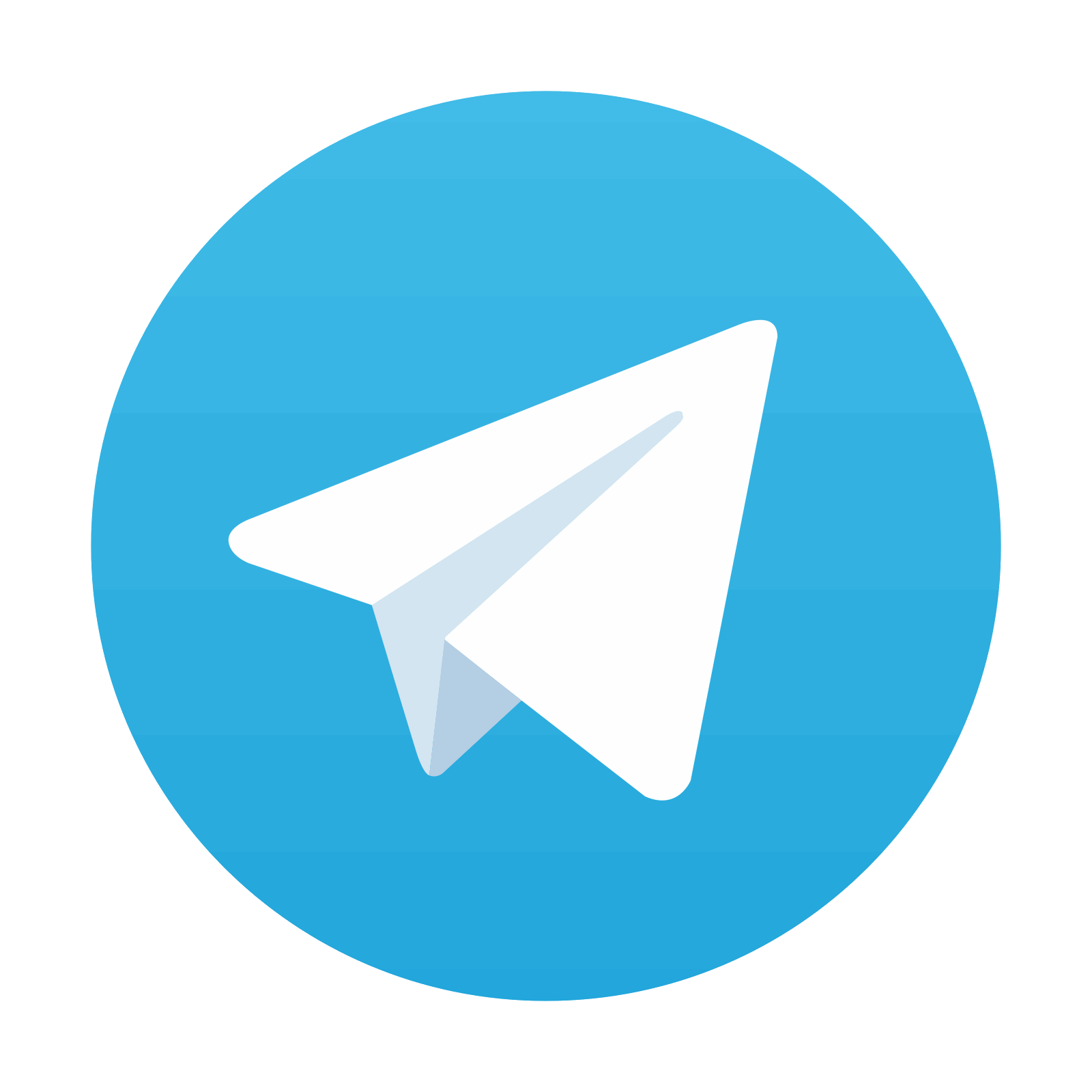
Stay updated, free articles. Join our Telegram channel

Full access? Get Clinical Tree
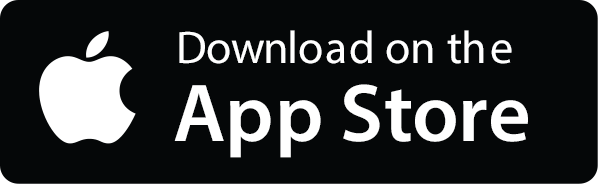
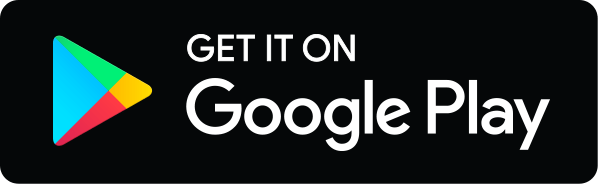