It is now more than 40 years since the first successful allogeneic hematopoietic stem cell transplantation (HSCT) for a child with severe combined immunodeficiency (SCID). In the succeeding years, HSCT for SCID patients have represented only a small portion of the total number of allogeneic HSCT performed. Nevertheless, the clinical and biologic importance of the patients transplanted for SCID has continued. SCID patients were the first to be successfully transplanted with nonsibling related bone marrow, unrelated bone marrow, T-cell depleted HSCT, and genetically corrected (gene transfer) autologous HSC. Many of the biologic insights now widely applied to allogeneic HSCT were first identified in the transplantation of SCID patients. This article reviews the clinical and biologic lessons that have been learned from HSCT for SCID patients, and how the information has impacted the general field of allogeneic HSCT.
More than 40 years ago, the first successful allogeneic hematopoietic stem cell transplantation (HSCT) was reported by Robert A. Good, MD and his colleagues for a child with severe combined immunodeficiency (SCID). In the succeeding years, HSCT for SCID patients have represented only a small portion of the total number of allogeneic HSCT performed. Nevertheless, the clinical and biologic importance of the patients transplanted for SCID has continued. SCID patients were the first to be successfully transplanted with nonsibling related bone marrow, unrelated bone marrow, T-cell depleted HSCT, and genetically corrected (gene transfer) autologous HSC. In addition, many of the biologic insights that are now widely applied to allogeneic HSCT were first identified in the transplantation of SCID patients. Therefore, this article reviews the clinical and biologic lessons that have been learned from HSCT for SCID patients, and how the information has impacted the general field of allogeneic HSCT.
Preludes
In 1956 it was established that rodents receiving total body irradiation (TBI) could be rescued from the lethality of bone marrow failure by the infusion of histocompatible bone marrow. In those studies the importance of histocompatibility for the successful rescue of the animals from lethal TBI by the prevention of graft-versus-host disease (GVHD) was identified. In the decade between the biologic reality that the transplantation of bone marrow could rescue irradiated animals and the first successful human allogeneic HSCT, clinical investigators attempted to apply the biologic principles to the treatment of patients. A sentinel event was the irradiation accident that occurred in Yugoslavia in 1959 where 6 patients, who were heavily irradiated, were subsequently treated by the infusion of either fetal liver and spleen cells or unrelated bone marrow cells. No sustained donor hematopoietic engraftment was seen in any patients, although slight increases in donor-type erythrocytes were transiently seen in some patients. The patient with the highest dose of irradiation died whereas the other patients had autologous hematopoietic recovery. Other early attempts included the use of high-dose irradiation/chemotherapy and pooled allogeneic bone marrow for the treatment of related and unrelated patients with acute leukemia. Patients with aplastic anemia were infused with bone marrow from identical twins with some patients having hematopoietic improvement, but it was unclear whether their improvement in hematopoiesis was due to the HSCT or the spontaneous recovery of their underlying aplastic anemia. Many allogeneic recipients developed acute GVHD that had similarities to GVHD seen in rodents following histoincompatible transplants. Thus, clinicians were aware that histocompatibility might improve the likelihood of successful HSCT. During the 1960s, the development of serologic reagents to detect human leukocyte antigen (HLA)-A and HLA-B permitted physicians to determine the class I histocompatibility of potential donors and recipients. The development of the mixed lymphocyte culture (MLC) permitted the determination of class II histocompatibility because no antiserum to HLA-DR existed.
Clinical advances
Allogeneic-Related HSCT
The first successful allogeneic HSCT was a member of a kindred in which 11 male infants had died due to severe recurrent infections during the first year of life. At admission, the child had draining skin pustules, no detectable lymph nodes, and lymphopenia. At that time, no phenotypic assays existed for the enumeration of T lymphocytes, but the diagnosis was confirmed by the absence of cutaneous delayed hypersensitivity as well as functional assays showing that the patient’s lymphocytes did not respond to stimulation with either phytohemagglutinin (PHA) or allogeneic cells. HLA-A and -B typing indicated that the patient and a sister were HLA-B identical but differed at one HLA-A antigen; however, the sister did not respond in MLC to stimulation with the patient’s cells. The patient was transplanted with a mixture of peripheral blood leukocytes and bone marrow. The cells were given intraperitoneally. A total dose of 3.5 × 10 8 peripheral blood leukocytes and 1 × 10 9 nucleated bone marrow cells were given. A week after transplantation, the patient developed an erthymatous rash, which on skin biopsy had histopathological features characteristic of GVHD. Stimulation of the patient’s peripheral blood lymphocytes showed the development of large lymphoblasts with a female karyotype, indicating that the circulatory lymphocytes were now responsive to stimulation by PHA and were of donor origin. The patient was challenged with dinitrofluorobenzene and responded to skin testing, demonstrating the development of normal delayed hypersensitivity.
The patient was blood group A and the donor blood group O. The patient’s anti-B titers rose, but he developed a Coomb positive hemolytic anemia. Eight weeks after HSCT the patient’s platelet and granulocyte counts began to drop, and a bone marrow aspirate showed hypocellularity with both male and female cells. The patient’s bone marrow progressed to complete aplasia with all cells being of donor origin.
Three months after the first transplant the patient was transplanted for the second time with 1 × 10 9 bone marrow cells: 20% into the right ileac marrow space and 80% intraperitoneally. The bone marrow was treated in vitro with a horse antihuman lymphoblast globulin for 2 hours before infusion. By 2 weeks there was an increase in the platelet count, and the white blood cell count began to increase. All bone marrow cells had a female karyotype. The patient is now more than 40 years old, with normal immune and hematopoietic function of donor origin.
The lessons
The authors of the initial report were not able to appreciate the significance of all their clinical and laboratory observations. The patient received peripheral blood T lymphocytes as well as bone marrow cells, and it is likely that the early onset of acute GVHD was due to the large number of donor T lymphocytes given, especially considering that the donor and patient were an HLA-A mismatch. In the second transplant to reduce the probability of GVHD, they tried to reduce the number of T lymphocytes infused by (1) taking smaller bone marrow aspiration to reduce peripheral blood contamination and (2) treating the bone marrow with antiserum to remove T lymphocytes. The patient did not develop any acute GVHD after the second transplant.
Subsequent animal experiments demonstrated that the efficiency of the intraperitoneal injection of HSC was approximately one-tenth that of intravenous injection. The present clinical use of the intravenous route for HSC infusion is based on the canine experiments performed by Thomas and his colleagues. The success of the initial transplants in the SCID patient was, therefore, due to the relatively large number of cells given, the small size of the patient, and the use of a HLA-B identical and MLC nonreactive donor.
The patient developed immune-mediated bone marrow aplasia, which was also seen in some other SCID patients during the 1970s. It is of interest that, although hemolytic anemia has been seen following ABO-incompatible or histoincompatible HSCT in SCID patients in more recent years, rarely has bone marrow aplasia occurred. The reason for this clinical change is unclear. The development of bone marrow aplasia, however, clearly demonstrated that immune cell-mediated events including GVHD can produce severe aplastic anemia, indicating that immunosuppression might have a role in the treatment of aplastic anemia, which was subsequently demonstrated in both animal studies and clinical trials using antithymocyte globulin and other immunosuppressive agents.
The patient, in addition to being the first patient to have an immune deficiency corrected by HSCT, also represented the first successful treatment of bone marrow failure by allogeneic HSCT. No evidence of donor hematopoietic engraftment occurred following the initial transplant. It is now clear that in SCID patients, no clinically significant donor HSC engraftment occurs without some myelosuppressive therapy. However, once the immune-mediated destruction of the recipient hematopoiesis had occurred and adequate “space” had been developed, it was possible even with the intraperitoneal infusion of donor bone marrow to establish donor-derived hematopoiesis without any chemotherapy. The patient demonstrated what it took another decade to formally prove, that is, that engraftment of donor HSC requires the elimination or reduction of the number of recipient HSC to permit the engraftment of donor hematopoietic cells. The present use of reduced intensity regimens that rely on the engraftment of the donor immune system to eliminate both normal and abnormal (neoplastic) recipient hematopoiesis is a direct descendant of the biologic events that occurred in the first SCID patient.
Related Nonsibling Donors
Because most SCID patients did not have an MLC-nonreactive sibling donor, clinicians began to explore other relatives to see if any potential donors were MLC nonreactive. In a limited number of cases, MLC-nonreactive donors were identified that were successfully used to treat cases of SCID. When related donors, who were MLC reactive, were used, patients usually died of acute GVHD, suggesting that MLC nonreactivity (HLA-DR locus identity with modern techniques) was a prerequisite for the successful HSCT of SCID without fatal GVHD. This approach to identifying appropriate donors was subsequently applied to other diseases as well.
The lessons
Differences at single class I alleles do not significantly decrease the overall likelihood of event-free survival, whereas class II differences are almost uniformly associated with poor outcome. Thus, the results from the early transplants for SCID were the basis for focusing on identifying donors who were MLC nonreactive or Class II identical.
Unrelated Donors
Because the majority of SCID patients did not have an MLC-nonreactive related donor, the possibility that an MLC-nonreactive unrelated donor might exist who could be a successful donor was explored. Despite the fact that formal programs to identify unrelated MLC nonreactive donors did not exist, a SCID patient, who had a prevalent haplotype, received 7 transplants from an unrelated individual who was MLC nonreactive. The donor and recipient were HLA-B identical but disparate at one HLA-A antigen. The patient was homozygous for HLA-A1 while the donor was heterozygous (HLA-A1, HLA-A2). At 5 months of age the patient received 10 × 10 6 bone marrow cells/kg by the intravenous route. The bone marrow had been shipped from Denmark to the United States. Ten days later the patient developed a macular rash consistent with GVHD, and PHA-responsive lymphocytes were detected. Three weeks later the patient received a second infusion of 10 × 10 6 cells. The patient developed detectable lymph nodes and increasingly severe acute GVHD. At 2 months the circulating donor lymphocytes disappeared, and the patient received a third transplant of 17 × 10 6 cells by the intravenous route. Again the patient developed PHA-responsive donor lymphocytes that persisted for 4 months. A fourth transplant at 13 months of age was performed with 10 × 10 6 bone marrow cells given intravenously. Again there was an increase in PHA-responsive lymphocytes of donor origin, but by 6 months after HSCT the PHA-responsive donor lymphocytes were no longer detected. Therefore, because of the possibility of hybrid resistance, the patient received 2 doses of cyclophosphamide (25 mg/kg) before HSCT, which consisted of 130 × 10 6 cells/kg of fresh bone marrow cells. The patient developed PHA-responsive donor lymphocytes and had in vitro responses to both mitogens and antigens. Donor T lymphocytes but no B lymphocytes were present in the patient’s circulation. Three months following the fifth transplant, when the patient was about to be discharged from the hospital, he developed severe aplastic anemia with all detectable residual bone marrow cells being of donor origin. Two months later, without preconditioning, the patient received frozen bone marrow cells from his fifth transplant that did not result in any hematopoietic engraftment. Therefore, 4 months later, after preparation with full doses of cyclosphosphamide (50 mg/kg × 4 days), the patient received 1 × 10 8 bone marrow cells/kg intravenously. At 2 weeks he developed donor hematopoiesis and acute GVHD. All T lymphocytes were of donor origin. B lymphocytes were detected for the first time, with spontaneous rises in his serum immunoglobulin levels. After discharge, all lymphoid and hematopoietic elements were of donor origin by both karyotyping and cell surface antigen analysis.
The lessons
Previous attempts to use unrelated bone marrow to treat aplastic anemia had been unsuccessful. This patient demonstrated that significant pretransplant immunosuppression may be necessary, even in patients with SCID, to achieve successful donor hematopoietic engraftment. The successful treatment of this patient and other SCID patients with unrelated HSCT were a major impetus for the establishment both of the National Donor Marrow Program and the international cooperation that is now available for obtaining unrelated bone marrow, mobilized peripheral blood cells, and cord blood.
Fetal Liver Cells
Based on studies from neonatally thymectomized mice, it was determined that histoincompatible HSCT could be done if the HSC inoculum was devoid of T lymphocytes capable of causing acute GVHD. Clinical investigators, therefore, attempted to identify sources of human HSC that did not contain T lymphocytes. Their attention initially focused on the potential use of fetal liver, which before 14 to 16 weeks of gestational age is a major source of hematopoiesis in the human fetus. Because no T lymphocytes are found in the circulation after 12 weeks of gestation, it was hypothesized that fetal liver obtained from electively aborted fetuses of less than 12 weeks of gestation would not contain significant numbers of T lymphocytes. Therefore, fetal liver cells could be an HSC source devoid of T lymphocytes. HLA typing was not possible before the transplantation of the fetal liver cells. Therefore, questions existed as to whether clinical benefit would be derived from the engraftment of the histoincompatible HSC. Initially, transplants with fetal liver were unsuccessful, possibly due to the use of cryopreserved fetal liver cells in most cases. The first successful immune reconstitution reported using fetal liver cells was achieved in a patient with SCID due to adenosine deaminase (ADA) deficiency. The patient received 25 × 10 8 fetal liver cells intraperitoneally when the patient was 5 months old. IgM-bearing cells were detected 19 days after transplantation, and an increase in T lymphocytes was seen by 40 days. PHA-responsive cells were present by day 74. The patient developed in vitro proliferative responses to mitogens, specific antigens (candida), and allogeneic lymphocytes. Immunization with φX174 resulted in a low primary IgM response with little IgG production after a repeat immunization. The patient developed appropriate isohemagglutinin antibodies. The patient was taken off replacement immunoglobulin and did well until 1 year of age when he developed nephrotic syndrome, from which he died.
Subsequent SCID patients without ADA deficiency were also transplanted with fetal liver cells. One patient had the correction of his T-lymphocyte immune deficiency after the transplantation of 8.4 × 8 7 fetal liver cells intraperitoneally at 13 months of age. He developed GVHD, which lasted for 6 weeks, and had the presence of normal numbers of PHA-responsive T lymphocytes by 12 weeks after transplantation. The patient developed a cutaneous response to candida antigen. Serum IgM levels rose to normal levels by 1 year, but he had no detectable IgG, requiring the continued administration of replacement immunoglobulin. However, subsequent series with larger numbers of patients confirmed the potential of fetal liver cells ± fetal thymus to correct T-lymphocyte and sometimes B-lymphocyte immunodeficiencies, but also demonstrated that durable engraftment was less than 30% with a low probably of achieving long-term immune reconstitution.
The lessons
The recipients of fetal liver cells demonstrated that fetal liver cells devoid of T lymphocytes were capable of supporting thymopoiesis without the development of GVHD. The first patient, who developed circulating B lymphocytes, had ADA deficiency. It is now known that cross-feeding can correct ADA deficiency. The investigators could not determine the origin of the circulating B lymphocytes, but they were most likely of recipient origin, while the donor-derived T lymphocytes were the source of ADA. Successful treatment of the ADA-deficient form of SCID with either exogenous enzyme therapy or HSCT results initially in increases in the number of B lymphocytes of recipient origin. Decreased primary and secondary response to φX174 stimulation suggests that there was a lack of normal T- and B-lymphocyte cooperation.
None of the initial recipients of fetal liver cells received any pretransplant chemotherapy. Therefore, it is unlikely that HSC engraftment occurred. The cells that gave rise to T lymphocytes of donor origin may thus have been derived from committed lymphoid progenitors (CLP) that were able to migrate to the recipient thymus, induce its differentiation, and differentiate into circulating T lymphocytes of donor origin. The follow-up of the fetal liver recipients should provide important biologic information about the longevity and the breadth of T-lymphocyte immunity derived from CLP.
T-Lymphocyte Depleted HSCT
In 1975 it was first demonstrated in mice that T-lymphocyte depletion of histoincompatible HSC permitted both the hematological and immunologic reconstitution of irradiated mice without GVHD. Attempts were therefore undertaken in humans to eliminate T lymphocytes from histoincompatible bone marrow using a variety of techniques, both physical and biological. The selective separation of T lymphocytes from HSC by albumin density gradients as well as the suicide of donor T lymphocytes after stimulation by recipient antigens were attempted. None of these approaches led to the correction of the immune deficiency of any SCID patients. Most patients had no signs of the engraftment of any donor cells.
The approach to T-lymphocyte depletion that was first shown to be clinically successful was the physical removal of T lymphocytes based on their agglutination with soybean agglutinin (SBA) followed by the physical rosetting of the residual T lymphocytes by sheep red blood cells (E), which had initially been used to immunophenotypically detect T lymphocytes. The combination of SBA agglutination followed by E rosette formation permitted the physical removal of the majority of T lymphocytes from human bone marrow, which could then be used for HSCT. Following preclinical studies in monkeys, patients were treated with HLA haploidentical disparate bone marrow depleted of T lymphocytes.
Of the first 6 SCID patients treated with T-lymphocyte depleted MLC-reactive paternal marrow, 5 had durable immune reconstitution, whereas GVHD was limited or nondetectable. None of the patients had chemotherapy before their engraftment. One patient had graft rejection and was successfully retransplanted after pretransplant chemotherapy.
The lessons
The clinical experience confirms the experiments in mice that T-lymphocyte depletion before HSCT could permit the engraftment of histoincompatible HSC without the development of clinically significant or fatal acute GVHD. However, pretransplant immunosuppression is required in some cases to achieve donor immune reconstitution due to the presence of either engrafted maternal T lymphocytes or hybrid resistance. The use of T-lymphocyte depleted HSCT is now in general use for both related and unrelated HSCT.
In Utero HSC Transplantation
A variety of genetic diseases (β- and α-thalassemia, adrenoleukodystrophy, Hurler disease, and so forth) can be cured or stabilized by the postnatal engraftment of normal allogeneic HSC. Some genetic diseases, however, have significant morbidity at the time of birth, suggesting that the engraftment of normal HSC before birth might provide clinical benefit to the patients. Fetuses with hemoglobinopathies have been transplanted in utero with HSC from either fetal liver or T-lymphocyte depleted parental bone marrow without any evidence of sustained hematopoietic engraftment. However, the transplants were performed in fetuses of more than 16 weeks of gestation, by which time the fetuses had T lymphocytes capable of responding to allogeneic cells.
In contrast, 2 SCID patients have been reported who were successfully transplanted with T-lymphocyte depleted parental histoincompatible bone marrow cells. In both cases, the genetic basis for the patients’ disease was defects in the common γ-chain. The first patient received a total of 18.6 × 10 6 cells intraperitoneally in 3 injections starting at 16 weeks of gestation. The second patient received 18 × 10 6 nucleated cells in 2 intraperitoneal injections beginning at 21 weeks of gestation. The clinical outcomes of both patients were similar. Both had PHA-responsive T lymphocytes of donor origin while their B lymphocytes continued to be of recipient origin. In the first case, immunizations were successful with the production of specific antibodies, whereas no information is available about antibody production in the second case. Thus, these patients with the X-linked form of SCID, who have defective natural killer (NK) cells, were able to be successfully engrafted with haploidentical T-cell depleted HSC without the development of any detectable GVHD.
The lessons
In contrast to the SCID patients, the patients with hemoglobinapathies, who have normal immune systems, were not able to be successfully transplanted with haploidentical T-lymphocyte depleted HSC even as early as 16 to 20 weeks of gestation. It is not clear as to whether the immune reconstitution that occurred in the SCID patients was due to HSC engraftment or whether the T lymphocytes are derived from CLP in the HSC inoculum. Nevertheless, the persistence of the donor lymphoid cells was achieved in the SCID patients. Sustained donor lymphoid or hematopoietic engraftment was not achieved in patients with nonimmune genetic diseases, although one patient may have died of in utero GVHD. Both successfully treated SCID patients had X-linked SCID and, therefore, an absence of functional NK cells and the ability to exhibit hybrid resistance. It would be interesting to know if patients with other forms of SCID, who had normal NK function after birth, could be successfully engrafted in utero.
Genetically Corrected HSC
The identification of the molecular basis of most forms of SCID (common γ-chain deficiency, ADA deficiency, interleukin [IL]-7 receptor deficiency, and so forth) made SCID patients logical candidates for the use of genetically corrected autologous HSC. Murine studies had demonstrated that retroviral vectors could transduce pluripotent hematopoietic stem cells as well as committed lymphoid progenitors. Thus, clinical investigators thought that transplantation of genetically corrected autologous HSC could provide all of the benefits associated with the transplantation of allogeneic HSC without the risks of acute or chronic GVHD.
The first gene to be cloned that was associated with SCID was ADA. Researchers in preclinical studies demonstrated that retroviral vectors containing the human ADA gene could transduce both murine HSC and human mature T lymphocytes. The transduction of mature T lymphocytes normalized their intracellular metabolism, demonstrating that the transduced ADA gene produced adequate levels of functioning enzyme. The first human gene transfer trial was in patients with ADA-deficient SCID, who received their own T lymphocytes that had been transduced in vitro. The patients had had adequate numbers of T lymphocytes for the transduction because they were on enzyme replacement therapy. The patients received multiple infusions of the transduced T lymphocytes. The persistence of the transduced cells could be detected for at least 7 years. It was difficult, however, to determine whether any clinical efficacy was associated with the transduced cells because the patients continued on their exogenous enzyme replacement therapy. However, no toxic effects were assessed with the infusion of the transduced T lymphocytes.
Additional patients were then transplanted with a mixture of transduced bone marrow plus transduced peripheral blood. Different retroviral vectors were used for the 2 transductions so that it would be possible to determine the source of any circulating T lymphocytes. Posttransplant analysis of myeloid cells revealed that all transduced cells contain the vector used to transduce bone marrow cells, whereas all the T lymphocytes early after transplantation were derived from the infused mature T lymphocytes. Over the course of the first year the proportion of T lymphocytes derived from the transduced T lymphocytes decreased, whereas the proportion derived from the transduced bone marrow increased, so that by 1 year all the transduced T lymphocytes contained the bone marrow vector. After the patients had their ADA replacement enzyme therapy discontinued, the frequency of their transduced T lymphocytes was 5% and of the bone marrow precursors 25%. Thus, the patients were able to have significant immune reconstitution following the transplantation of the gene corrected cells with the production of specific antibody and the generation of responses to mitogen stimulation. However, the majority of their immune function was due to nontransduced cells, demonstrating the effect of cross-correction between the transduced and the nontransduced cells.
With the identification of defects in the common γ-chain as the basis for the X-link form of SCID, preclinical research was undertaken to evaluate gene transfer. Using a retroviral vector, French investigators transplanted patients with autologous bone marrow transduced with a retroviral vector containing the human common γ-chain gene. In the majority of patients there was the rapid development of T lymphocytes containing the transduced gene as well as the ability to develop antigen-specific T-lymphocyte proliferation and the production of specific antibodies, so that patients could be removed from immunoglobulin therapy. In comparison with the results with the ADA gene transfer, all of the circulating T lymphocytes contained the transduced gene. Unfortunately, 5 patients have developed acute T-lymphocyte leukemia due to the activation of the LMO2 gene by the inserted gene. The development of leukemia has resulted in gene transfer trials for X-linked SCID being put on hold.
Because of the limited number of transduced T lymphocytes seen in the patients with ADA deficiency, Italian investigators explored the possibility of pretransplant myeloablative therapy to reduce the number of recipient HSC at the time of transplantation. Patients with ADA deficiency transplanted after reduced doses of busulfan have improved immune reconstitution compared with those with no pretransplant chemotherapy, with a larger percentage of both the myeloid cells and T lymphocytes containing the transduced gene. No cases of leukemia have been seen in the patients receiving gene transfer for ADA deficiency.
The lessons
The major difference between the ADA deficiency and X-linked SCID is that a selective advantage exists in vivo for the transduced T lymphocytes in patients with X-linked SCID, whereas no significant selective advantage for the transduced T lymphocytes exists in patients with ADA deficiency due to the cross-correction of nontransduced T lymphocytes by enzyme replacement or enzyme produced by the transduced cells. Therefore, to increase the frequency of the engraftment of the transduced HSC it was necessary to administer pretransplant myelosuppressive therapy with anti-HSC activity. The use of pretransplant myelosuppressive therapy has the associated risks of neutropenia and thrombocytopenia as well as the possibility of the later development of leukemia. Nevertheless, the use of pretransplant myelosuppressive therapy has resulted in an increased frequency of engraftment of the transduced HSC as well as an increase in the frequency of transduced T lymphocytes. The use of pretransplant myelosuppressive therapy is therefore being entertained for gene transfer trials in which the transduced cells will not have a significant selective advantage, including the hemoglobinopathies.
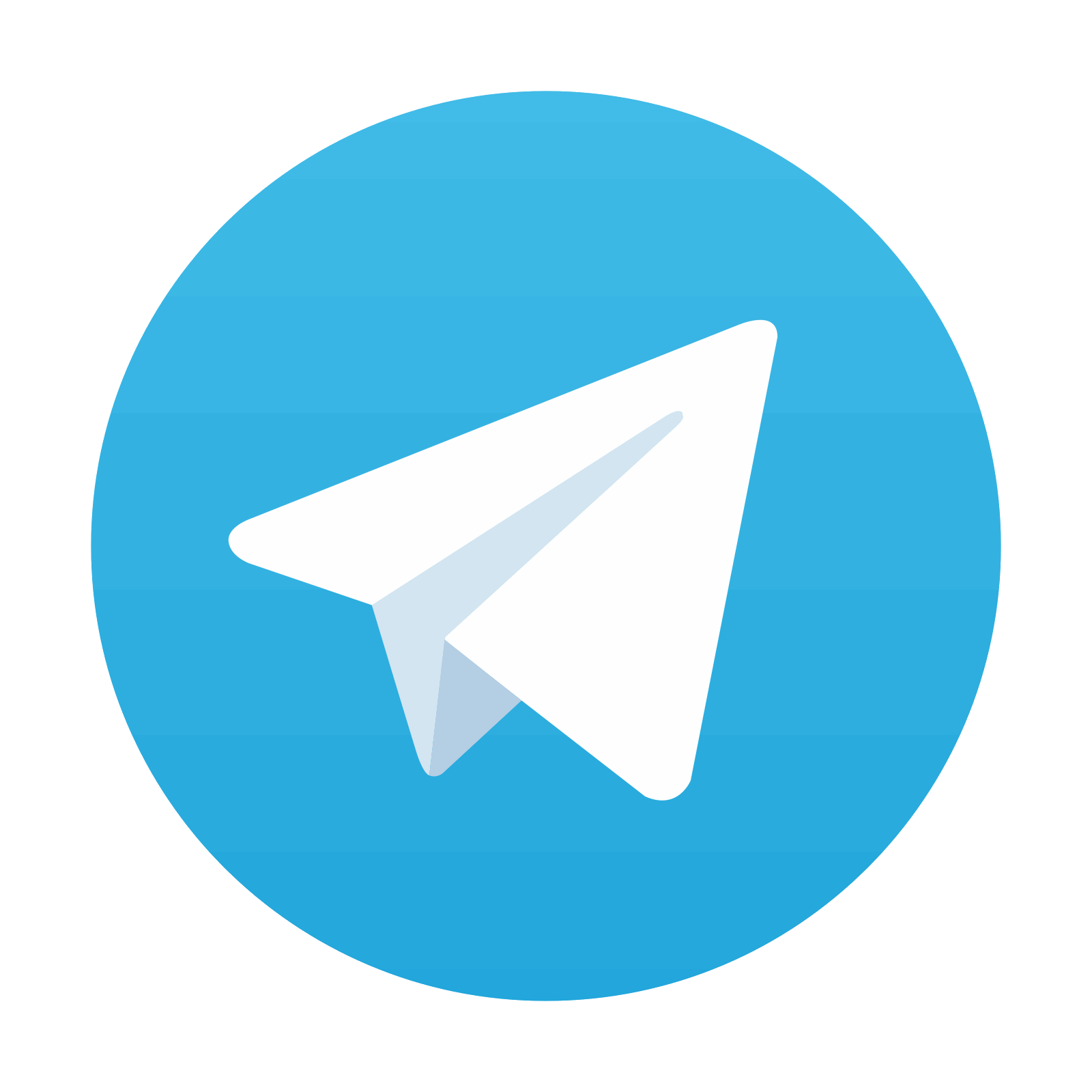
Stay updated, free articles. Join our Telegram channel

Full access? Get Clinical Tree
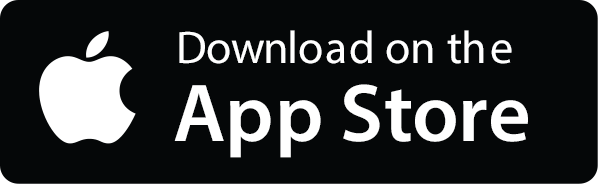
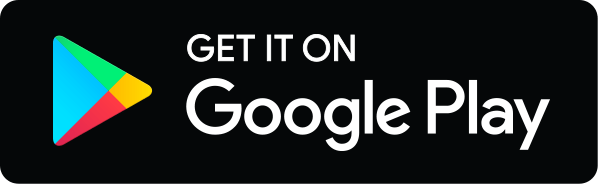