The concept of gene therapy emerged as a way of correcting monogenic inherited diseases by introducing a normal copy of the mutated gene into at least some of the patients’ cells. Although this concept has turned out to be quite complicated to implement, it is in the field of primary immunodeficiencies (PIDs) that proof of feasibility has been undoubtedly achieved. There is now a strong rationale in support of gene therapy for at least some PIDs, as discussed in this article.
The concept of gene therapy emerged as a way of correcting monogenic inherited diseases by introducing a normal copy of the mutated gene into at least some of the patients’ cells. Although this concept has turned out to be quite complicated to implement, it is in the field of primary immunodeficiencies (PIDs) that proof of feasibility has been undoubtedly achieved. There is now a strong rationale in support of gene therapy for at least some PIDs, as discussed later in this article.
Rationale
Many PIDs are lethal diseases. In the absence of treatment, severe combined immunodeficiencies (SCIDs) cause death within the first year of life. Many other combined deficiencies of adaptive immunity (such as Wiskott Aldrich syndrome [WAS] and diseases causing hemophagocytic lymphohistiocytosis [HLH]) can also be fatal in young infants. Allogeneic hematopoietic stem cell transplantation (HSCT) can cure many of these disorders by replacing the diseased hematopoietic lineages with normal ones. These results prove that transplantation of normal hematopoietic stem cells or their progenitors can correct a large variety of PIDs of the adaptive and innate immune systems. However, HSCT is associated with several serious adverse events, including the toxicity of myeloablative chemotherapy and, above all, the consequences of the potential immune conflict between donor and recipient. The latter can result in either graft failure or, conversely, graft-versus-host disease, a cause of serious morbidity and mortality. In the context of human leukocyte antigen (HLA) mismatch, the risk for immune conflict can be alleviated by removing donor T cells from the stem cell inoculum. However, this type of approach is still marred by the risk for graft failure and prolonged immunodeficiency before donor-derived immunity develops. Although advances in HSCT methodology may provide better solutions to these problems, it is clearly legitimate to search for alternative, gene-based approaches.
Most PIDs display Mendelian inheritance, so that addition of a normal copy of the mutated gene can correct the deficiency provided that the right cells are targeted and transgene expression is appropriate. At present, the pathophysiological mechanisms of many PIDs have been worked out and provide further clues to the use of gene therapy.
Information on gene expression pattern and regulation is essential for assessing the therapeutic feasibility. For instance, the tightly regulated expression of CD40L (the protein that is deficient in X-linked hyper IgM syndrome) precludes gene therapy in its present form because such precise regulatory control cannot yet be mimicked. Indeed, continuous expression of CD40L led to the development of lymphoma when tested in CD40L-deficient mice. Given that several of the genes mutated in PIDs encode proteins involved in cell survival/proliferation, their expression is expected to provide a significant growth advantage over non-corrected cells during differentiation (see later discussion). This key point was eventually verified in the first successful clinical trials.
While the pathophysiology of many PIDs was being deciphered, significant advances were also made in the development of vectors capable of efficiently transducing mature lymphocytes and progenitor cells. These new vectors (γ retroviruses, lentiviruses, spumaviruses, and possibly retrotransposons) are all characterized by their ability to integrate into the host genome so that the therapeutic transgene is replicated during cell division and thus is stably transmitted to the progeny. This property is mandatory for gene therapy in mitotic cells, such as immune cell precursors and mature lymphocytes. Initial attempts used the long terminal repeat (LTR) of retroviruses as a promoter, whereas progress toward the use of internal promoters in conjunction with deletion of the LTR enhancer activity (the so-called self-inactivated LTR [SIN-LTR]) were made to promote safety (see later discussion). Furthermore, the technical aspects of ex vivo gene transfer into hematopoietic progenitors have been improved over the years by using appropriate cytokine cocktails to put cells in cycle retrovirus (RV) or in G1 of the cell cycle lentivirus (LV) and thus facilitate vector integration. Use of fibronectin fragments also promotes cell infection by vectors. These developments made gene therapy a feasible option for some PIDs at least.
Gene therapy for severe combined immunodeficiencies -X1
The most frequent SCID is SCID-X1; the condition is caused by a deficiency in the common γc cytokine receptor subunit, which causes a complete block in T-cell and Natural killer (NK)-cell development. It was first thought that SCID-X1 was the most accurate model for assessing gene therapy because spontaneous reversion of the mutation in the γc-encoding IL2RG gene led to significant correction of the immune deficiency. This observation supported the hypothesis whereby transduced lymphocyte progenitors have a selective advantage over their non-transduced counterparts. Furthermore, IL2RG is expressed by all hematopoietic cells and is not tightly regulated, thus reducing the risk for toxicity related to aberrant expression. It was thus expected that gene correction of a proportion of lymphoid progenitors would be enough to restore at least the T-cell compartment and that the effect would be long lasting (because T cells live for several decades).
Thus, following in vitro and in vivo preclinical tests in γc-deficient mice, clinical trials were designed. The vectors were based on the use of RV constructs in which IL2GR was placed under the transcriptional control of the LTR, with the use of either an amphotropic envelope or the gibbon ape leukemia virus envelope. Between 1999 and 2006, 20 subjects with archetypal SCID-X1 were treated in two trials in Paris and then London. All the subjects lacked an HLA-identical donor. They received ex vivo transduced CD34 cells in the absence of any additional therapy. The outcome of these trials (in toxicity and then efficacy) can be summarized as follows. Five of the 20 subjects developed T-cell leukemia 2.5 to 5 years after gene therapy. In four cases, chemotherapy was easily able to destroy abnormal clones so that these children are currently in remission and doing well. However, despite chemotherapy, the one remaining subject died from refractory leukemia. The occurrence of these complications was serious enough to prompt discontinuation of the trials. Considerable efforts were made to understand the mechanism by which (with an unexpectedly high frequency) this complication had occurred. It was found that proliferative clones carried RV integrations within oncogenes loci. One oncogene in particular (LMO-2) was targeted in four out five cases. Integration of the vector containing a functional LTR enhancer had led to uncontrolled expression activity of the oncogenes. Secondary genomic modifications, such as loss of the p19Arf locus and NOTCH1-activating mutations, contributed to the clonal selection. It was then realized that the pattern of RV integration was, in contrast to previous hypotheses, semi-random with selective integration into gene loci (60% of all integrations) equally distributed between regulatory sequences 5′ from the transcription start site and the gene itself (including the first few introns). Furthermore, integrations were more frequent in genes being actively expressed in the target cells, as it is the case for several proto-oncogenes (including LMO-2) in hematopoietic progenitor cells. It thus became clear that RV-mediated gene transfer could deregulate proto-oncogene expression through the LTRs enhancer activity. It is nevertheless likely that additional factors are involved, because none of the 14 subjects successfully treated with a similar gene-therapy approach for another SCID (adenosine deaminase [ADA] deficiency, see later discussion) developed leukemic complications, despite the fact that a similar RV integrations pattern was found. These observations suggest that either γc expression by lymphoid progenitors exerts some form of synergistic effect with oncogene deregulation (although there is no evidence of γc overexpression or active downstream signaling) or that an impairment in progenitor cell distribution caused by γc or ADA deficiency influences the risk for oncogene transactivation.
Indeed, progenitor cells differ in their sensitivity to transformation, perhaps because of gene expression or activation or the cell cycle patterns. These considerations are obviously of the utmost importance for further assessment of the risk associated with gene therapy. At present, there is a consensus that use of SIN vectors featuring an internal promoter with weak enhancer activity should reduce this risk. Although this is supported by in vitro experiments, the available in vivo experimental models do not yet constitute a sufficiently predictive toxicity assay despite considerable experimental efforts. Further safety measures include the presence of insulators in the vector (to prevent the transactivation of neighboring genes) and the potential addition of a suicide gene. However, none of these modifications are likely to be absolute solutions and may carry disadvantages.
Lentiviral vectors based on HIV might be safer because they do not target 5′ regulatory responses. However, they still integrate into genes. Given the overall higher transduction efficiency of LV (as based on the number of integration sites in progenitor cells), LV and RV vectors do not greatly differ in oncogenicity. Spumaviruses may also be of value because they integrate into genes less frequently than RVs and LVs do. Nevertheless, significant issues in vector production must be solved before the effective use of spumaviruses can be envisaged.
The efficacy of γc gene transfer has been clearly demonstrated. Between 4 and 10.5 years after gene therapy, 17 of the 20 subjects are alive and display full or nearly full correction of the T-cell immunodeficiency : T-cell subset counts, sustained detection of naive T cells (even in the subjects who had been treated for leukemia), a diversified T-cell repertoire, and T-cell–mediated immune functions. The γc gene transfer led to clear clinical benefits, because patients first recovered from ongoing infections with a poor prognosis and were then able to live in a normal environment without any evidence of particular susceptibilities to infection. The extent of correction of the NK-cell deficiency was not as impressive. Despite an early rise, NK-cell counts eventually dropped to low values. Remarkably, this was also observed in patients with non-myeloablated SCID-X1 who had undergone allogeneic HSCT. A partially persistent NK-cell deficiency does not appear to be harmful. This finding indicates that NK-cell population dynamics (cell development, expansion, and survival) differ significantly from those of T cells. The frequency of transduced B cells was low (<1%) and these cells were no longer detected in the blood 2 to 3 years after gene therapy. This observation emphasizes the lack of persistence of transduced progenitors in the bone marrow. In contrast, the sustained detection of naive T cells (even in patients having undergone chemotherapy for leukemia) strongly suggests the persistence of transduced (T) cell precursors that have perhaps localized in the thymus. Despite the apparent lack of persistent γc + B cells, most patients do not require immunoglobulin substitution. Overall, these results constitute the first proof of principle of gene therapy and its sustained efficacy. As anticipated in this context, efficacy is based on the selective advantage provided by γc expression in lymphoid progenitors. These data pave the way for further use of gene therapy in patients with SCID-X1, as now scheduled in an international trial (in the United Kingdom, United States, and France) using SIN vectors. Similar attempts have been made to treat patients with atypical SCID-X1 (caused by hypomorphic mutations) or those who displayed limited T-cell reconstitution following HSCT. Effective CD34-cell transduction resulted in little or no improvement in T-cell production, probably as a consequence of thymic function loss. This parameter must therefore also be taken into account when considering gene therapy in patients who are T-cell deficient after the first few years of life.
Gene therapy for severe combined immunodeficiencies -X1
The most frequent SCID is SCID-X1; the condition is caused by a deficiency in the common γc cytokine receptor subunit, which causes a complete block in T-cell and Natural killer (NK)-cell development. It was first thought that SCID-X1 was the most accurate model for assessing gene therapy because spontaneous reversion of the mutation in the γc-encoding IL2RG gene led to significant correction of the immune deficiency. This observation supported the hypothesis whereby transduced lymphocyte progenitors have a selective advantage over their non-transduced counterparts. Furthermore, IL2RG is expressed by all hematopoietic cells and is not tightly regulated, thus reducing the risk for toxicity related to aberrant expression. It was thus expected that gene correction of a proportion of lymphoid progenitors would be enough to restore at least the T-cell compartment and that the effect would be long lasting (because T cells live for several decades).
Thus, following in vitro and in vivo preclinical tests in γc-deficient mice, clinical trials were designed. The vectors were based on the use of RV constructs in which IL2GR was placed under the transcriptional control of the LTR, with the use of either an amphotropic envelope or the gibbon ape leukemia virus envelope. Between 1999 and 2006, 20 subjects with archetypal SCID-X1 were treated in two trials in Paris and then London. All the subjects lacked an HLA-identical donor. They received ex vivo transduced CD34 cells in the absence of any additional therapy. The outcome of these trials (in toxicity and then efficacy) can be summarized as follows. Five of the 20 subjects developed T-cell leukemia 2.5 to 5 years after gene therapy. In four cases, chemotherapy was easily able to destroy abnormal clones so that these children are currently in remission and doing well. However, despite chemotherapy, the one remaining subject died from refractory leukemia. The occurrence of these complications was serious enough to prompt discontinuation of the trials. Considerable efforts were made to understand the mechanism by which (with an unexpectedly high frequency) this complication had occurred. It was found that proliferative clones carried RV integrations within oncogenes loci. One oncogene in particular (LMO-2) was targeted in four out five cases. Integration of the vector containing a functional LTR enhancer had led to uncontrolled expression activity of the oncogenes. Secondary genomic modifications, such as loss of the p19Arf locus and NOTCH1-activating mutations, contributed to the clonal selection. It was then realized that the pattern of RV integration was, in contrast to previous hypotheses, semi-random with selective integration into gene loci (60% of all integrations) equally distributed between regulatory sequences 5′ from the transcription start site and the gene itself (including the first few introns). Furthermore, integrations were more frequent in genes being actively expressed in the target cells, as it is the case for several proto-oncogenes (including LMO-2) in hematopoietic progenitor cells. It thus became clear that RV-mediated gene transfer could deregulate proto-oncogene expression through the LTRs enhancer activity. It is nevertheless likely that additional factors are involved, because none of the 14 subjects successfully treated with a similar gene-therapy approach for another SCID (adenosine deaminase [ADA] deficiency, see later discussion) developed leukemic complications, despite the fact that a similar RV integrations pattern was found. These observations suggest that either γc expression by lymphoid progenitors exerts some form of synergistic effect with oncogene deregulation (although there is no evidence of γc overexpression or active downstream signaling) or that an impairment in progenitor cell distribution caused by γc or ADA deficiency influences the risk for oncogene transactivation.
Indeed, progenitor cells differ in their sensitivity to transformation, perhaps because of gene expression or activation or the cell cycle patterns. These considerations are obviously of the utmost importance for further assessment of the risk associated with gene therapy. At present, there is a consensus that use of SIN vectors featuring an internal promoter with weak enhancer activity should reduce this risk. Although this is supported by in vitro experiments, the available in vivo experimental models do not yet constitute a sufficiently predictive toxicity assay despite considerable experimental efforts. Further safety measures include the presence of insulators in the vector (to prevent the transactivation of neighboring genes) and the potential addition of a suicide gene. However, none of these modifications are likely to be absolute solutions and may carry disadvantages.
Lentiviral vectors based on HIV might be safer because they do not target 5′ regulatory responses. However, they still integrate into genes. Given the overall higher transduction efficiency of LV (as based on the number of integration sites in progenitor cells), LV and RV vectors do not greatly differ in oncogenicity. Spumaviruses may also be of value because they integrate into genes less frequently than RVs and LVs do. Nevertheless, significant issues in vector production must be solved before the effective use of spumaviruses can be envisaged.
The efficacy of γc gene transfer has been clearly demonstrated. Between 4 and 10.5 years after gene therapy, 17 of the 20 subjects are alive and display full or nearly full correction of the T-cell immunodeficiency : T-cell subset counts, sustained detection of naive T cells (even in the subjects who had been treated for leukemia), a diversified T-cell repertoire, and T-cell–mediated immune functions. The γc gene transfer led to clear clinical benefits, because patients first recovered from ongoing infections with a poor prognosis and were then able to live in a normal environment without any evidence of particular susceptibilities to infection. The extent of correction of the NK-cell deficiency was not as impressive. Despite an early rise, NK-cell counts eventually dropped to low values. Remarkably, this was also observed in patients with non-myeloablated SCID-X1 who had undergone allogeneic HSCT. A partially persistent NK-cell deficiency does not appear to be harmful. This finding indicates that NK-cell population dynamics (cell development, expansion, and survival) differ significantly from those of T cells. The frequency of transduced B cells was low (<1%) and these cells were no longer detected in the blood 2 to 3 years after gene therapy. This observation emphasizes the lack of persistence of transduced progenitors in the bone marrow. In contrast, the sustained detection of naive T cells (even in patients having undergone chemotherapy for leukemia) strongly suggests the persistence of transduced (T) cell precursors that have perhaps localized in the thymus. Despite the apparent lack of persistent γc + B cells, most patients do not require immunoglobulin substitution. Overall, these results constitute the first proof of principle of gene therapy and its sustained efficacy. As anticipated in this context, efficacy is based on the selective advantage provided by γc expression in lymphoid progenitors. These data pave the way for further use of gene therapy in patients with SCID-X1, as now scheduled in an international trial (in the United Kingdom, United States, and France) using SIN vectors. Similar attempts have been made to treat patients with atypical SCID-X1 (caused by hypomorphic mutations) or those who displayed limited T-cell reconstitution following HSCT. Effective CD34-cell transduction resulted in little or no improvement in T-cell production, probably as a consequence of thymic function loss. This parameter must therefore also be taken into account when considering gene therapy in patients who are T-cell deficient after the first few years of life.
Gene therapy for adenosine deaminase deficiency
Adenosine deaminase deficiency is a rare, autosomal recessive PID characterized by a profound impairment in the generation of T, B, and NK lymphocytes. It is thus a particularly severe form of SCID, with early onset of infectious complications. Adenosine deaminase deficiency results in a purine metabolism defect; the accumulation of adenosine, deoxyadenosine and their metabolites (notably deoxyadenosine triphosphate [dATP]) induces the premature death of lymphoid progenitor cells. It is a disease with broad consequences, because ADA deficiency also impairs (to a varying extent) bone, brain, lungs, liver functions and perhaps the epithelia. It is thus one of the SCIDs with the worst overall prognosis. Adenosine deaminase deficiency was the first PID in which gene therapy was tested. The initial approach was ex vivo ADA gene transfer into peripheral T cells that were obtained from patients undergoing enzyme substitution therapy. Although this approach failed to reconstitute immunity, it demonstrated the feasibility of gene transfer and the long-term viability (>10 years) of transduced CD8 T cells at least in one subject. Adenosine deaminase deficiency was also the first PID in which researchers tested ex vivo ADA gene transfer mediated by an RV vector in CD34 progenitor cells from bone marrow and cord blood . At that time, gene-transfer technology was not efficient enough to ensure sufficient progenitor cell transduction and correction of the PID. Nevertheless, long-term detection of a few transduced T cells was confirmed. Concomitant enzyme substitution therapy also optimally reduced the transduced cells’ selective advantage.
In the modern era of gene therapy, efficient ex vivo ADA gene transfer mediated by an RV vector in CD34 cells has been achieved. When combined with a partially myeloablative conditioning regimen (4 mg/kg busulfan), this protocol corrected the PID in 14 out of 20 patients treated once enzyme substitution had been withdrawn (or was not initiated). Sustained correction has now been observed for up to 8 years, with clear-cut clinical benefits and no toxicity (see earlier discussion). Because of the administration of busulfan, it was found that transduced cells were detectable not only in T populations (all of which were transduced) but also in NK, B, and myeloid populations. The degree of T-cell correction in ADA deficiency does not appear to be as complete as in SCID-X1; this is probably a consequence of additional effects of ADA deficiency, such as damage to the thymic epithelium, that are not amenable to correction by ex vivo gene transfer into CD34 cells. In any case, the reproducible, sustained correction of the consequences of immunodeficiency in two SCIDs clearly proves the feasibility of this approach and suggests that, provided oncogene transactivation can be prevented, ex vivo gene transfer is an alternative to HSCT in the absence of available HLA-identical donors.
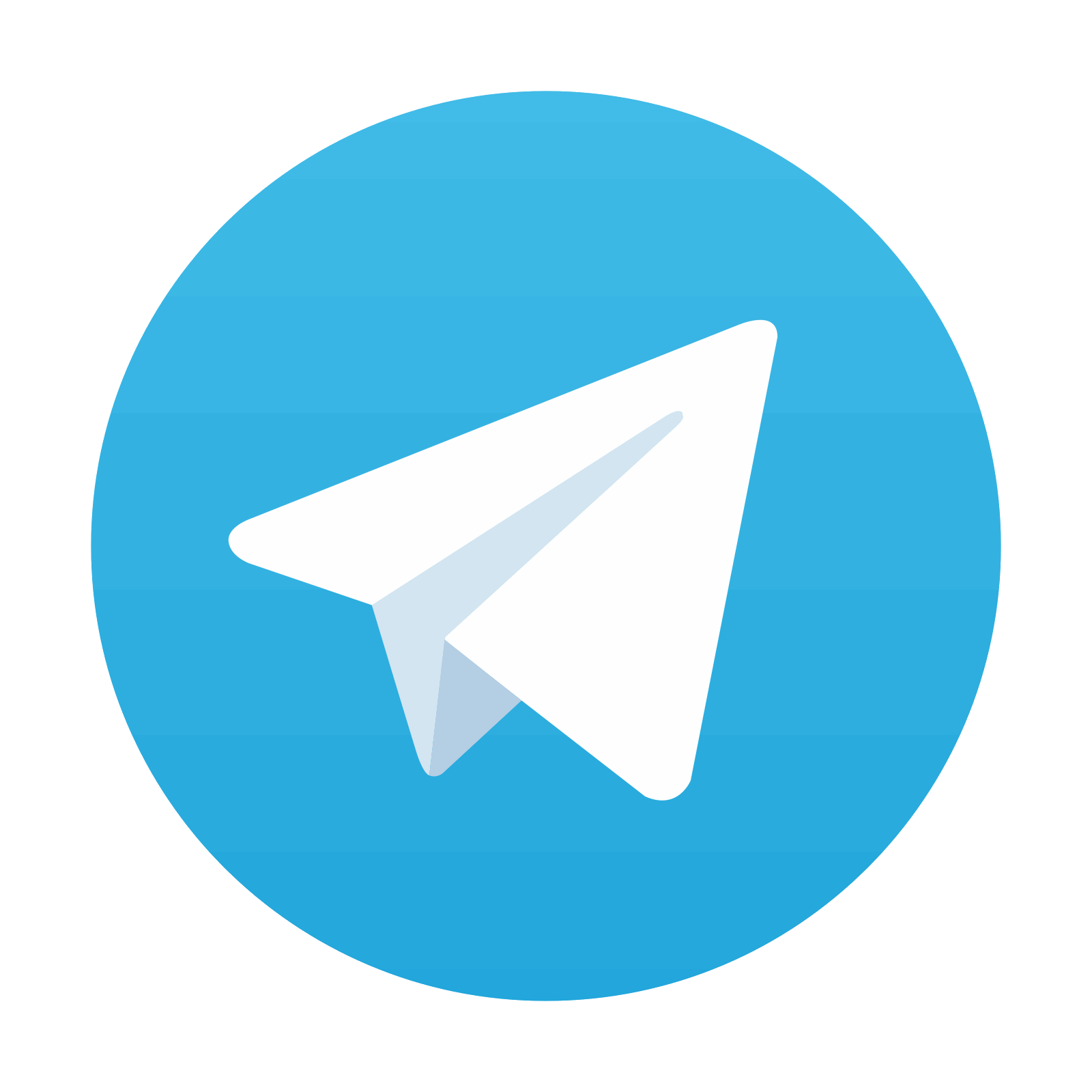
Stay updated, free articles. Join our Telegram channel

Full access? Get Clinical Tree
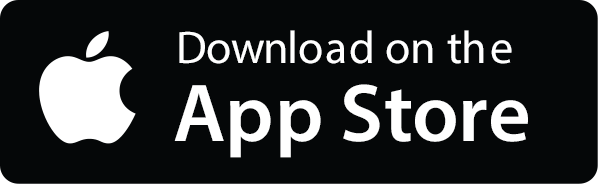
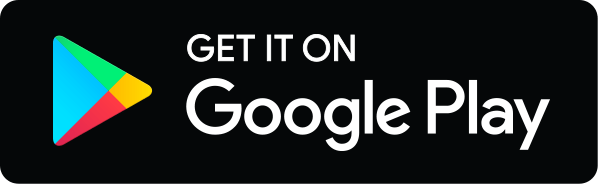