Late effect
Radiotherapy dose
Type of systemic therapy
Bone and muscle
Hypoplasia
Bisphosphonates [93]
Trismus
RT dose >40 Gy to pterygoid and masseter muscles [51]
Osteoradionecrosis
Skin
Severe dermatitis
RT dose >40 Gy [50]
Permanent epilation
Mean RT dose >46 Gy to hair follicles [53]
Necrosis/ulceration
RT dose >70 Gy [10]
Teeth
Growth disturbances
Cyclophosphamide
Vincristine
Vocal cords
Vocal cord dysfunction secondary to head and neck RT
Mean RT dose to the larynx, and lateral pharyngeal wall >65 Gy [20]
Vocal cord dysfunction secondary to recurrent laryngeal nerve injury
Salivary gland
Xerostomia
Stem cell transplantation [15]
Ear
Sensorineural hearing loss
Cisplatin (cumulative dose >360 mg/m2)
7.2.2.1 Skin and Mucous Membranes
The skin and mucosa exhibit early epithelial damage and delayed permanent vascular injury that are dependent on the total radiation dose, the fraction size, and the volume of irradiated tissue. Early radiation injury to the skin is directly attributable to the effect of ionizing radiation on the stratum germinativum cells [27]. Release of vasoactive substances results in increased capillary permeability and dilatation that manifest as skin erythema [78]. An increase in melanin-containing cells at 2–3 weeks enhances pigmentation. Moist desquamation that occurs 3–4 weeks from the initiation of treatment has been found to correlate with the development of severe delayed telangiectasias [3]. In some situations when patients are neutropenic from chemotherapy, the acute effects of radiotherapy on the skin and mucous membranes may be more pronounced.
Late radiation effects are primarily caused by fibrosis and vascular damage, particularly to small vessels. Arterioles become narrow as a result of myointimal proliferation and destruction of capillaries and sinusoids [25]. Delayed histologic manifestations of these changes include fibrin deposition, ulceration, and fibrosis. Telangiectasias are caused by endothelial cell depletion and basement membrane damage that cause capillary loops to contract into distorted sinusoidal channels. While the incidence of mucositis in patients being treated with chemotherapy approaches 40 %, stomatotoxicity resulting from individual chemotherapeutic agents, such as methotrexate, doxorubicin, 5-fluorouracil, bleomycin, and cytosine arabinoside, has not been associated with long-term effects [77]. However, when administered in conjunction with radiotherapy, or when given in high doses as required prior to stem cell transplantation, acute injury may be enhanced, resulting in an increased risk for long-term damage. Mucositis is the end result of a multistep process that begins with direct damage to the DNA of basal epithelial cells by chemotherapeutics and/or radiation and the generation of reactive oxygen species [81]. This damage leads to upregulation of transcription factors (i.e., nuclear factor-kappa beta proteins, NF-KB; wnt; and p53) causing the production of proinflammatory cytokines and enzymes (i.e., tumor necrosis factor-alpha, TNF-α). Mucosal injury is then amplified by both feedback loops and activation of macrophages by bacteria colonized within the mouth. Once ulcers have formed, the majority will heal on their own within 2 weeks if caused by standard chemotherapy. Those patients with mucositis secondary to radiotherapy and/or chemoradiotherapy may require 4 or more weeks after therapy is completed for recovery.
7.2.2.2 Bone and Connective Tissue
Irradiation of the growing bone causes injury to actively dividing mesenchymal cells, osteoblasts, and endothelial cells [26]; it also causes impairment of the osteoid formation. The long-term injury observed in irradiated growth centers includes atrophy, fibrosis of marrow spaces, and lack of osteocytes. Impaired vascularity and fibrosis of both the periosteum and endosteum can occur.
Rat models have been used to determine the mechanism of action that chemotherapeutics have on bone formation. Doxorubicin administration leads to thinning of the growth plates [27, 86]. Corticosteroids alter bone formation by suppressing osteoblastic activity [27, 91]. Historically, bone alterations secondary to methotrexate have been better investigated and are caused by disruptions of the growth plate because of reduced cartilage formation and decreased bone formation by direct toxicity of the agent to osteoblasts and bone marrow osteoprogenitor cells [27].
Toxicity within the soft tissues may also be affected by radiation. Fibrosis occurs as a consequence of increased fibroblast proliferation, combined with collagen deposition, in children whose craniofacial structures are irradiated [28]. Hypoplasia also occurs. Mucosal atrophy, reduced tissue vascularity, and tumor effects predispose to osteoradionecrosis, chondronecrosis, and soft tissue necrosis, particularly with high radiation dose/time and large irradiated volume. Interstitial implants and intraoral techniques further enhance the likelihood of such outcomes. In children receiving conventional fractionation radiotherapy (1.8–2 Gy per day), the dose of radiation to cause musculoskeletal impairment varies from approximately 20 Gy where hypoplasia of the developing muscle and bone can occur to 60 Gy where osteonecrosis and fracture may develop.
7.2.2.3 Salivary Glands and Taste Buds
The parotid, submandibular, and sublingual glands are the major salivary glands. Other (minor) salivary glands are variably distributed throughout the oral cavity and pharynx. In the resting state, saliva production comes primarily from the submandibular gland. With food intake, 60 % of the saliva may originate from the parotid gland. The composition of saliva produced is characteristic of the specific gland. Parotid saliva production is primarily serous, while the minor salivary glands secrete a predominantly mucous fluid that is more viscous. The submandibular and sublingual glands produce mixed mucous and serous secretions. Radiation damages the serous cells to a greater extent than it does the mucous cells and epithelium of ducts. Histopathologic changes 10–12 weeks after initiation of RT to doses of 50–70 Gy consist predominantly of serous acini loss, mild fibrosis, dilatation and distortion of ducts, and aggregation of lymphocytes and plasma cells [10]. Clinically, however, a decrease in the amount of salivary production can be seen at lower RT doses. When the salivary flow rates were measured in 88 patients with head and neck cancer, most glands that received a mean dose >24–26 Gy produced no saliva after 1 month and had no improvement in salivary production by 1 year after RT [22]. There is little evidence that standard doses of chemotherapy have a long-term effect on salivary gland function; however, a recent study noted the presence of xerostomia in the long-term survivors of stem cell transplantation in which busulfan, not radiation, was used in the preparative regimen [15, 58].
Modification in taste occurs as a result of changes in oral mucosa and saliva [57]. Patients retain the perception of sweet and salt more readily than that of sour and bitter. Dietary changes thus enhance dental decay in an environment already conducive to caries production [5]. Although the taste buds are considered relatively radioresistant, some taste alterations may be caused by damage to the microvilli.
7.2.2.4 Teeth
Radiotherapy (RT) effects on dentition are influenced by the developmental stage of the tooth, with the most severe disturbances occurring in children younger than 6 years of age [12, 47, 80]. Prior to morphodifferentiation and calcification, irradiation may result in agenesis. Direct irradiation at a later stage may cause microdontia, enamel hypoplasia, incomplete calcification of enamel, and arrested root development. Chemotherapeutic agents, such as cyclophosphamide, vincristine, and vinblastine, have also been shown to affect dentition, resulting in hypodontia, enamel hypoplasia, microdontia, and root malformation with the greatest individual risk factor for dental abnormalities being treated prior to the age of 5 [1, 14, 24, 40, 44, 48, 63, 66, 75, 82]. A report from the Childhood Cancer Survivor Study showed that RT dose >20 Gy was associated with dental abnormalities [49].
7.2.2.5 Ear
Children who present with primary tumors of the head and neck area or brain frequently encounter radiation to the external, internal, and middle ear during the course of their treatment [64]. Hearing loss may occur secondary to tumor involvement and extension to the auditory apparatus and may be treatment related (Fig. 7.1). There can be effects on the otic structures, both during the treatment sessions and months to years following therapy. The immediate effect on the ear is desquamation of the columnar epithelium, which lines the ears and covers the ossicles, leading to edema of the mucosa within the ear. Altered production of cerumen, in conjunction with epithelial desquamation, leads to plugging of the ear canals that may persist long after completion of therapy. More chronic effects from fibrosis and scarring can lead to chronic radiation otitis and hearing loss. Hearing loss secondary to radiation therapy is usually permanent and can be sensorineural or conductive, depending on the structures affected by the radiation. Direct effects of radiation on the cartilaginous structures can lead to stenosis or necrosis of the ear canal and external ear.
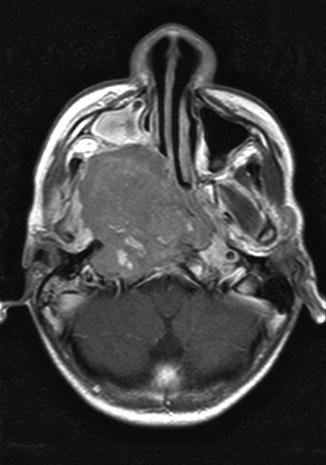
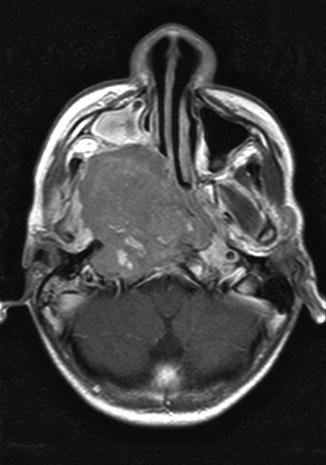
Fig. 7.1
A 13-year-old male who presented with hearing loss (right > left), headaches, and nasal bleeding. Axial, T1-weighted, post-contrast MRI shows a large right-sided nasopharyngeal mass. Hearing loss was exacerbated after cisplatin chemotherapy radiation to the primary tumor site
As noted in Table 7.1, cisplatin and carboplatin have dose-dependent, long-term sensorineural effects that are most prominent in the high-frequency ranges (which can be affected significantly by radiation – see Chap. 8). For cisplatin, approximately 40–60 % of children may experience bilateral, irreversible hearing loss [62], and toxicity has been reported within 72 h of drug infusion [6]. Changes in hearing are thought to be secondary to both direct DNA damage and the generation of reactive oxygen species within the cochlea. However, as the presentation and degree of toxicity varies in patients treated with similar doses of platinum-based compounds, many groups have begun to investigate the role of pharmacogenomics and genetic variants in cisplatin-associated ototoxicity [62].
Although rare, retinoids (i.e., isotretinoin) have also been implicated in acute and permanent hearing abnormalities ranging from tinnitus to hearing loss [76].
7.2.2.6 Vocal Cords
There are a limited number of studies that have evaluated the progression of pediatric vocal cord development. Hartnick et al. described the pathologic changes seen in necroscopy sections from children 0 to 18 years of age and noted that at birth, only a hypercellular monolayer within the lamina propria exists [38]. It was not until the ages of 11–12 that three distinct layers could be found on the autopsy samples: a hypocellular superficial layer, a middle layer consisting of elastin, and a deeper layer of collagen fibers (Table 7.2). Although only microscopic analysis was studied, the assumption proposed by the authors was that an increasing complexity of mechanical stimulation required of the vocal cords as children grow leads to cellular differentiation [38]. This suggests that patients with head and neck tumors may be more at risk for vocal cord injuries secondary to direct tissue toxicity or neuronal toxicity during early development.
Table 7.2
Development and maturation of the vocal cord according to age
Age | Developmental event |
---|---|
Birth | Hypercellular monolayer within the human vocal fold lamina propria |
2 months | Cellular differentiation and development of bilaminar structure (hypocellular superficial layer and deeper hypercellular layer) |
11 months | Three-layer structure (superficial hypocellular layer below by epithelial layer and a deeper hypercellular layer) is first noted and found in approximately 20 % of subjects |
7 years | Three-layer lamina propria structure seen in all cases |
11–12 years | Classic vocal ligament is identified with maturation of vocal folds and fiber deposition. There now exists the classic pattern of a hypocellular superficial layer followed by a middle layer of predominantly elastin fibers and a deeper layer of predominantly collagen fibers |
To date, the most common cause of unilateral or bilateral vocal cord paralysis is trauma to the recurrent laryngeal nerves. Due to its longer length and proximity to many thoracic structures, unilateral paralysis is more likely to occur on the left. Patients with unilateral paralysis are typically hoarse, but may present with symptoms of stridor. For patients with bilateral paralysis, clinical symptomatology is directly related to the position of the cords: airway obstruction occurs if the cords are closer together (near midline), as compared to aspiration, hoarseness, or the inability to speak if the cords are further apart (lateral positioning). Patients that suffer from vocal cord paresis, in which some function of the cords is maintained, may present with high-pitched stridor and a normal voice as the adductor muscles typically work better than the abductor muscles with this type of injury.
Although rare, acute vocal cord paralysis has been reported after treatment with vinca alkaloids and includes both unilateral [7] and bilateral [2] paralyses. It is speculated that the neuropathogenesis secondary to vincristine is due to the disruption of microtubule formation after it binds to α-tubulin [2].
7.3 Clinical Manifestation of Late Effects
7.3.1 Skin and Mucous Membranes
Mucosal atrophy after conventionally fractionated doses of 60–70 Gy over a period of 6–7 weeks is common, but necrosis, chronic ulceration, and bone exposure rarely occur unless large daily doses are delivered or the total dose exceeds 70 Gy in 7 weeks [10]. Thrombosis of small blood vessels in the submucosa results in ischemia and the consequent appearance of ulcers and telangiectasias. This condition may become apparent as soon as 6 months after irradiation or as late as 1–5 years and is irreversible. Scarring and fibrosis of the nasal mucosa can alter sinus drainage and predispose patients to persistent rhinosinusitis. Children may complain of symptoms of chronic sinusitis, which include chronic nasal discharge, postnasal drip, headache, and facial pain. Smell acuity is significantly affected by radiation treatment of the olfactory mucosa, and, although this is not usually voiced as a specific complaint, it can contribute to decreased appetite and poor nutrition.
Severe skin reactions, including permanent hyperpigmentation, telangiectasias, and skin ulcerations, are rarely seen with the use of modern-day megavoltage RT, unless the skin is intentionally treated with a high dose. A recent study showed that increasing RT dose and skin volume receiving >40 Gy were associated with the severity of radiation dermatitis in children receiving RT for sarcoma [49]. Another study compared children with nasopharyngeal carcinoma treated with conventional and intensity-modulated RT (IMRT). Both grade 3 skin (47.1 % vs. 5.3 %) and mucous membrane (52.9 % vs. 15.8 %) were found to be higher in the conventional RT compared to IMRT-treated patients [51]. Doxorubicin and actinomycin can interact with radiation to produce severe skin reactions and may contribute to late skin effects. When these drugs are given early in the course of radiation, such reactions may be seen after low doses of 20–30 Gy. These and other chemotherapeutics (i.e., capecitabine, gemcitabine, melphalan, docetaxel), if delivered after radiation, can be associated with either “radiation sensitivity” or “radiation recall,” in which skin reactions appear in the treated field [8, 19, 35]. Typically radiation sensitivity is defined by a time interval of <7 days between the end of radiation and the start of chemotherapy, and recall is reserved for intervals greater than 1 week apart [8]. The skin often remains chronically dry due to damage to the sebaceous and eccrine glands. The sebaceous glands are as radiosensitive as the basal epithelial cells of hair follicles; eccrine glands are less sensitive [36].
Epilation within the treatment field usually occurs 2–3 weeks into the course of radiation treatment. The permanency of the hair loss depends on the total dose of radiation delivered to the hair follicles, and this, in turn, depends on the treatment technique and beam energy. Single fraction doses of 7–8 Gy or more and total doses (after fractionated therapy) of greater than 45 Gy can result in permanent hair loss [53]. Hair loss is common with chemotherapy. After chemotherapy treatments have been concluded, hair begins to regrow within 1–2 months. It may be lighter in color and have a finer texture [26]. Microscopic analysis of hair samples of patients receiving chemotherapy has shown fracture and splitting of the hair cortex and decrease in diameter and depigmentation of the hair shaft, all of which may account for the changes in color and texture [65].
7.3.2 Bone and Connective Tissue
Clinical manifestations of radiation include hypoplasia, deformities, fracture, and necrosis [39, 73]. The age at which RT is given is the most important factor determining orbital growth retardation in tumors like retinoblastoma. As the orbit has three growth spurts, the first between 0 and 2 months, the second between 6 and 8 months, and the third during adolescence, radiation in children younger than 6 months of age is more damaging to orbital growth than if administered at an older age [43].
The craniofacial development of children is affected, resulting in reduced temporomandibular joint mobility, growth retardation, and osteoradionecrosis [13]. Impaired growth of the mandible and facial bones can contribute to malocclusion. Eventual fibrosis of the temporomandibular joint results in muscle pain and headaches [14]. Tumor invasion of the temporomandibular joint, surgery, and the use of large daily fractions further increase the risk of radiation-induced trismus. Combined modality therapy has a greater impact on facial structures when radiation doses are high as children receiving doses of 24 Gy or less to the temporomandibular joint have not demonstrated clinical signs of trismus [56]. Maxillary and mandibular hypoplasias are also common dento-maxillofacial defects after chemoradiation. Linear cephalometric values suggest that the growth of the mandible may be more affected than that of the maxilla [60].
Overall, the facial skeleton appears to be the most susceptible to high radiation doses before age 6 and at puberty, which are critical times of skeletal development. In a study of 26 children receiving a mean dose of 54 Gy for either nasopharyngeal cancer or rhabdomyosarcoma, cephalometric measurements utilizing CT showed deviations in the cranial vault, anterior and mid-interorbital distances, and lateral orbital wall length, compared with normal skulls [17]. Figure 7.2 shows an example of bony and muscular hypoplasia after surgery and two radiotherapy courses for rhabdomyosarcoma.
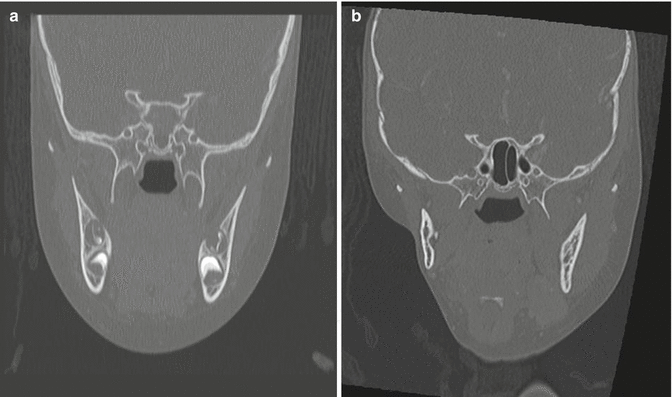
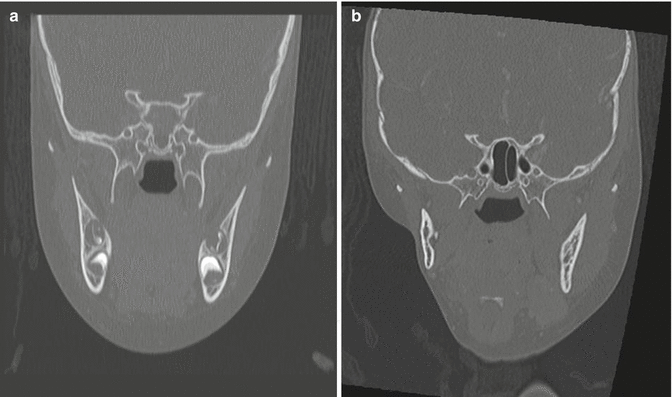
Fig. 7.2
(a) Reformatted CT with contrast of the neck in a 3.5-year-old female prior to therapy. (b) The image details both bony and soft tissue changes including hypoplasia of the right hemi-mandible and pterygoid and masseter muscles in a 7.5-year-old female with a history of recurrent right cheek rhabdomyosarcoma. She received 5,040 cGy to the area at 2 years of age, and after a local recurrence, with positive post-resection margins, she received another 4,140 cGy to the right cheek
Chemotherapy may also affect the growing skeleton. A recent review of the alterations in height for long-term survivors of acute lymphoblastic leukemia noted that chemotherapy administration was associated with a decrease in height during treatment; that the use of intensive chemotherapy leads to a long-term decrease in height that can be worsened if patients also received radiotherapy; and that young children are more at risk for severe height loss [88].
Rhabdomyosarcoma of the head and neck is a condition in which the long-term side effects of combined modality therapy have been extensively studied. Late side effects in children treated with combined modality therapy for head and neck rhabdomyosarcoma are usually seen within the first 10 years after treatment [68], given that the most will have experienced their pubertal growth by that time. Clinical or radiographic dentofacial abnormalities have been observed in 80 % of long-term survivors [24]. Abnormalities including enamel defects, bony hypoplasia/facial asymmetry, trismus, velopharyngeal incompetence, tooth/root agenesis, and disturbance in root formation were the most common findings. The largest report on the late effects in pediatric head and neck rhabdomyosarcoma comes from IRS II and IRS III, in which 213 patients were followed for a median length of 7 years. Seventy-seven percent had one or more late sequelae, including poor statural growth, facial and nuchal asymmetry, dental abnormalities, and vision/hearing dysfunction [74].
Cosmetic effects become more apparent as normal growth proceeds in adjacent unirradiated areas. In 1983, Guyuron et al. reported on 41 patients who had been treated as children with RT to the head and face. They noted that hypoplastic development of the soft tissue and bone was a common finding [37]. Irradiation of the cranial base was often correlated with soft tissue deficits in the upper face and midface. Soft tissue was more vulnerable to RT than growing facial bones, with a threshold dose as low as 4 Gy (in contrast to 30 Gy for facial bones). In 1984, Jaffe reported on the maxillofacial abnormalities seen in 45 patients who had been treated as children with megavoltage RT for lymphoma, leukemia, rhabdomyosarcoma, and miscellaneous tumors [44]. Forty-three of the 45 patients also received chemotherapy (including vincristine, actinomycin D, cyclophosphamide, methotrexate, 6-mercaptopurine, prednisone, procarbazine, or nitrogen mustard in various combinations). In 82 % of the radiated patients, dental and maxillofacial abnormalities were detected, including trismus, abnormal occlusal relationships, and facial deformities. The most severe radiation deformities were seen in younger patients who received higher radiation doses.
Paulino found that 11 of 15 children treated for head and neck rhabdomyosarcoma with RT and chemotherapy developed facial asymmetry in the RT field at doses between 44 and 60 Gy [68]. Sonis studied 97 patients with acute lymphoblastic leukemia (ALL) who received either chemotherapy alone or with 18–24 Gy cranial RT [80]. The treatment fields routinely included the temporomandibular joints, posterior tooth buds, and the ramus of the mandible. A significant dose-effect relationship was seen between 18 and 24 Gy (2 Gy/fraction). Children under the age of 5 who received 24 Gy of cranial RT and chemotherapy had a 90 % incidence of craniofacial abnormalities, but no craniofacial abnormalities were seen in children over the age of 5 or in those receiving only 18 Gy of cranial RT and chemotherapy. No craniofacial abnormalities were noted after chemotherapy alone. It is unlikely that chemotherapy alone contributes to bony or soft tissue abnormalities, although it clearly does affect dental development in relation to age at treatment [59, 63]. Although amifostine, a radioprotective agent, has been demonstrated to reduce craniofacial growth inhibition in immature rabbits, evidence for its applicability in humans is lacking [29].
Radiation therapy also has an effect on wound healing that may be critical for those who require a surgical procedure in the irradiated region [18]. RT may also affect the connective tissues and bone, leading to fibrosis and osteoradionecrosis. In the Fromm series, two patients developed temporomandibular joint fibrosis with limitation of jaw motion [30]. While many studies have documented the appearance of bone hypoplasia in the dose range of 18–24 Gy, limited information is available regarding RT dose to the mandibular muscles (pterygoid and masseter) and its effect on jaw dysfunction including trismus. Krasin and colleagues found that for each 10 % of mandibular muscle volume treated above 40 Gy, a 2 mm reduction in jaw depression was seen [50]. Osteoradionecrosis has been well described in the adult head and neck literature; however, little has been written on its incidence in the pediatric population. Osteoradionecrosis usually develops in the mandible, and its risk is directly correlated with total radiation dose, fractionation dose, tumor size, and bony involvement by the tumor. In the adult literature, osteoradionecrosis is uncommon at mandibular doses <60 Gy. The incidence of osteoradionecrosis for adult head and neck cancer patients ranges from 5 % to 10 % in most series [54]. In one study, the incidence was 1.2 % for all head and neck and 5.5 % for oral cavity sites using IMRT. Maximum mandibular dose >70 Gy and mean mandibular dose >40 Gy correlated with dental extractions after IMRT [34]. In a small single-center review of the long-term effects of IMRT and platinum-based chemotherapy in pediatric patients with nasopharyngeal carcinoma (NPC), Louis et al. noted that while all patients experienced toxicity in at least three body systems, two of the five patients developed osteoradionecrosis of the mandible [55]. Historically, this risk is increased in patients who received postirradiation dental extraction, compared with pre-irradiation extraction. It is believed that radiation is associated with decreased blood flow and oxygen levels, consequently compromising tissue repair. An example of osteoradionecrosis in a nasopharyngeal patient treated with radiotherapy is shown in Fig. 7.3.
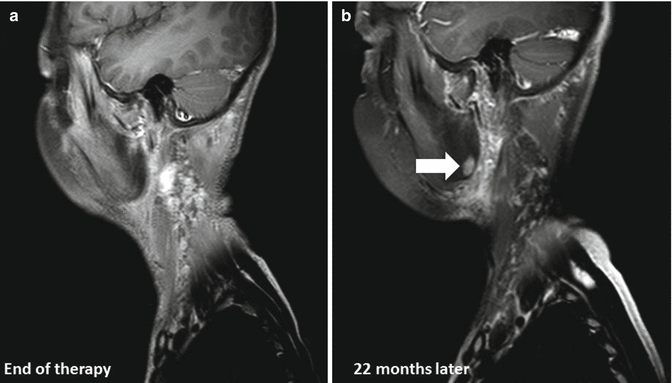
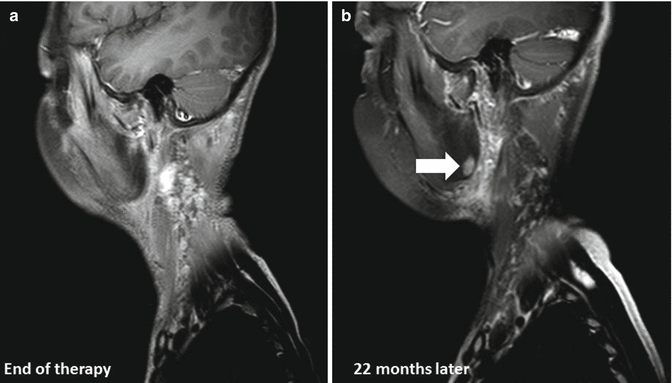
Fig. 7.3
(a) End of therapy scan. (b) Development of osteoradionecrosis in the right mandibular angle (white arrow) in a 13-year-old male with a history of stage III nasopharyngeal carcinoma 22 months after treatment with induction chemotherapy and cisplatin-based chemoradiotherapy, delivering 61.2 Gy in 34 fractions
Bisphosphonate-related osteonecrosis of the jaw has also been reported. The median onset of bisphosphonate-related osteonecrosis of the jaw was 21 months with zoledronic acid, 30 with pamidronate, and 36 with zoledronic acid and pamidronate [93].
7.3.3 Salivary Glands and Taste Buds
Salivary gland dysfunction may occur when one or more of the major salivary glands are irradiated. Permanent damage can lead to xerostomia, predisposing to dental caries, decay, and osteoradionecrosis. Studies of salivary function in children after RT are limited [52]. Fromm found that 8 of 11 parotid glands that received >45 Gy to more than 50 % of the gland volume failed to secrete saliva, whereas all parotid glands receiving 40 Gy retained the ability to secrete [30]. More recent studies in adult patients have shown a lower dose-response effect. In 2010, Deasy summarized the data from published manuscripts evaluating salivary function as related to radiation dose-volume parameters and noted that a minimal reduction of function is seen with doses to the parotid glands of <10–15 Gy, a gradual decrease in function with doses between 20 and 40 Gy, and severe (>75 %) reduction with doses >40 Gy [16]. The use of amifostine, as a radioprotector for xerostomia, is gaining popularity in the pediatric oncology community despite limited experience in children. Currently, patients on Children’s Oncology Group Protocol ARAR0331, treatment of nasopharyngeal cancer with neoadjuvant chemotherapy followed by concomitant chemoradiotherapy, are given amifostine prior to their daily RT dose. This practice is an extrapolation of results from randomized trials in adult oncology which shows the efficacy of amifostine in reducing acute and late xerostomia without compromising locoregional disease control [79, 92].
Chemotherapy for children with acute leukemia alters salivary function [58]. Mansson-Rahemtulla and colleagues showed decreased thiocyanate concentration in saliva following cytotoxic chemotherapy, which can lead to alteration in function of the salivary peroxidase system, as well as increased oral complications. Patients who undergo bone marrow transplantation are also at risk. Xerostomia, as has been noted in patients with chronic graft-versus-host disease, can persist for as long as a year and results in a high risk for developing dental caries [4]. Although only one of five children with NPC reported in the Louis et al. cohort reported xerostomia immediately after chemotherapy, four of five had symptoms noted during their long-term follow-up [55].
7.3.4 Teeth
Late effects on dentition in children can be attributed directly to the cytotoxic effects on the growing tooth buds and indirectly to salivary gland damage. Salivary gland damage results in a pronounced shift toward highly acidogenic and cariogenic oral microflora, which promotes dental caries [10]. The severity and frequency of long-term dental complications due to RT are related to the type of RT given, the total dose, the size and location of RT fields, and the age of the patient. Growing tooth buds may be arrested with <10 Gy, while doses >10 Gy can completely destroy buds [57]. Root shortening, abnormal curvature, dwarfism, and hypocalcification are noted with doses of 20–40 Gy [44, 80].
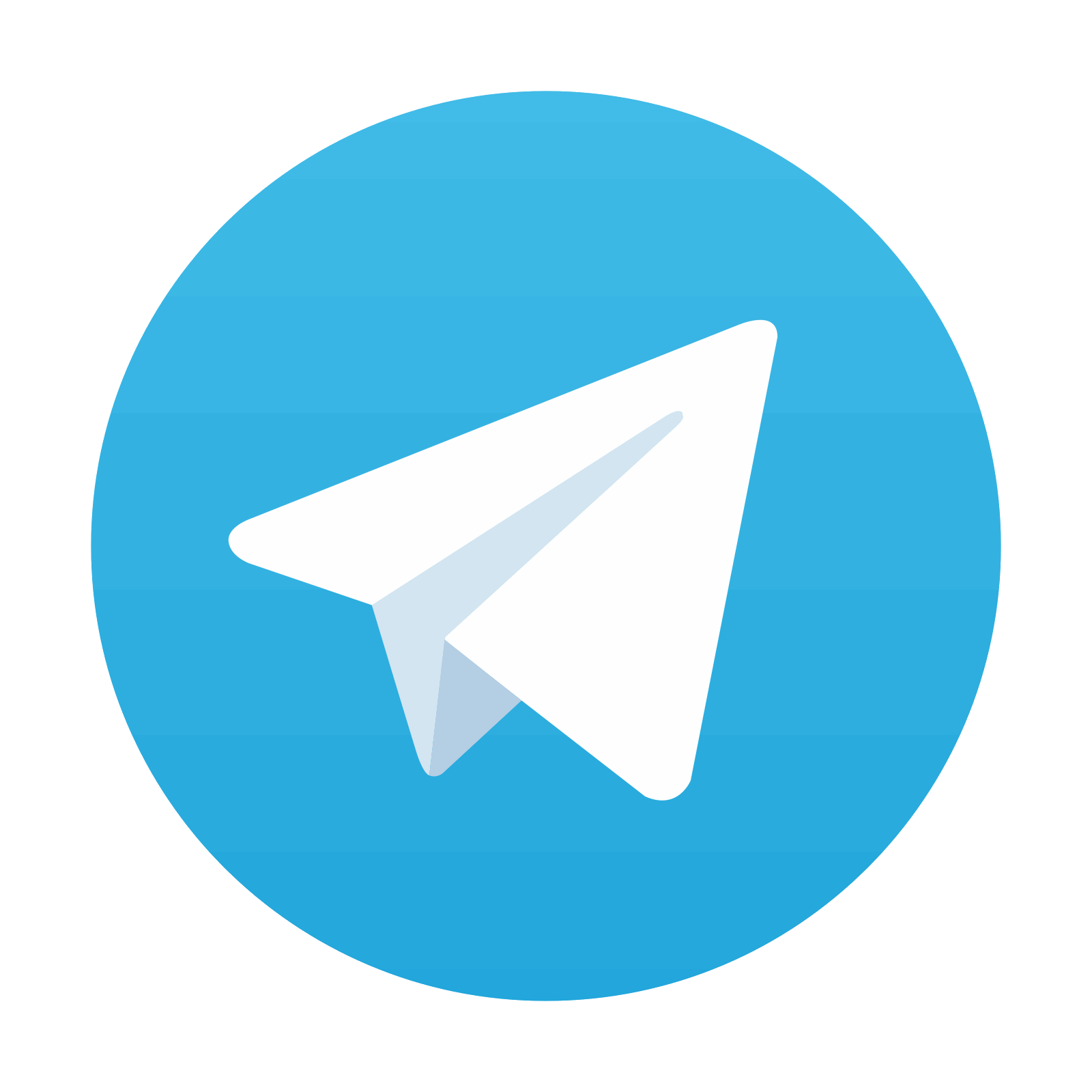
Stay updated, free articles. Join our Telegram channel

Full access? Get Clinical Tree
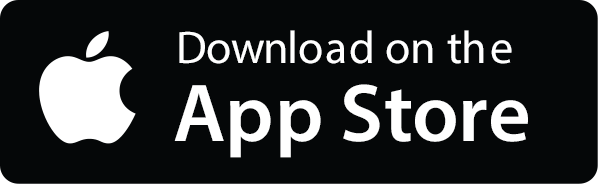
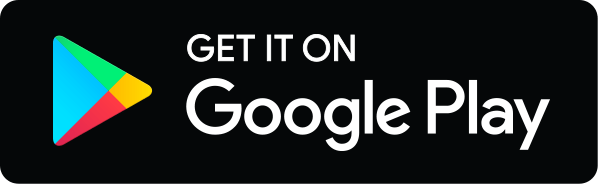