The reproductive consequences of cancer potentially affect a large number of patients in the United States. Between the years 2006 and 2010, there were 175,214 people between the ages of birth and 45 years old that were diagnosed with cancer in the United States.8 Of these, 20,506 were diagnosed between the ages of birth and 19 years old. Both males and females share the burden of cancer development from birth to 39 years of age, with males having a risk of cancer in that age group of 1 in 69, whereas females have a risk of 1 in 46.9 In women, the risk of developing breast cancer specifically within this age range is 1 in 202. The creation of patient advocacy groups such as RESOLVE and LIVESTRONG both underscore the importance of these issues. Furthermore, quality of life committees in professional oncology groups also support the importance of issues such as these to cancer patients.
This chapter will first review the effects of cytotoxic agents in men, women, and children. Next, the effects of cranial irradiation with respect to gonadal dysfunction will be addressed. Subsequently, the preservation of fertility in males and females will be reviewed in the context of both standard of clinical care and experimental technologies. Finally, the genetic and pregnancy outcome concerns after treatment will be addressed.
EFFECTS OF CYTOTOXIC AGENTS ON ADULT MEN
Biologic Considerations
The testis consists of the seminiferous (or germinal) epithelium arranged in tubules and endocrine components (testosterone-producing Leydig cells) in the interstitial region between the tubules. The seminiferous tubules contain the germ cells, which consist of stem and differentiating spermatogonia, spermatocytes, spermatids, sperm, and the Sertoli cells, which support and regulate germ cell differentiation. The testicular vasculature is permeable, and drugs can freely reach the Leydig and Sertoli cells and the spermatogonia, which are at the outer rim of the tubules. Many chemotherapeutic drugs may penetrate the Sertoli cell barrier, albeit at a lower intensity, and damage late-stage germ cells.10
Among the germ cells, the differentiating spermatogonia proliferate most actively and are extremely susceptible to cytotoxic agents. In contrast, the Leydig and Sertoli cells, which do not proliferate in adults, survive most cytotoxic therapies. These cells, however, may suffer functional damage. Frequently, after cytotoxic therapies, germ cells appear to be absent and the tubules contain only Sertoli cells, a state described as germinal aplasia. This could be a result of killing of the spermatogenic stem cells, the loss of the ability of the somatic cells to support the differentiation of a few surviving stem cells, or a combination of the two.
After cytotoxic treatment, sperm count diminishes with a time course that depends on the sensitivities of the different spermatogenic cells and their kinetics of maturation to sperm.11 Because the later-stage germ cells (spermatocytes onward) are relatively insensitive to killing and progress through spermatogenesis, sperm count is not immediately affected. However, these later-stage cells are susceptible to the induction of mutagenic damage, and studies in rodents have shown they can transmit mutations induced in their DNA to the next generation.12 The eventual recovery of sperm production depends on the survival of the spermatogonial stem cells and their ability to differentiate. Surviving stem cells can remain in the testis but fail to differentiate to sperm for several years after cytotoxic insult.13 It is important to note that human studies have not necessarily shown an increased rate of congenital anomalies.6,7
The loss of germ cells has secondary effects on the hypothalamic–pituitary–gonadal axis. Inhibin secretion by the Sertoli cells declines, and because inhibin limits follicle-stimulating hormone (FSH) secretion by the pituitary, serum FSH rises. Germinal aplasia reduces testis size, and testicular blood flow is consequently reduced, which results in the distribution of less testosterone into the circulation (Table 141.2).14 Because testosterone is a negative regulator of luteinizing hormone (LH) secretion by the pituitary, and LH is the primary stimulator of testosterone synthesis by the Leydig cells, LH increases to maintain constant serum testosterone levels.
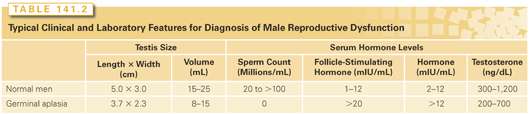
Characteristics of Gonadal Toxicity
Although subnormal semen profiles, ejaculatory dysfunction, and low libido can often be results of cancer treatment, a thorough examination should be done to determine whether symptoms of these problems might have been present before treatment. A sexual and reproductive history should be taken, including information on developmental factors such as ages at testicular descent and puberty; surgery or injury to the genitals; diseases that might affect reproduction; drug, chemical, or heat exposure; and pretreatment fertility and libido status. A physical examination should consist of an examination of the testicles and secondary sexual characteristics such as beard and hair distribution pattern. If there are indications, a semen analysis and a hormone profile should be done and results should be compared with normal values (see Table 141.2).
Cytotoxic therapies in the form of chemotherapy or radiation can have varying effects on the sperm quantity and quality. A recent study of testicular germ cell cancer patients looked at patients treated with less than or equal to two cycles of chemotherapy, more than two cycles of chemotherapy, and irradiation.15 For all three groups, the sperm count nadir was at 3 months posttreatment. This is expected because it is the time required for differentiating spermatogonia to become sperm. In the group of patients treated with two or less of bleomycin, etoposide, and cisplatin (BEP), the sperm counts returned to pretreatment levels by 12 months, whereas in the other two groups, it took 24 months to return to pretreatment levels. Another recent study shows similar results with the beginning of normalization of seminal parameters by approximately 18 months posttreatment.16
The induced azoospermia can be either temporary or prolonged, depending on the survival of stem spermatogonia and their ability to proliferate, differentiate, and produce spermatozoa, which in turn depends on the nature of the cytotoxic agent and dose (Table 141.3).17–20 If treatment is limited to the cytotoxic agents that do not kill stem spermatogonia or block their differentiation, normospermia is usually restored within 3 months after the cytotoxic therapy. However, if agents that kill stem spermatogonia or affect differentiation are used, longer periods of azoospermia ensue.13,21
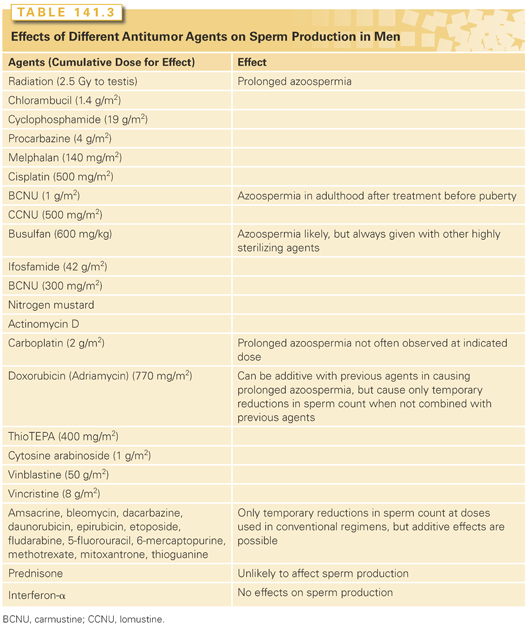
To evaluate the effects of cytotoxic therapy, one must consider the initial gonadal status. Men with testicular germ cell tumors have impaired semen quality even before cytotoxic therapy is instituted. Approximately 65% are oligospermic (counts <20 million/mL) and approximately 20% are azoospermic,22 whereas the values are 9% and 1%, respectively, in control populations. In half of the patients with reduced semen quality, this impaired testicular function is a result of abnormalities in the remaining gonad, such as carcinoma in situ or a history of cryptorchidism. In the other half, it may be a result of reversible factors such as chorionic gonadotropin production by the tumor with resulting increases in estradiol levels or the trauma of the recent orchiectomy.23 In addition, emission and ejaculation may have been compromised by earlier RPLND so that the sperm density may not accurately reflect gonadal function.24 In contrast to the severe dysfunction seen in cases of testicular cancer, in Hodgkin’s lymphoma, between 16% and 50% of patients are oligospermic, only 2% to 8% are azoospermic, and the distribution of counts in the remainder is not significantly different from normal.25,26 Poor semen quality was more prevalent in patients with advanced than with early-stage Hodgkin’s lymphoma, but specific risk factors have not been identified. In patients with other lymphomas and sarcomas, pretreatment sperm counts tend to be normal except for similar modest increases in the incidence of oligospermia.13,21
The age at treatment is not a major factor in recovery from gonadal damage in men. Most studies have failed to show any age effect,13,21 but a few have indicated increased testicular damage after cytotoxic therapy in older men.27
Individual Drugs
The most sterilizing drugs are the alkylating agents (with the exception of dacarbazine) and cisplatin. The cumulative dose appears to be more important than the dose rate in determining whether sperm production will recover (see Tables 141.3 and 141.4). Chlorambucil28 and cyclophosphamide29 induce prolonged azoospermia when given alone. The gonadal toxicities of other agents have generally been deduced from the effects of combination regimens; the doses given might be underestimates because there may be additive contributions from other drugs in the regimen. Procarbazine30 and cisplatin in high doses31 are also highly sterilizing. Busulfan has an additive effect on the sterility resulting from cyclophosphamide treatment.32 The addition of high doses of ifosfamide (46 g/m2) to cisplatin-containing chemotherapy regimens significantly reduced the recovery of spermatogenic function.33 Carboplatin, an analog of cisplatin, appeared in the same combination regimens to produce less sterility than cisplatin.22 The treatment of boys with carmustine (BCNU) and lomustine (CCNU) produced prolonged azoospermia,34 and it is likely, but not proven, that the same would occur in adults. The agents that have only temporary effects were also identified from single-agent and combination chemotherapy regimens.
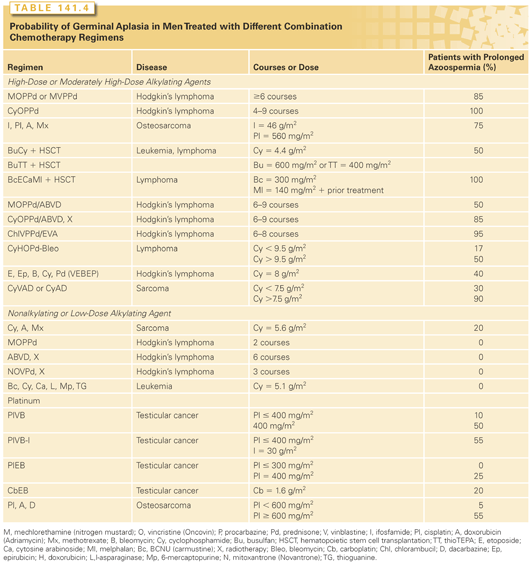
Radiation Therapy
The effects of radiation on the testes depend on the fractionation regimen. Whereas in all other organ systems, fractionation of radiation reduces the damage, radiation doses to the germinal epithelium of the testis given in 3- to 7-week fractionated courses cause more gonadal damage than single doses.35 In the usual fractionated regimens, doses to the testes above 0.15 Gy are required to produce any reduction in sperm count. Doses between 0.15 and 0.5 Gy cause oligospermia.36 Above 0.6 Gy, azoospermia occurs. The duration of azoospermia is dose dependent, and recovery can begin within 1 year after doses of less than 1 Gy, but not until more than 2 years after delivery of 2 Gy. Cumulative doses of fractionated radiotherapy of more than 2.5 Gy generally result in prolonged and likely permanent azoospermia. However, after a single dose of 8 Gy or fractionated doses of 10 to 13 Gy, given as total body irradiation in preparation for bone marrow transplantation, sometimes also with cyclophosphamide (4.5 g/m2), spermatogenesis eventually recovers in 15% of the patients.20
Testicular androgen production is relatively resistant to irradiation: doses of over 18 Gy are required before an effect is evident, and major damage does not occur until the dose exceeds 30 Gy.37 The radiation-associated impotence after such treatments is likely a result of vascular damage.
Combination Regimens
The sterilizing potential of each combination chemotherapy or chemotherapy plus radiotherapy regimen is the additive effects of the doses of the individual agents given (see Table 141.3); there is no evidence for synergistic interactions between the agents. Although it is impossible to predict whether sperm production in any given man will recover, the probability of prolonged azoospermia has been determined for many combinations (see Table 141.4). The sterilizing potential of new regimens can be predicted from the information in Table 141.3 and the results found with similar combinations. The additive effects of agents can be seen in the case of cyclophosphamide: When cyclophosphamide is given alone, 19 g/m2 is required to produce prolonged sterility in half the men, but only 15 g/m2 is required when cyclophosphamide is given with a median doxorubicin dose of 450 mg/m2 in the cyclophosphamide, doxorubicin, vincristine, and prednisone (CHOP) regimen, and only 11 mg/m2 is required when it is given with a median doxorubicin dose of 880 mg/m2 in the cyclophosphamide, vincristine, doxorubicin, and dacarbazine (CY[V]ADIC) regimen.13,21
EFFECTS OF CYTOTOXIC AGENTS ON ADULT WOMEN
Biologic Considerations
Gonadal cell kinetics in women are opposite to those in men: the germ cells are nonproliferative, whereas the somatic cells proliferate. Female germ cells only proliferate prenatally, and by birth, are arrested at the oocyte stage. At birth, there are 1 million oocytes, which are reduced to 300,000 at puberty and to fewer than 1,000 oocytes when menopause occurs at approximately age 50 years.38
Before recruitment to the process leading to ovulation, oocytes are found in primordial follicles with few pregranulosa cells, surrounded by theca cells of the ovarian stroma. The stimulus for the initiation of follicular maturation depends on local growth factors, not the gonadotropic hormones. Once a follicle is recruited into growth, it develops until it either degenerates or ovulates. Follicular maturation is characterized by proliferation of granulosa cells and the development of steroidogenic potential of both the theca and granulosa cells. Estrogen production involves the stimulation of both these cell types by LH and FSH and causes the LH surge that triggers ovulation. The cyclic variations in FSH, LH, and estradiol are essential for menstrual cycles and reproduction.
Because the destruction of oocytes results in loss of follicles, germ cell loss leads directly to estrogen insufficiency. Radiation and chemotherapy appear to cause this germ cell loss by direct apoptotic action on the oocytes in mouse models.39 Although the direct action on oocytes may be the dominant mechanism in the most gonadotoxic agents, other mechanisms such as effects on granulosa cells, surrounding stroma, and blood vessels may indirectly affect the oocyte.40 When maturing follicles are destroyed by cytotoxic therapy, the result is oligomenorrhea or temporary amenorrhea. If the number of primordial follicles is reduced below the minimum necessary for menstrual cyclicity, irreversible ovarian failure and menopause (>12 months without a menstrual period) occur.
Characteristics of Gonadal Toxicity
Because the size of the germ cell population in women cannot be determined, menstrual and reproductive histories are important in assessing the effects of cytotoxic therapy on ovarian function. Information on the patient’s sexual function and menstrual histories during and after therapy is particularly useful (Table 141.5).41 Because the mechanisms of temporary and irreversible treatment-related amenorrhea are different, a posttreatment follow-up must be given to evaluate the significance of the outcome. To determine if effects are related to cytotoxic therapy, pretherapy ovarian function should be evaluated from the history of menarche, menses, pregnancies, and oral contraceptive use. The symptoms of recent primary ovarian failure, including hot flashes, night sweats, insomnia, mood swings, irritability, vaginal dryness, dyspareunia, decreased libido, and bladder infection, should be recorded. Laboratory measurements of hormone levels are most definitive in the diagnosis of primary ovarian failure. FSH level is the most sensitive, but LH and estradiol levels are also useful (Table 141.5). Antimüllerian hormone (AMH), a hormone produced by growing follicles from the primary to antral stage, is another hormone that can be used to determine ovarian reserve by inferring the number of primordial follicles present. AMH has been shown to be a useful marker of ovarian reserve in both prepubertal and postpubertal girls after gonadotoxic treatments.42 The physical symptoms and the changes in hormone levels associated with ovarian failure may be masked by oral contraceptive use or hormone replacement therapy.
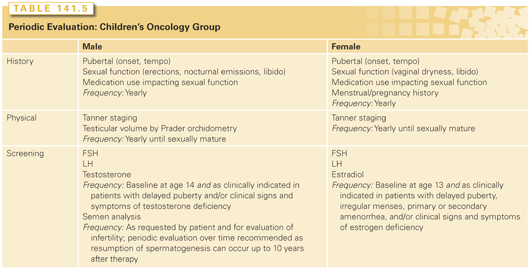
Cytotoxic therapy often induces temporary amenorrhea, which may occasionally last several years.43 Some patients with treatment-induced temporary amenorrhea do display menopausal symptoms. The permanent amenorrhea may begin during chemotherapy or, subsequently, after several years of oligomenorrhea.44 Factors resulting in temporary amenorrhea during treatment appear to be age independent.45 In contrast, the incidence of permanent treatment-induced amenorrhea (ovarian failure) dramatically and continuously increases with age at treatment (Tables 141.6 and 141.7), as expected from the decreasing number of follicles with increasing age.
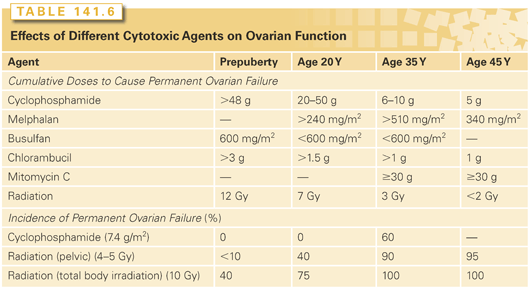
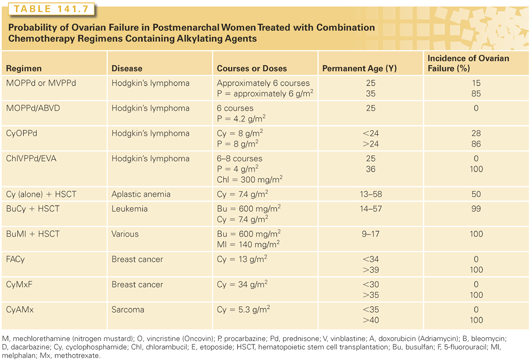
Childhood cancer survivors who retain ovarian function after treatment have an increased relative risk of 13 for developing premature menopause.46 When women aged 13 to 19 years are treated with alkylating agent chemotherapy and radiotherapy below the diaphragm, their median age at menopause is 32 years, compared with 44 years for women treated with either radiotherapy or chemotherapy alone and 49 years for controls.47
Cytotoxic therapy–induced ovarian failure accelerates bone density loss. However, when only chemotherapy is used, some residual ovarian function may be present despite the menopausal state, and the rate of bone density loss is less than when pelvic radiotherapy is used.48
Individual Drugs
In women, as in men, alkylating agents appear to be the most likely to produce permanent gonadal failure (see Table 141.6). The cumulative dose appears to be more important than the dose rate, as evidenced by similar incidences of ovarian failure in women who received similar total doses of cyclophosphamide, given either daily over several months49 or within 4 days.50 High doses of cisplatin (≥600 mg/m2) also produce permanent ovarian failure.51 Procarbazine is likely to be highly sterilizing inasmuch as permanent ovarian failure is observed after treatment with several different procarbazine-containing combinations (see Table 141.7).
Nonalkylating agents are thought to be far less likely to induce permanent ovarian failure. No ovarian failure was observed with 5-fluorouracil (30 g),49 methotrexate (200 g) plus vincristine (40 g),52 etoposide (5 g),53 or cisplatin (<450 mg/m2) plus doxorubicin (<400 mg/m2)51 in women aged 15 to 35 years. Doxorubicin (300 mg/m2), bleomycin, vincristine, and dacarbazine (ABVD) do not appear to induce ovarian failure because none was observed after this treatment of women up to age 41 years.54
Radiation Therapy
Radiation therapy induces permanent ovarian failure with marked age dependence in sensitivity (see Table 141.6). Irradiation of the para-aortic nodes for Hodgkin’s lymphoma results in cumulative ovarian doses of only 1.5 Gy and does not appear to interfere with menstruation in most patients.55 Total nodal irradiation for Hodgkin’s lymphoma or pelvic radiation for cervical cancer results in an ovarian dose of 4 to 5 Gy when proper transposition (oophoropexy) and shielding of the ovaries are performed, which preserves fertility in some younger women.56 Total body irradiation in preparation for bone marrow transplantation delivers 8 to 12 Gy to the gonads, which destroys ovarian function in nearly all adult women.50,57 Ovarian damage from radiation appears to be independent of whether it is given in one or six fractions.32
Combination Regimens
All combinations that include procarbazine and other alkylating agents (mechlorethamin, vincristine, procarbazine, prednisone [MOPP]; CHOP; mechlorethamine, vinblastine, procarbazine, and prednisone [MVPP]; and chlorambucil, vinblastine, procarbazine, and prednisone [ChlVPP]) induce ovarian failure in almost all older women and even in some younger ones (see Table 141.7). Ovarian function is maintained better by reducing the doses of these drugs through use of the MOPP/ABVD regimen.58 Radiation and MOPP chemotherapy have additive, but not synergistic, effects, and the combination results in a higher incidence of ovarian failure than either modality alone.59
Similarly, regimens containing cyclophosphamide also produce ovarian failure; however, the age dependence obscures any possible dose dependence. The treatments used for leukemia, which involve lower doses of cyclophosphamide and other agents that do not destroy primordial follicles, allow for the recovery of menses in women under the age of 35 years.60 Combination chemotherapy regimens without alkylating agents do not produce ovarian failure.
EFFECTS OF CYTOTOXIC AGENTS ON CHILDREN
Extent of Gonadal Toxicity in Boys
The germinal epithelium in the prepubertal testis is not any more resistant to cytotoxic therapy than that in adults. When chemotherapy doses to boys are expressed appropriately on a per-meter-squared basis and radiation doses are calculated, the sterilizing effects of a variety of chemotherapy61 and radiotherapy62 regimens can be predicted on the basis of their effects on adult testes (see Tables 141.3 and 141.4).
As in adults, radiation; alkylating agents such as procarbazine, cyclophosphamide, chlorambucil, BCNU, and CCNU; and cisplatin are the most sterilizing and produce prolonged and sometimes permanent azoospermia.34,51 In addition, high doses of doxorubicin and cytosine arabinoside are additive with the aforementioned agents in producing gonadal damage.63 Regimens lacking alkylating agents, such as some used for acute lymphocytic leukemia, can even allow pubertal progression of spermatogenesis during treatment.64
Most chemotherapy regimens do not affect Leydig cell function, and hence, the timing of pubertal development and postpubertal testosterone levels are generally normal.65 However, busulfan plus cyclophosphamide, used in preparation for hematopoietic stem cell transplantation, appears to delay puberty in approximately 30% of boys.43
Extent of Gonadal Toxicity in Girls
Prepubertal girls appear to be less susceptible than young postpubertal women to cytotoxic therapy–induced ovarian failure (see Table 141.6). Furthermore, girls treated with radiation and alkylating agents after puberty have about a 10 times greater relative risk of developing early menopause versus those treated before puberty.66 Exposure of the ovaries to high-dose radiation, alkylating agents, and procarbazine are significant risk factors for acute ovarian failure in childhood cancer survivors.67 Radiation is the most damaging agent to the prepubertal ovary: 20 to 30 Gy induces permanent ovarian failure in nearly all girls.68 Doses of 8 to 15 Gy, usually with cyclophosphamide or melphalan in preparation for bone marrow transplantation, cause failure of ovarian function and pubertal development in 50% of girls,69 with younger girls being less sensitive than older ones.43 Radiation doses of up to 7 Gy do not cause ovarian failure or inhibit puberty62 but can have additive effects if chemotherapy is also given.
Most chemotherapy regimens do not cause loss of primordial follicles or failure of pubertal development and menarche. This includes high doses of cyclophosphamide of up to 48 g,70 various combination chemotherapy regimens used to treat leukemia, and the ABVD regimen for Hodgkin’s lymphoma.71 Even MOPP or nitrosourea chemotherapy does not affect pubertal and menstrual function development in 90% of girls.62,72 However, ChlVPP produces ovarian failure in 25% of girls73 and busulfan plus cyclophosphamide inhibits puberty in 50% of girls.43
Besides causing ovarian damage, irradiation for solid tumors in the abdomen (20 Gy or more for Wilms’ tumor) or in preparation for hematopoietic stem cell transplantation produces irreversible damage to uterine growth and blood flow in girls.74 This damage affects achievement and outcome of pregnancy later in life.75 If radiation doses are not too high (approximately 10 Gy, as in total body irradiation for transplantation), sex steroid replacement can improve uterine function.
GONADAL DYSFUNCTION AFTER CRANIAL IRRADIATION
Whereas gonadal irradiation causes primary hypogonadism, cranial irradiation is associated with secondary hypogonadism because of damage to the hypothalamus or the gonadotrophs in the pituitary, or both. In patients with pituitary or suprasellar lesions, gonadotropin deficiency is often present before antineoplastic therapy; however, patients with nasopharyngeal cancer or brain tumors have normal hypothalamic–pituitary–gonadal axes until they receive therapeutic irradiation with fields encompassing the hypothalamic–pituitary areas. In contrast, none of the cancer chemotherapeutic drugs directly impairs hypothalamic or anterior pituitary function.
In children, prophylactic cranial irradiation with 24 Gy for leukemia does not affect LH or FSH pulsatile secretion in the first 6 years,76 but it does produce subtle effects that appear after puberty (Table 141.8).77 However, in the shorter term, they may indirectly increase LH and FSH levels and cause precocious puberty in both boys and girls.78 Because irradiation also produces growth hormone deficiency, precocious puberty further exacerbates the risk of decreased adult stature. Treatment with a gonadotropin-releasing hormone (GnRH) analog to delay closure of the epiphyseal growth plates and, with growth hormone, can be given to avoid growth stunting.
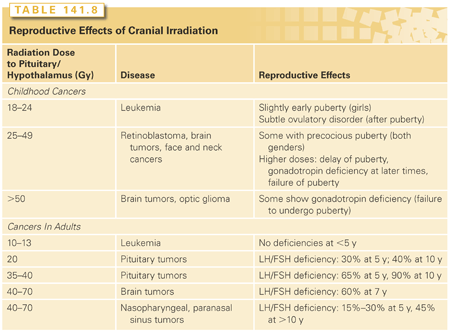
In adults, LH and FSH secretion decreases after cranial irradiation, and the decline becomes more severe with increasing treatment dose and time since treatment. This results in oligomenorrhea in women and low testosterone levels in men. Hyperprolactinemia might mediate the observed gonadal dysfunction in some cases.79
PRESERVATION OF FERTILITY, HORMONE LEVELS, AND SEXUAL FUNCTION
Choice of Regimens
It is sometimes possible to choose, among nearly equally curative regimens, one that minimizes the doses of the agents that are most sterilizing (see Tables 141.3 and 141.6). The use of ABVD instead of MOPP to treat Hodgkin’s lymphoma has dramatically reduced gonadal toxicity in a large group of patients.80 In the treatment of non-Hodgkin’s lymphoma, regimens that minimize the dose of cyclophosphamide, such as doxorubicin, cyclophosphamide, etoposide, vincristine, bleomycin, prednisone (VAPEC-B), produce gonadal failure less often in men than does CHOP with bleomycin.81
Gonadal Shielding and Oophoropexy
Except when the gonads must be irradiated because of actual or potential neoplastic involvement, they must be outside the field or shielded from the direct radiation beam. Nevertheless, an appreciable radiation dose from the accelerator head, collimator scatter, or internal lateral scatter may reach the gonads. Although moderately low radiation doses may be achieved, further reductions are desirable because of the possibilities of additive effects from chemotherapy and genetic damage to the sperm.
Gonadal dose depends on the distance from the field edge, field size, and photon energy. Pelvic radiation fields result in significant doses to the testis. Clamshell or other shields and beam blocking can reduce testicular doses two- to fivefold to 2 to 3 Gy for the inverted Y field used for Hodgkin’s lymphoma and to 0.2 to 0.8 Gy for the hemipelvic field used for seminoma.82
To reduce ovarian dose, an oophoropexy, in which the ovaries are surgically translocated away from the direct beam, can be performed. That region is further shielded using lead blocks. Some investigators report reductions in cumulative ovarian doses to 4 to 5 Gy during total nodal irradiation,62 which preserves fertility in younger women (see Table 141.6).56 There is convincing evidence that moving the ovaries outside of the radiation field is beneficial in preserving ovarian function and future fertility in an adult population.83,84 There is also evidence that this technique can preserve fertility in girls treated with radiation for Hodgkin’s lymphoma who were ultimately able to become pregnant.85 With advancement in the field of endoscopic surgery, these procedures can now be performed laparoscopically86,87 or robotically,88 allowing for a shortened hospital stay, earlier mobilization, and earlier return to normal activity.
Sperm Cryopreservation
Semen cryopreservation is an important procedure for preserving the fertility potential of men after cytotoxic treatment for cancer.89 The significance of notifying the patient of the potential risk of iatrogenic sterility as early as possible cannot be overemphasized.90 Physicians often are aware early during the diagnostic process that the patient will most likely need to receive potentially sterilizing cytotoxic therapy, although the exact diagnosis, stage, and treatment regimen may not have yet been decided. This time should be used to initiate and complete the cryopreservation procedure. The number of samples to be banked may depend on how much time is available before starting therapy, sperm quality, and the cost of storing samples. A collection of three or four samples with approximately 48-hour periods of abstinence between sampling (a total of more than 5 days) is ideal. With current assisted reproductive technologies, however, success can be achieved with fewer samples, even with poor-quality semen. It is strongly advisable to complete sperm banking before starting therapy to avoid increased genetic damage in sperm collected after the start of therapy.12 It is even possible to obtain semen with normal characteristics from 14- to 17-year-old boys by masturbation,91 penile vibratory stimulation, or electroejaculation.92 In addition, testicular sperm extraction is successful in 43% of pretreatment cancer patients who are azoospermic,93 and the sperm obtained in this way can be cryopreserved and used in intracytoplasmic sperm injection (ICSI) procedures.94 Testicular samples for sperm extraction can be obtained from orchiectomy or biopsy specimens that are routinely taken from testicular cancer patients if the surgeon is aware of the need and the processing procedures required for harvesting the sperm. Although a controversial topic, cryopreservation of immature testicular tissue in prepubertal boys with cancer may prove to be another suitable alternative, but the fear of reintroducing malignant cells through a graft is real, especially given the limitations of current technology.95
The success of in vitro fertilization (IVF) and ICSI makes cryopreservation of all samples containing any live sperm worthwhile. Even though 100% of oncologists in a study by Zapzalka et al.96 reported discussing fertility issues with their patients, Schover et al.89 found that only 60% of 900 male cancer patients surveyed who replied stated that they were informed about fertility issues and that only 51% stated they had been notified about sperm banking. According to Kohler et al.,97 only 36% of surveyed pediatric oncologists believe sperm banking is affordable, and the most likely reason to not offer it is poor survival prognosis.97 The cost of sperm banking three samples, including analysis and storage for 5 years, is between $1,200 (at a university medical school clinic) and $2,500 (at some private sperm banks). Intrauterine insemination is the least expensive assisted reproductive technology method (cost per cycle: $200 to $500, plus $1,200 for superovulation of the female partner, if necessary), but the sperm count and quality after thawing must be high, and conception rates are lower than with IVF. If sperm count or motility is impaired or sample amounts are limited, IVF is necessary. The average cost of IVF, including the medications used, is $15,000 per cycle.
Optimization of Fertility after Treatment
If sperm count recovers after cancer therapy, it usually reaches normospermic levels. However, some men remain oligospermic for many years. Although controlled studies have not been done, men who have recovered sperm production appear to have normal fertility, and their incidence of infertility does not appear to be any higher than the 15% rate among couples in the general population. Furthermore, cancer patients with recovered sperm counts ranging from just below 1 million/mL to 10 million/mL are able to successfully father children.19 Infertile patients with oligospermia should be managed in the same way as are those in the general population with male factor infertility, including the use of IVF with or without ICSI.98
Assisted Reproductive Technologies
Currently, the choices for preserving fertility in chemotherapy patients are limited (Table 141.9). Some are investigational, and all have demonstrated variable success. Fertility preservation can be attempted either with assisted reproductive technologies, gamete cryopreservation, or medical treatment. Assisted reproductive technology refers to in vitro handling of human oocytes and sperm or embryos for the purpose of establishing a pregnancy. Techniques for gamete preservation include sperm cryopreservation, oocyte cryopreservation, ovarian cortical cryopreservation, or whole ovary cryopreservation. Medical therapy includes treatment with hormonal agents such as oral contraceptive pills, progesterone, and GnRH analogs.99–112
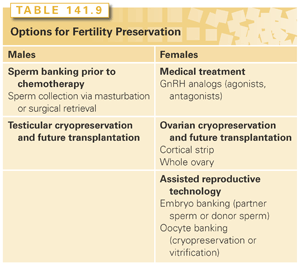
Assisted reproductive technology is still undergoing rapid advances and provides new options for cancer survivors with gonadal damage to achieve fertility. Previously, intrauterine insemination following cryopreserved sperm had been the only fertility preservation method available, and only 30% of men who had stored high-quality semen samples were able to achieve pregnancy.113
Currently, IVF with ICSI is the most successful method for achieving pregnancy from banked sperm. ICSI makes it possible to use a single spermatozoa to achieve oocyte fertilization through IVF. This technology has revolutionized the treatment of male infertility.114 ICSI is also often used with frozen banked sperm. IVF can be performed to create embryos that can be frozen and stored for later use. Pregnancy outcomes vary by IVF center but are excellent overall with frozen embryo transfers. The downside of IVF is that a single cycle can take 2 to 3 weeks to complete and necessitates a delay in cancer treatment.
Women with estrogen-responsive tumors can undergo ovulation induction with an aromatase inhibitor either as a solo agent or as an adjunct to standard stimulation protocols.115,116 To avoid the potential risks of rising estradiol levels during ovarian stimulation (sometimes 10 to 20 times levels seen in natural cycles), an increasing number of fertility specialists are using aromatase inhibitors (letrozole or tamoxifen) for ovarian stimulation.117 To date, the recurrence and survival rates of breast cancer patients undergoing such protocols were comparable with those of unstimulated control breast cancer patients.118
Clinical pregnancy rates of 30% to 50% per cycle and live birth rates of 25% to 50% can be expected at most fertility clinics. Cumulative live birth rates after four cycles have been reported between 60% to 80% in patients with male factor infertility, ovulatory dysfunction, endometriosis, and unexplained infertility.119 Although fresh sperm is preferred to frozen sperm in intrauterine insemination and IVF cycles, studies have shown excellent outcomes using sperm that was frozen and banked prior to cancer treatment.120 Although insemination with testicular sperm is an acceptable procedure, the use of nuclei from earlier stages (round spermatid nuclear injection) is not currently advisable.121 Overall, embryo banking is the most successful form of fertility preservation and should be offered to all women who are either in a serious relationship, married, or who are open to the idea of using donor sperm.
Oocyte banking either via cryopreservation (slow freezing) or vitrification (rapid freezing) is an option for single women who are not interested in using donor sperm. Similar to IVF, this entails ovarian hyperstimulation followed by surgical oocyte retrieval and, similar to IVF, also necessitates a delay in treatment ranging from 2 to 6 weeks.122 Mature oocyte cryopreservation and vitrification were recently changed from experimental treatments to options that can be offered in routine clinical care.123 There is no statistically significant difference in outcomes for fresh oocytes versus vitrified oocytes in oocyte donation programs.124 Although oocyte vitrification appears to be a good option for the female cancer patient without a partner, the technology to achieve comparable pregnancy rates is not available at all IVF centers and, thus, may not be available to all patients. Furthermore, when embryo cryopreservation is a viable option, it should be used instead of oocyte preservation because of the greater experience with that method.
A recent meta-analysis125 showed a 32% increase over baseline risk of birth defects in children born as a result of IVF/ICSI treatment, whereas another study126 found no significant difference after controlling for confounders in its IVF population but still found an increased risk in its ICSI population. These results should be interpreted with caution in terms of counseling patients for fertility preservation. First, assuming the baseline risk of birth defects is approximately 5%, even a 30% increase risk results in an absolute risk of only 6.5%. Second, subfertile couples, who are the majority undergoing IVF/ICSI, already carry an increased risk of birth defects, which would not apply to the fertility preservation patient.126 The increased risk of birth defects in ICSI treatment also has to be interpreted with caution in the setting of counseling the fertility preservation patient. There is an increased risk of approximately 3% for chromosomal abnormalities, mostly in the numbers of sex chromosomes, in offspring of ICSI procedures.127 Most of the chromosome abnormalities were not caused by ICSI, but were already present in the sperm of the infertile male parent. These abnormalities, which are common in cases of primary male infertility, should not be present in cryopreserved sperm from cancer patients.
Female Germ Cell Cryopreservation and Development
In addition to cryopreservation of oocytes, cryopreservation of ovarian tissue can also be undertaken (see Table 141.9). One method entails the surgical removal of ovarian tissue containing numerous primordial follicles, cryopreservation of tissue slices, and subsequent grafting back to the site of the ovary. This was initially successful in restoring ovarian endocrine function and fertility in sheep.128
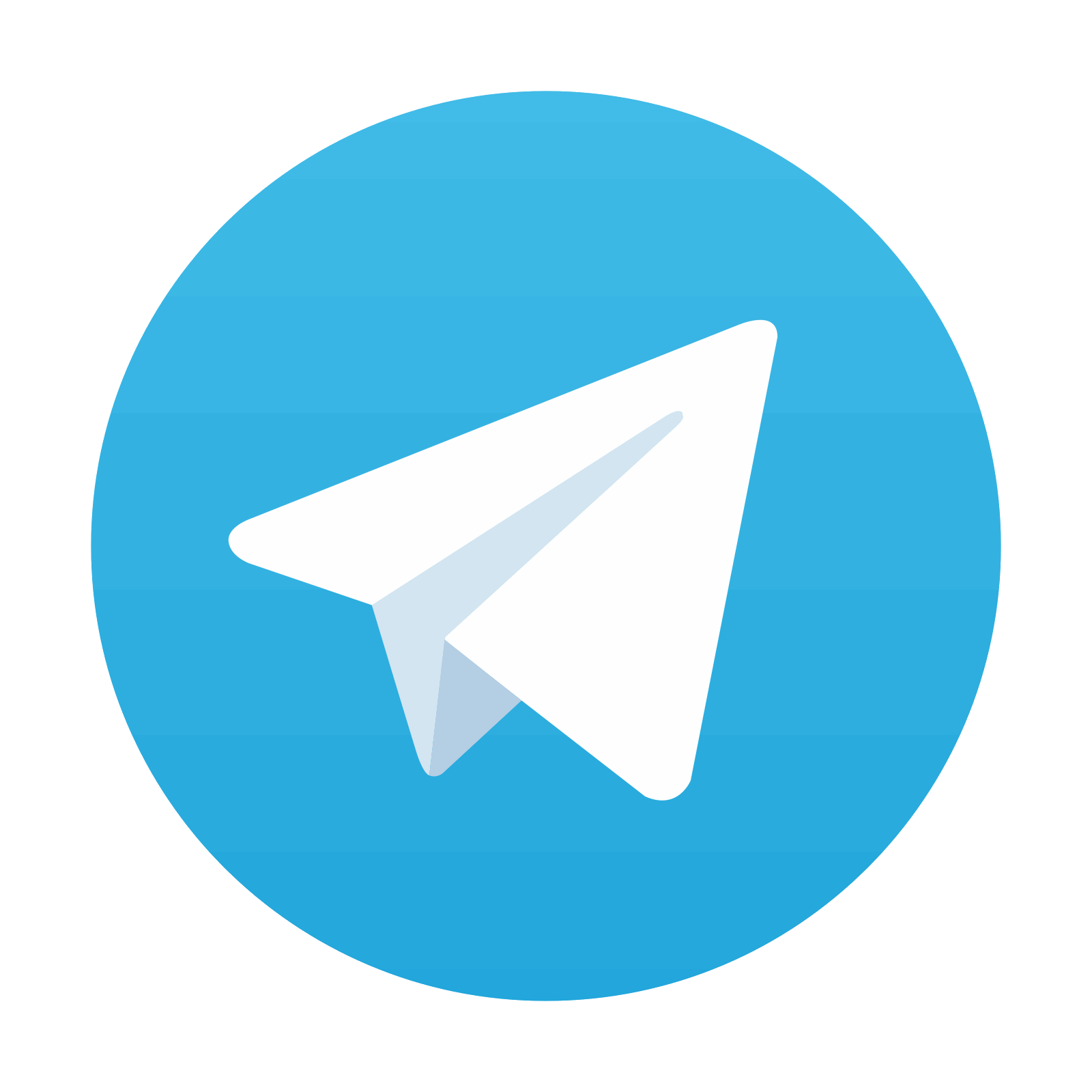
Stay updated, free articles. Join our Telegram channel

Full access? Get Clinical Tree
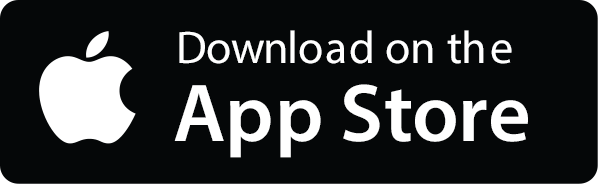
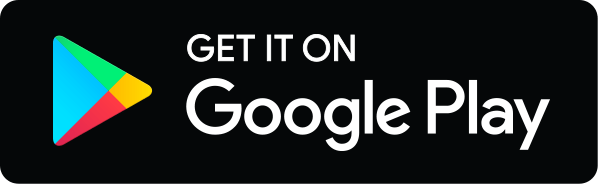