(1)
Medical Sciences Division Northern Ontario School of Medicine West Campus, Lakehead University, Thunder Bay, Ontario, Canada
Key Topics
Helicobacter pylori gastritis
Molecular pathology of gastric cancer (GasCa)
Screening for H. pylori infections
Circulating GasCa biomarkers
Circulating GasCa cells
Key Points
The 5-year survival rate is ~90 % when GasCa is confined to the mucosa but is markedly reduced when the tumor involves the muscularis propria. Biomarkers for early detection will therefore make an impact on patient survival.
An established etiologic agent of GasCa is Helicobacter pylori (H. pylori) infection, which accounts for the vast majority of intestinal-type GasCa. The molecular pathology of GasCa involves alterations in TP53, CTNNB1, HER2, RUNX3, and importantly CDH1, which is silenced via mutations or promoter methylation in almost all diffuse-type GasCas.
Circulating biomarkers, including targets of H. pylori, methylated genes, and circulating GasCa cells, have been in routine clinical practice or being pursued for development of noninvasive products for GasCa management.
6.1 Introduction
Gastric cancer is forth in incidence and the second leading cause of cancer-related deaths worldwide. The 2012 global incidence and mortality were 631,293 and 468,970, respectively. The 2016 data from the American Cancer Society estimate an incidence of 26,370 and mortality of 10,730 for the US. However, Asia-Pacific, Latin America, and Eastern Europe are some geographic regions with the highest incidence and prevalent rates in the world. For example, the age-standardized incident rates reveal high geographic regions such as Korea, Japan, and China with rates >20/100,000, intermediate regions including Singapore, Taiwan, and Malaysia with rates between 11 and 19/100,000, and low-risk areas such as Northern India, Thailand, Australia, and New Zealand with rates below 10/100,000. There are also pockets of relatively high incidences in the developed world. Los Angeles and Korean men with age-standardized incident rates of >40/100,000 and Alaskan natives with age-standardized incident rates of 36/100,000 are among the highest in the world. These figures imply the need for interventional measures to curtail mortalities from GasCa.
There are various risk factors that predispose an individual to developing GasCa, and these risks relate to the major subtypes of GasCa. Primarily genetic defects underlie the development of diffuse-type GasCa, while environmental and lifestyle factors such as diet and H. pylori gastritis are known risk factors of intestinal-type GasCa. Gastric cancer is a major cause of cancer-related deaths globally, partly because of late diagnosis. The 5-year survival is only 30–50 % for advanced stage disease even after curative-intent surgery with lymph node dissection. Local and distant recurrences are common, suggesting ineffective therapeutic targeting of residual disease. Early detection and effective treatment are key to improving the prognosis of GasCa. Because the outlook is good when diagnosed early, there are intensive screening programs in areas with high incidence and prevalence rates. Noninvasive, cost-effective early detection, and other companion diagnostic biomarkers will complement these efforts.
6.2 Screening Recommendations for GasCa
Cancer screening involves employing simple cost-effective tests across an entire healthy population defined to be at elevated risk, such that asymptomatic individuals with the disease or evidence of an impending disease can be identified and treated early. Screening programs are recommended only in situations where the prevalence of the disease is high enough to justify the effort and costs of screening. Thus, in view of the generally low incidence of GasCa in most Western societies, screening is not recommended. However, GasCa screening programs are in place in endemic areas such as Korea, Japan, Taiwan, and China. Currently, many screening programs involve the use of double contrast barium X-ray imaging, photofluorography, and endoscopy. Whereas these procedures are useful in detecting upper gastrointestinal pathology, they carry a risk of radiation exposure and are uncomfortable and expensive for population screening. Moreover, they detect established disease and thus are not generally useful in early detection strategies.
Similar to many cancers, early detection of GasCa is associated with decreased mortality and better quality of life following treatment. The 5-year survival rate for early localized cancer is over 90 %. Moreover, these early lesions are amenable to less invasive procedures such as endoscopic mucosal resection and/or submucosal dissection. While these procedures have equal benefits as gastrectomy, they offer much better quality of life. Late disease has dismal outcome with less than 50 % 5-year survival rate. Because the etiology and pathogenesis of gastric cancer are well established (especially for the intestinal type), other useful adjunct tests to the above procedures that will enable early detection include noninvasive screening for H. pylori infection. Other biomarkers to assess early cancer risk, such as biomarkers of field cancerization (e.g., methylation biomarkers), will be clinically relevant.
6.3 Helicobacter pylori and GasCa
H. pylori was first observed in 1979 by an Australian pathologist, Barry J. Marshall, and physician, J. Robin Warren. The organism was subsequently isolated in 1982, after Marshall infected himself, developed gastritis, and isolated the bacilli from his mucosa [1]. Deservingly, Marshall and Warren received the Nobel Prize for Physiology or Medicine in 2005 for their work.
H. pylori infection is strongly associated with intestinal-type non-cardia GasCa. The Centers for Disease Control and Prevention (CDC) estimates that two thirds of the global population is infected with H. pylori. The prevalence is estimated to be between 19 and 80 %. However, prevalence rates vary considerably in different geographic regions, reflecting the socioeconomic status of different communities. In general, infection rates are much higher in the developing parts of the world, with prevalence being up to 80 %, than in the developed world where rates are below 40 %. However, only ~1 % of all those infected develop GasCa. Thus, the high prevalent rates in Africa and Northern India are not directly correlated with high GasCa incidences. Other contributing factors facilitate H. pylori-mediated GasCa development. Noteworthy is the epidemiologic principle of agent-host-environment interactions:
The virulent factors from the agent, H. pylori bacilli, include polymorphisms in vacuolating cytotoxin A (VacA) and cytotoxin-associated gene A (CagA).
Host genetic factors identified include polymorphisms and expression of cytokines (IL-1β, TNFα, IL-10), mucins (MUC-1), and HLA genes.
Environmental interplay includes increased salt intake, which elevates the risk for GasCa because salt may be a mucosal irritant, while increased intake of fresh fruits and vegetables is protective due to their antioxidant effects.
Another infectious etiology of unknown mechanism in GasCa pathogenesis as yet is Epstein–Barr virus (EBV) infection. The virus is detected in 2–16 % of GasCas, with the majority being tumors from the proximal and middle portions of the stomach. Additionally, expression of EBV-related genes including EBER1, EBER2, EBNA1, LMP2A, BARF0, and BARF1 is found in GasCas.
6.4 Molecular Pathology of GasCa
The two major types of GasCa are the intestinal and diffuse types (Table 6.1). While H. pylori infection is associated with the intestinal type, diffuse-type GasCa often has a distinct genetic etiology. The mutagenic or epimutagenic effects of H. pylori gastritis are noteworthy in the molecular events that mediate gastric carcinogenesis.
Table 6.1
Comparison of intestinal and diffuse-type gastric adenocarcinomas
Features | Intestinal (differentiated) type | Diffuse (undifferentiated) type |
---|---|---|
Frequency | >85 % | ~15 % |
Age | Older | Younger |
Sex distribution | Males > females | Males = females |
Regional distribution | High-risk population (e.g., Japan) | Any population |
Risk factors | H. pylori infection, environment and host factors | Host genetic factors |
Region of stomach mostly involved | Non-cardia (antrum, lesser curvature) | Entire gastric epithelium can be affected |
Pathology | Ulcerative lesion | Cellular dispersion and thickening of gastric wall (“linitis plastica” or “leather bottle” appearance |
Mode of progression | Multistep carcinogenesis | None |
Prognosis | Good (early cancers) | Poor |
6.4.1 Classification of GasCa
Gastric cancer is classified based on tumor anatomic location, gross, and histologic appearance, as well as clinical features. Anatomically, there are distal and proximal cancers, but three types are recognized; type I (distal to esophagus), type II (cardia), and type III (stomach distal to cardia) (Fig. 6.1). Cancers originating from the cardiac region (proximal) and, which may involve the gastroesophageal junction (GEJ) pose a unique challenge as whether to be classified as gastric or esophageal cancers. Indeed, while the incidence of GasCa in general is on decline, GEJ tumors are on the rise. Histologic classification reveals extensive heterogeneity, while clinical classification as to early or advanced stage disease guides planned treatment protocols.
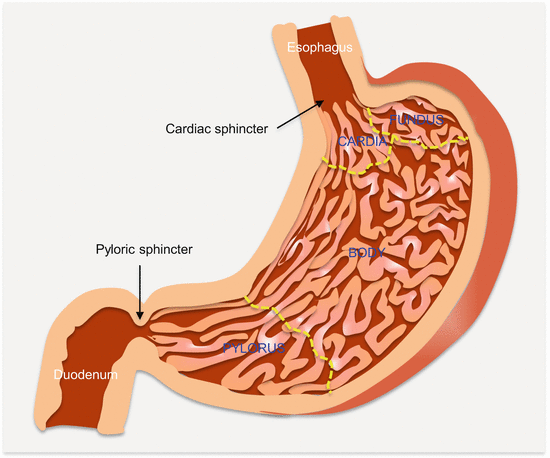
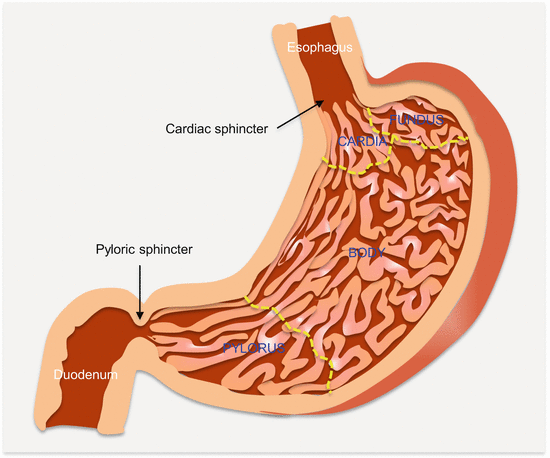
Fig. 6.1
Anatomic parts of the stomach
Clinically, GasCas confined to the mucosa and/or submucosa irrespective of lymph node involvement and tumor size are considered early stage disease. The 5-year survival for these patients is ~90 % compared to <60 % for those in whom the cancer has invaded the muscularis propria. According to Borrmann’s classification, advanced stage GasCa exhibit four types of gross appearances or growth patterns. They may be polypoid (type I), fungating (type II), ulcerating (type III), or infiltrating (type IV, also known as linitis plastica) in structure. But these cancers are very heterogeneous at the histologic level.
Histologically, GasCas exhibit architectural and histologic heterogeneity such that even different histologic elements can be found in the same tumor. Lauren in 1965 classified GasCas into three main categories: intestinal, which form the majority (54 % of GasCas), diffuse that is next in frequency (32 % of GasCas), and indeterminate (14 %) types. The intestinal type follows H. pylori gastritis and intestinal metaplasia, while the diffuse type has more of a genetic etiology. The WHO, however, identifies four histologic types based on the predominant histologic feature. These are tubular, papillary, mucinous, and signet ring cell (discohesive) carcinomas. The WHO also recognizes uncommon histologic types as mixed carcinomas. In context with Lauren’s classification, the papillary, tubular, and mucinous tumors belong to the intestinal type, while the poorly cohesive (signet ring cell) carcinomas belong to Lauren diffuse type. Finally, the numerous rare WHO histologic types (mixed carcinomas) are the indeterminate tumors (Table 6.2).
Table 6.2
Classification of GasCa
Lauren (1965) | WHO (2010) |
---|---|
Intestinal type | Tubular adenocarcinoma Papillary adenocarcinoma Mucinous adenocarcinoma |
Diffuse type | Signet ring cell carcinoma Other less cohesive types |
Indeterminate | ~16 different histologic subtypes |
Tubular adenocarcinomas histologically appear as tubules with intraluminal accumulation of mucin and inflammatory infiltrates. The tubules, may have irregularly distended, fused, or branching architecture. Papillary adenomas are often from the proximal stomach and tend to occur in older people. They also tend to be aggressive with early metastasis to the liver and lymph nodes. Histologically, papillary adenomas form epithelial projections with central fibrovascular tissue. Extensive extracellular mucin (>50 % of tumor) characterizes mucinous adenomas, which are less common than the first two. The cancer cells are either irregularly clustered or form abortive glands. Isolated signet ring cells may be found in these tumors. Signet ring cell carcinomas are usually composed of signet ring cells or other cell types (non-signet ring cell mixture). The non-signet ring cell, but poorly cohesive carcinomas are composed of histiocytes, lymphocytes, and plasma cells.
6.4.2 Genetic Alterations in GasCa
Gastric cancers are mostly sporadic; however, there are evidences of some familial clustering, especially with known cancer-associated syndromes such as familial adenomatous polyposis, Lynch (Peutz–Jeghers), juvenile polyposis, Li–Fraumeni, and hereditary breast–ovarian cancer syndromes, which account for about 1–3 % of all GasCas. As a genetic disease, 10–44 % of GasCas have MSI due to somatic loss of MLH1. In addition to established genes such as CDH1, HER2, TP53, CTNNB1 and RUNX3, alterations of several other genes relevant to the pathogenesis of sporadic GasCa include BAX, CDKN2A, IGFR2, CDX2, APC, and TGFβR2. Modifier genes that promote GasCa development in H. pylori infections include genetic variations of pro-inflammatory genes such as IL1b, IL-8, IL-10, TLR4, and TNF.
6.4.2.1 CDH1 Alterations in GasCa
Hereditary GasCa is an autosomal dominant disease with about 30 % of these patients having germline CDH1 (E-cadherin) mutations and deletions. It has a high penetrance such that mutation carriers have over 80 % lifetime risk of developing GasCa. Hence, prophylactic gastrectomy is recommended as a preventive measure for mutation carriers. Because the risk of developing invasive ductal carcinoma of the breast is equally high among women with these mutations, breast cancer screening by mammography is offered beginning at age 35. Mutations in CDH1 also underlie half of sporadic diffuse-type GasCas, with methylation and reduced expression noted in the remaining 50 %. Thus, almost all sporadic diffuse-type GasCas appear to originate on the background of CDH1 silencing.
6.4.2.2 HER2 Alterations in GasCa
Amplification and overexpression of HER2 is demonstrated in 12–35 % of GasCas. The prevalence is higher (~35 %) in intestinal-type GasCas originating from the proximal stomach and GEJ. All GasCas are tested for HER2 status, because of the evidence of response to anti-HER2 antibody-targeted therapy and associated good prognosis. The ToGA phase III international trial of combined trastuzumab and chemotherapy revealed a prolonged OS and PFS, suggestive of the effectiveness of trastuzumab in HER2-positive GasCas [2].
6.4.2.3 CTNNB1 Alterations in GasCa
Mutations in CTNNB1, APC, and AXIN1 associated with aberrant WNT/β-catenin pathway activation underlie the pathogenesis of some GasCas. About ~27 % of GasCas harbor CTNNB1 mutations. Mutations in CTNNB1, especially at the APC control regions, make it refractory to regulation by APC, thus leading to β-catenin accumulation, which in complex with TCF in the nucleus induces the transcription of genes such as CCND1 and MYC.
6.4.2.4 TP53 Alterations in GasCa
TP53 mutations and loss of tumor suppressor functions are common in sporadic intestinal and diffuse-type GasCas. These mutations are early events in cancer development because ~50 % of H. pylori gastritis harbors them. Similarly, ~38 % and 58 % of intestinal metaplasia and dysplastic lesions, respectively, have TP53 mutations. TP53 LOH occurs in up to 60 %, and mutations in as many as 77 % of GasCas. While the mutational spectrum is diverse, commonly mutated regions are in codons 175, 213, 245, 248, 273, and 285. Of interest, the mutations mostly involve G:C to A:T transitions at CpG regions. The mutations are more frequent in proximal tumors and in younger patients. They are also more common in advanced than early stage tumors.
6.4.2.5 RUNX3 Alterations in GasCa
The tumor suppressor gene, runt-related transcription factor 3 (RUNX3), is downstream of the TGFβ signaling pathway. Activated RUNX3 induces the expression of genes such as CDKN1A that represses cellular proliferation and proapoptotic BCL2L11 (BIM). Additionally, RUNX3 inhibits tumor metastasis and angiogenesis by decreasing VEGFA expression. RUNX3 loss of function via promoter hypermethylation and deletions occur in as many as 45–60 % of GasCas. These alterations are also early in GasCa development because they are present in H. pylori-infected gastric mucosa, intestinal metaplasia, and gastric adenomas.
6.5 Screening for H. Pylori Infection
H. pylori is a spiral gram-negative microaerophilic flagellate bacillus. Warren and Marshall discovered its carcinogenic effects accidentally in 1982. H. pylori is a gastric carcinogen, classified by the WHO as a group I carcinogen. It is the major etiologic agent of GasCa. H. pylori infection causes chronic gastritis that can lead to peptic ulceration, atrophic gastritis, intestinal metaplasia, and eventually GasCa. It has been established based on randomized control clinical trial evidences that H. pylori eradication significantly reduces the risk of individuals developing GasCa. Therefore in high-risk populations, screening for and treating H. pylori infection (though there are issues with this approach such as emergence of resistance strains) is a prudent recommendation. Screening programs currently target adults beginning a decade or two ahead of the period of known risk for individuals developing GasCa within the population. Children are exempt from screening programs because of the high rate of reinfection in endemic areas.
Infection with H. pylori usually leads to the production of H. pylori-specific immunoglobulins (e.g., IgG and IgA) in a vast majority of patients. Additionally, some infected people have H. pylori proteins such as VacA and CagA in circulation. These antibodies and proteins are targets used to develop H. pylori serologic tests. There are a couple of issues with the blood serologic assays. First, because of the high background prevalence of infection and hence serologic markers in endemic populations, it is important to develop locally validated serologic assay for different populations. Second, the test is unsuitable as a routine test and is not recommended for evaluating treatment effectiveness, because a positive test result does not differentiate between current and past infection (antibodies may persist for 1–2 years.). The diagnosis of H. pylori infection is traditionally by endoscopic visualization and sampling of gastric mucosa for histologic examination, microbiologic culture, and urease testing (urease positive). However, three noninvasive tests permit cost-effective population-based screening. These are the gold standard urea breath test and the serologic and stool antigen tests (Table 6.3).
Table 6.3
Screening for early detection of GasCa
Features | 13C-UBT | 14C-UBT | Serology | HpSAg | Endoscopy |
---|---|---|---|---|---|
Sample | Exhaled breath | Exhaled breath | Blood/serum | Stool | Tissue |
Sample acquisition | Noninvasive | Noninvasive | Minimally invasive | Noninvasive | Invasive |
Quick to process | No | No | Yes | Yes | No |
Cost | Moderate | Moderate | Low cost | Low cost | Expensive |
Radiation exposure | No | Yes | No | No | No |
Test utility | Detection/assessment of eradication | Detection/assessment of eradication | Detection | Detection/assessment of eradication | Detection/assessment of eradication |
Sensitivity | Up to 98 % | – | Up to 95 % | Up to 95 % | Up to 95 % |
Specificity | Up to 98 % | – | Up to 95 % | Up to 100 % | Up to 95 % |
Overall performance | Gold standard | Gold Standard | Suboptimal | Suboptimal | Sampling errors due to patchy infections |
6.5.1 Urea Breath Test
This is the gold standard of H. pylori testing because it performs much better than all other available tests, including endoscopy, which misses some infections because of the patchy nature of gastric mucosal sampling. The test is used for detection and hence eradication of H. pylori infection. Though not globally available, it is the best noninvasive test for H. pylori in several endemic communities.
The test, which was initially developed simultaneously by Graham et al. and Marshall and Surveyor, is based on the principle of breath tests used to detect malabsorption [3, 4]. In the case of H. pylori testing, it is based on the ability of the organism to produce an active and specific urease in the gut. The test involves an ingestion of a standard 75 mg of carbon isotope-labeled urea after an overnight fast. In the stomach, the urea is broken down by H. pylori urease enzyme into carbon dioxide (CO2) and ammonia. The resulting isotope-labeled 13CO2 diffuses into the blood stream and is exhaled through the breath. The level of isotope exhaled, which indicates H. pylori activity in the stomach, is detected and measured by mass or infrared spectroscopy.
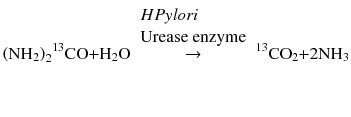
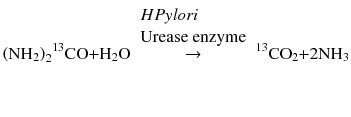
Measurement is done before (baseline) and up to 60 min after urea ingestion. The result is computed as Δ13C/12CO2 ratio (δ), which is a measure of isotope 13C to non-isotope 12CO2. Cutoff levels may be dependent on other factors, such as age, but in general are considered positive when above 2–5 %. 13C is a natural isotope, and therefore samples can be collected anywhere such as in the physician’s office and mailed to the laboratory for analysis. However, 14C is a radioactive isotope requiring that testing be done in a controlled nuclear medicine facility. Also because of the long half-life of 14C (5.730 years.), its use is restricted by environmental protection policies.
6.5.2 Stool Antigen Test
The only known niche for H. pylori in the gastrointestinal tract is the stomach and duodenum. It is adapted to the acidic environment through the production of urease enzyme that enables the production of ammonia to neutralize the acid. Because of this only known habitat, detection of H. pylori antigen in gastrointestinal samples reflects the presence H. pylori gastric mucosal infection. The stool antigen test is a direct assay targeting H. pylori-specific antigens in stool. Following affinity-purified whole cell lysates from stool, monoclonal, or polyclonal antibodies are used to detect the presence or absence of H. pylori in the sample. The procedure can be done with ELISA, which takes about 2 h to complete, or rapid lateral flow chromatography that takes just 10–30 min. The lateral flow method is conducive for small community laboratories that handle just a few samples at a time.
6.5.3 Serum Pepsinogen Test
Serum pepsinogen (PG) levels are influenced by functional and morphological gastric mucosal changes reminiscent of intestinal-type GasCa progression from H. pylori infection, gastritis, atrophy, and then to GasCa. Measurement of serum PG as a screening assay for GasCa risk and hence the need for more invasive and expensive procedures have been recommended and been in clinical practice in some endemic areas such as Japan, Korea, and Matsu (an island between Taiwan and mainland China).
There are two biochemically and immunologically distinct PG isoforms produced by gastric mucosal glands: PGI (or PGA) and PGII (PGC). Fundic glands, chief and mucous neck cells produce both types. Additionally, cells of pyloric, cardiac, and duodenal Brunner’s glands secrete PGII (Fig. 6.1). Therefore, gastritis, atrophic, and metaplastic changes in fundic glands lead to decrease serum PGI, while PGII levels remain close to normal because of compensatory production by cells of pyloric and cardiac regions, as well as duodenal Brunner’s glands. These changes cause a decrease in the ratio of PGI/PGII. Thus, progressive decreases in this ratio strongly correlate with progressive mucosal changes from normal mucosa to extensive gastritis and gastric atrophy with shrinking numbers of fundic glands.
Complex dynamic changes in PGI/PGII ratio became evident with the discovery of H. pylori and its effects on gastric mucosa. H. pylori directly and indirectly stimulates PG secretion. Directly, sonicate and lipopolysaccharide from H. pylori and indirectly gastric inflammation caused by H. pylori stimulate PG release. The effect of H. pylori on PG secretion is more pronounced on PGII levels. Thus, infection leads to very high levels of PGII, and hence the ratio of PGI/PGII still remains low, but at a different level from noninfected gastric mucosa. Similarly, with H. pylori eradication, PGII levels decrease more than PGI, and thus elevating the PGI/PGII ratio. This has been one source of controversy on the usefulness of the PG test.
H. pylori atrophic gastritis migrates from the antrum proximally toward the cardia, with a progressive reduction in fundic glands. Initially, this progressive mode is associated with more PGII than PGI secretion and hence a reduced PGI/PGII ratio. As disease progresses to atrophy, chief cells are replaced by pyloric glands (metaplasia) leading to much decreases in PGI, but PGII levels remain high; hence, the PGI/PGII ratio is much reduced indicating gastric atrophy and metaplasia. Aside from H. pylori status, factors that affect PG release by gastric mucosal cells are age, gender, and histopathologic type of cancer, as well as the distribution and stage of cancer. Different test kits and cutoff values also hinder standardization of the test. However, evidence-based medicine indicates a clear value of PG test as adjunct to imaging-based GasCa screening in endemic areas, especially for the early detection of the intestinal type.
6.5.4 The Combine Serum H. pylori and PG Tests
This test, also referred to as the ABC (gastritis A, B, C, D) method, is a GasCa risk stratification-screening assay used in Japan. Because no single screening test performs optimally, Dr. Kazumasa Miki [5] developed the combined H. pylori IgG antibody and serum PG level assay. For example, the PG test alone is unable to pick up diffuse-type GasCa, which still develops in up to 40 % of patients with negative PG test. The ABC test combines serologic assay for anti-Hp IgG antibody and serum PG levels to stratify individuals into four groups of gastritis or gastric mucosal changes:
Group A individuals are Hp(−)PG(−), and these individuals have no infection and therefore have normal healthy gastric mucosa. They are not recommended for any further evaluation.
People in Group B are Hp(+)PG(−). They have mild or no chronic active gastritis (CAG) and only have elevated risk for peptic ulcer. It is recommended that they undergo upper gastrointestinal endoscopy every 3 years.
Group C individuals are both positive for Hp and PG, i.e., Hp(+)PG(+). They have evidence of CAG and at elevated risk for developing gastric adenoma, hyperplastic polyps, and cancer. Hence they require endoscopy every 2 years.
Finally Group D individuals are Hp(−)PG(+). They demonstrate the presence of severe CAG, and advanced atrophy, and are therefore at high risk for developing GasCa, especially the intestinal type. Annual endoscopy is recommended for this group.
While, much of the evidence for the diagnostic utility of these biomarkers is from Asian population, some evidence also indicates their possible generalizability to other populations as well. A longitudinal nested case control study of 52 GasCa patients and 104 controls from the Health, Alcohol and Psychosocial Factors In Eastern Europe (HAPIEE) study assessed the predictive value of PGI, PGII, G-17, and IgG antibodies to H. pylori (HP-Ab) (named the GastroPanel) for GasCa detection in the Caucasian population. Below baseline cutoff values of all the biomarkers were useful predictors of GasCa, with diagnostic performance ORs of 2.9 for PGI, 9.0 for PGII, 3.3 for PGI to PGII ratio, 1.8 for G-17, and 0.4 for HP-Ab. In multivariate analyses however, PGI/PGII ratio was the strongest independent predictor of GasCa with OR of 2.9 [6].
6.6 Circulating GasCa Biomarkers
Noninvasive biomarkers have been explored in the circulation for GasCa management. These are desirable to complement the current screening efforts for H. pylori gastritis in early cancer detection. Thus, being extensively investigated are alterations in the epigenome, noncoding RNA, proteins, and peptides, as well as circulating gastric cancer cells.
6.6.1 Circulating Cell-Free Nucleic Acid Content as GasCa Biomarkers
While primarily focused on the detection of ctDNA through tumor-associated mutations or methylations, a few studies have addressed ccfDNA content variations between GasCa patients and healthy people and their clinical relevance [7–9]. The findings have been consistent with those of other tumors. The levels of ccfDNA are much significantly higher in GasCa patients than controls. As a diagnostic biomarker, the performance of ccfDNA content has been impressive, with AUROCC of 0.75–0.991 [7, 9]. Although elevated ccfDNA is not GasCa specific, these findings demonstrate the facile detection of GasCa DNA in circulation that can be exploited for various clinical applications.
6.6.2 Circulating GasCa Epigenetic Biomarkers
Epigenetic changes in circulation have been explored for their noninvasive diagnostic and prognostic utility in GasCa patients. Indeed, genes commonly methylated in GasCa tissue samples (some of which demonstrate field cancerization) are measurable in circulation of GasCa patients. Their value in early cancer detection deserves further investigation.
6.6.2.1 Circulating GasCa Diagnostic Epigenetic Biomarkers
To test DNA methylation as an early detection biomarker, preoperative serum samples from 106 GasCa patients were subjected to methylation analysis of CDKN2A and CDH1. Methylation in one or both genes was present in 37 % of the samples including 28 % from early stage cancer patients, suggesting a potential utility in early detection [10]. Subsequent study by this group attempted to increase the sensitivity of the previous assay by incorporating methylation of RARβ. The sensitivity was increased modestly from 37 to 48 %. In a follow-up analysis, hypermethylation was demonstrated in three cases with recurrent diseases [11]. Methylation in paired tumor and sera of DAPK, CDH1, GSTP1, CDKN2B, and CDKN2A were studied in GasCa patients. Hypermethylation of all five genes were frequent in GasCa and matched sera. Over 60 % of serum samples from cancer patients harbored epigenetic alterations, suggesting their utility as screening biomarkers [12]. Methylation of CDKN2A promoter in tumor and paired sera from GasCa patients revealed similar methylation frequencies, occurring at a rate of 38 % in primary GasCa tissues and at 26 % in plasma samples. This methylation status, as expected, was very specific to cancer [13]. Preoperative GasCa patient serum samples showed promoter hypermethylation of at least CDKN2A, CDH1, or RARβ in 44 % of cases, including early stage disease patients. Methylation was significantly associated with venous invasion as well [14]. In another series, preoperative serum samples from patients were subjected to methylation analysis of CDKN2A, CDH1, and RARβ, and the results were compared to levels of conventional serum markers (CEA and CA19-9). Hypermethylation was demonstrated in 57 % of patients that showed elevated CEA or CA19-9 levels. No correlation was found between methylation status and elevated serum CEA or CA19-9 levels, indicating complementary use could increase sensitivity for GasCa detection [15]. ATP4A and ATP4B are downregulated in GasCa, and this is possibly due to intragenic exon 7 (ATP4A) and exon 1 (ATP4B), but not promoter, hypermethylation. These methylation patterns were detected in ccfDNA in patient plasma samples as well, and are potential GasCa biomarkers [16].
6.6.2.2 Circulating GasCa Prognostic Epigenetic Biomarkers
The prognostic potential of methylated CDKN2A, CDH1, and RARβ in preoperative serum from GasCa patients receiving curative-intent gastrectomy was evaluated. Hypermethylation of at least one gene was demonstrated in 48 % of patients, but only CDH1 had prognostic association [17]. Ling et al. evaluated the diagnostic, prognostic, and recurrence prediction value of methylation of XAF1 in samples from GasCa patients [18]. Methylation and expression of XAF1 was assayed in 202 GasCa patient tumor tissues, normal adjacent tissues, and paired sera. The downregulation of XAF1 in GasCa was confirmed, and promoter methylation was demonstrable in as many as 83.2 % of tumor tissues and 27.2 % of normal adjacent tissues (indicative of field cancerization in gastric mucosa), but was completely absent in 88 normal gastric tissue control samples. Similarly, XAF1 methylation was detected in as many as 69.8 % of patient sera. These methylation patterns performed very well as diagnostic biomarkers, with AUROCC of 0.85 for tissue samples and 0.91 for the serum samples. They also significantly correlated with poor prognosis and predicted recurrences after surgery. RUNX3 methylation in preoperative and postoperative sera from patients with GasCa revealed a rate of 29 % in preoperative samples, and these levels decreased after surgery. RUNX3 methylation index (defined as the ratio of amounts of methylated RUNX3 to ACTB) was associated with cancer stage, histology, and lymphatic and vascular invasion [19].
A meta-analysis of DNA methylation in GasCa samples, including whole blood, serum, and plasma, uncovered 77 relevant genes that showed significant differential methylation between cancer and normal samples. The methylation of four genes was detectable in plasma, and seven in serum samples (promoter hypermethylation of CDKN2B was present in both media). The general findings were that DNA methylation of 18 genes had prognostic significance, while methylation of CDKN2A was significant in predicting outcomes in patients on chemotherapy. Additionally, gene methylation, especially CDKN2A, DAPK, CDH1, and CHFR, demonstrated field effect in gastric mucosa. The methylation of these genes was significantly different between normal gastric tissues from GasCa patients and those from people without cancer. This meta-analytical study identified the following genes, DAPK, CDH1, CDKN2A, CDKN2B, SULF1, RPRM, RNF180, SOCS1, SFRP2, and MGMT, to show significant differential methylation in circulation of GasCa patients compared to controls [20]. Multiple studies suggest the diagnostic and prognostic potential of methylated genes in GasCa patients.
6.6.3 Circulating GasCa Genetic Biomarkers
The role of serum MSA in GIST was questioned using LOH at sites of 12 polymorphic markers on five chromosomes. LOH in preoperative serum was detected in 65.4 % of GIST patients but in only one control sample, giving a diagnostic specificity of 87.7 %. This serum LOH strongly correlated with Fletcher risk group. Metastasis was associated with preoperative LOH of two or more markers. The sensitivity and specificity for prediction of relapse were 75 % and 64.1 %, respectively. Serum LOH significantly predicted OS [21]. In a follow-up study by this group, the 12 polymorphic markers were used to determine LOH in ccfDNA, which was detected in 32.6 % of patient samples. An LOH score of two or more markers was observed in 58 % of patients with recurrent disease on CT imaging scans, and this significantly contrasted with only 25 % in those with no clinical evidence of recurrence. However, LOH had no prognostic association after a median 48-month follow-up [22]. The relationship of various clinicopathologic factors to mutated TP53 in primary tumor tissue and in circulation, as well as circulating p53 antibodies in GasCa patients, was examined. Circulating mutant TP53 detection was related to progressive tumor depth, and patients positive for mutant TP53 in both tumor and blood, as well as circulating p53 antibodies, had significantly poorer prognosis [23].
6.6.4 Circulating GasCa Noncoding RNA Biomarkers
Noncoding RNAs, primarily miRNAs and lncRNAs, have been extensively investigated in circulation of GasCa patients. GasCa is characterized by deregulated miRNA expression. Thus, several studies have pursued their differential circulating levels in patients compared to healthy control subjects. Table 6.4 summarizes circulating GasCa miRNAs. MiR-17-92 (miR-17, miR-18, miR-19a, miR-20a, and miR-92) and miR-106-363 clusters are strongly associated with GasCa. Moreover, miR-17, miR-20a, miR-106a, and miR-106b have high sequence homology and therefore may regulate identical genes. These miRNAs have thus shown redundant and overlapping roles in GasCa. A large number of GasCa miRNAs show consistent levels with their tissue expression, and such miRNAs may be passively released or nonselectively packaged in vesicles. However, some miRNAs show discordant levels to their tissue expression. These miRNAs are likely packaged in a selective fashion into extracellular vesicles or actively secreted. Irrespective of the mechanism of release, circulating miRNAs have shown better diagnostic performances than traditional GasCa serum biomarkers.
Table 6.4
Circulating GasCa miRNAs
Increased | Decreased |
---|---|
let-7e, miR-1, miR-17, miR-17-5p, miR-20a, miR-21, miR-27a, miR-27b, miR-34a, miR-106a, miR-106b, miR-175-5p, miR-187, miR-191, miR-196a, miR-199a-3p, miR-200c, miR-221, miR-222, miR-223, miR-335, miR-370, miR-371-5p, miR-376c, miR-378, miR-421, miR-423-5p, miR-451, miR-457, miR-486, miR-744 | let-7a, miR-195-5p, miR-218, miR-375 |
6.6.4.1 Circulating GasCa Diagnostic MiRNA Biomarkers
A number of studies have demonstrated the diagnostic potential of circulating miRNAs in GasCa. They have proven to be early detection biomarkers and shown the ability to differentiate between proximal vs. distal cancers.
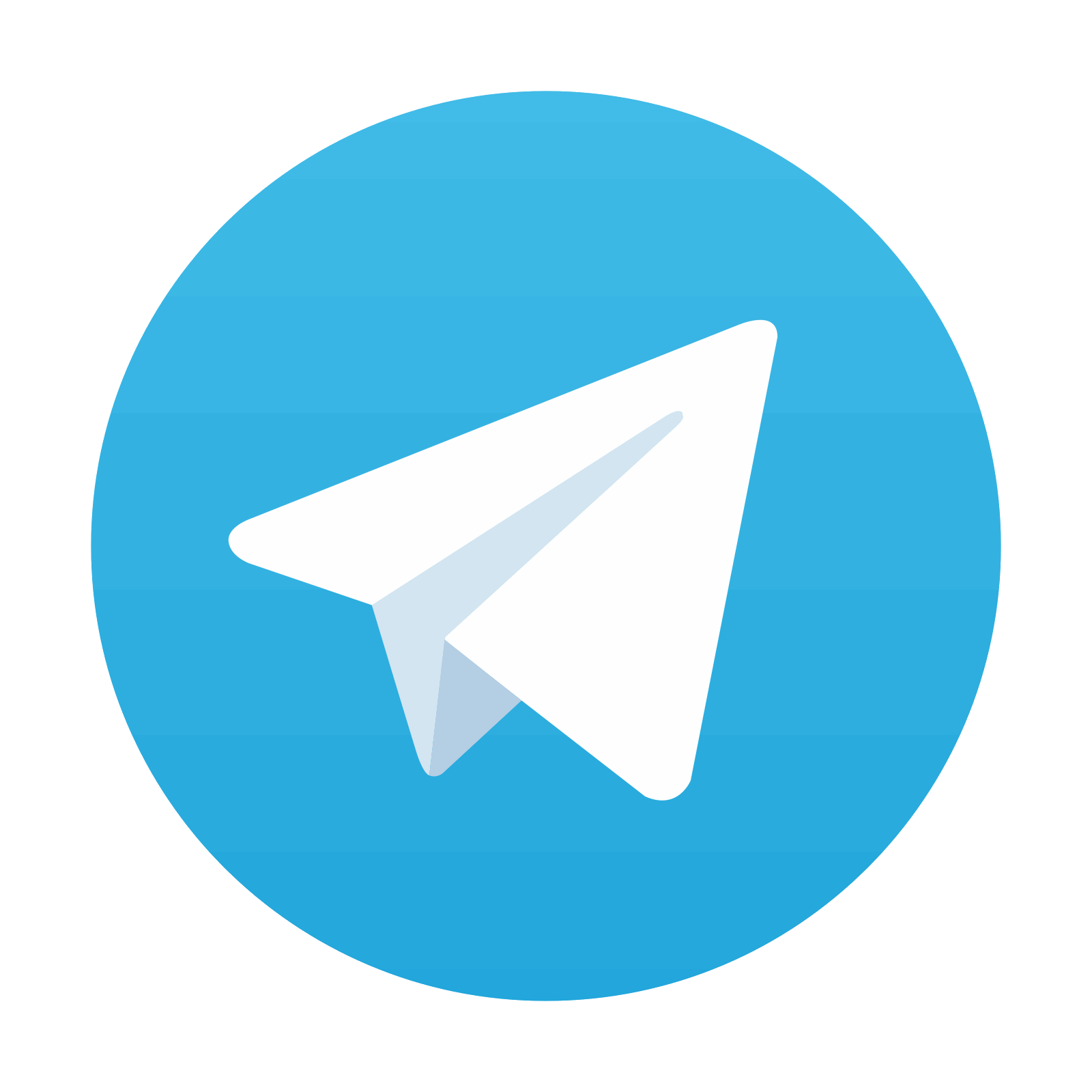
Stay updated, free articles. Join our Telegram channel

Full access? Get Clinical Tree
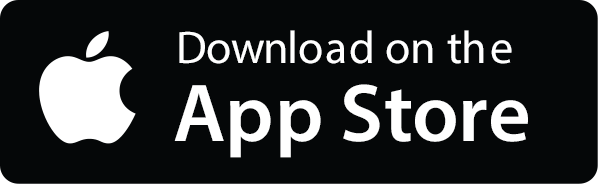
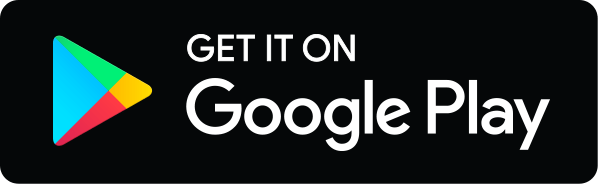
