Exercise and Pituitary—Testicular Function
Normal Men—Exercise Responses
Testosterone
It is generally accepted that short-term, maximal, and anaerobic-type exercise results in an elevation of the circulating testosterone level (see Table 13.1 for definitions of exercise-types terminology) [28, 32–34]. It is still an issue of some debate whether this effect is due solely to hemoconcentration and reduced metabolic clearance, or whether there is also an increase in testosterone production [6, 27, 35].
Table 13.1
Categorization and definition of exercise intensive and exercise-type relative to exercise studies of physically fit men
Category term | Relative intensity (%VO2max) | Energy pathway predominating | Typical duration | Other terminology |
---|---|---|---|---|
Light or easy exercise | <35 | Aerobic | >30 min | Submaximal |
Moderate exercise | >35 < 70 | Aerobic | >30 min < 180 min | Submaximal |
Heavy exercise | >70 | Aerobic—anaerobic | <120 min | Submaximal |
Maximal exercise | 100 | Aerobic—anaerobic | <15 min | Maximal or max |
Supra-maximal exercise | >100 | Anaerobic | <1 min | Sprints, power |
Unlike changes during maximal exercise, testosterone responses to submaximal exercise are more variable, and seem dependent on the duration and intensity of exercise. Progressive increases in testosterone levels during moderate intensity exercise lasting 45–90 min have been found [28, 36, 37]. However, 90 min of submaximal moderate intensity exercise has also been reported to produce no change or a slight decrease in testosterone concentrations [28, 36]. Exercise of a moderate or hard intensity until exhaustion of greater than ~2 h duration typically lowers testosterone concentrations [28, 36, 37].
Several explanations for these dissimilar changes during submaximal exercise have been proposed. Initially, hemoconcentration may increase the testosterone concentration; but as exercise continues, testicular testosterone production declines. The latter change may be due partly to reduced testicular blood flow [29, 30]. Hepatic blood flow may also decline reducing hepatic clearance [29, 30]. This latter change may be offset by an increased uptake of testosterone by peripheral target tissue (i.e., skeletal muscle) as exercise duration continues, gradually reducing circulating testosterone levels.
Gonadotropins
Research results examining the gonadotropin response to maximal and anaerobic-type exercise are somewhat contradictory [35, 41, 42]; however, the majority of the evidence indicates there are small transient increases in the circulating gonadotropins [40, 43–49]. In addition, the outcomes of these studies, and their interpretations, are subject to methodological limitations. In many cases, researchers did not obtain blood samples frequently enough to assess the highly pulsatile gonadotropin secretory profile [12, 19, 27]. Resistance-type exercise induces small increases in serum gonadotropin levels in both men and women [46, 49–51].
Likewise, the prolonged (~1–2 h) submaximal exercise responses of the gonadotropins vary considerably for both men and women [28, 46, 47]. Evidence of significant increase, decrease, and no change has been reported [36, 40, 43, 44, 46, 47]. If submaximal exercise is extended for extremely long periods, or until exhaustion occurs, gonadotropin levels are suppressed [46, 51–54].
These acute exercise-induced gonadotropin disturbances are transient and last for relatively short periods into the recovery from exercise [35]. The exception to this point is that submaximal exercise of many hours duration can produce disturbances that last several days [53, 54]. Interestingly, scant research exist on the influence of exercise on the pulsatile release of the gonadotropins in men, and these findings are inconclusive [36, 37, 41, 43, 44].
Other Pituitary Hormones—Prolactin
PRL presents an interesting paradox in the reproductive physiology of exercise. At physiological concentrations, PRL may be necessary for normal testicular function, while high PRL levels disrupt both central and peripheral aspects of the hypothalamic-pituitary-testicular axis [19, 55]. Circulating PRL concentrations increase during most exercise. The magnitude of the increase appears approximately proportional to the intensity of the activity [28, 46, 56]. Short-term, maximal, and anaerobic-type exercise increases PRL levels [28, 46, 56–60]. Submaximal exercise of 30 to 60 min duration, provided it is intense enough, increases circulating PRL levels as well [28, 47, 57–60]. Extending the duration of a submaximal exercise bout augments the magnitude of the PRL response [27, 47, 56, 57].
There are several factors that further increase the PRL response to prolonged exercise: (a) consumption of a diet rich in fat, (b) fasting for an extended period of time prior to exercise, (c) performing exercise in conditions promoting an increase in body temperature, and (d) administration of β-adrenergic blocking agents prior to exercise [28, 47, 57, 60]. On the other hand, PRL release is inhibited by α-adrenergic blockade [28, 47]. Exercise training history (i.e., training type or mode) also seems to have a positive or negative influence on this response to submaximal exercise [28].
PRL changes following maximal exercise or submaximal exercise of 1–2 h duration appear transient in nature [57–59]. Interestingly, Hackney and associates have reported nocturnal PRL levels are 2–3 times greater following 1.5 h of intensive endurance exercise than when no daytime exercise was performed [43, 61].
Exercise-Hypogonadal Males
Basal Hormonal Responses
Retrospective comparative studies examining isolated, single blood samples have found lower testosterone levels in chronically endurance-trained males. The subjects in those studies were typically endurance athletes (e.g., distance runners) who had been involved with the physical training aspects of their sport for 1–15 years. In those studies, total and free testosterone levels were only 60–85% of the levels of matched sedentary controls [7, 40, 59, 62–64]. Many of the early studies reporting this finding suffered from small sample sizes. However, recent work with larger numbers of subjects has substantiated those findings [65, 66]. These low resting testosterone levels seem highly reproducible, and are not just an aberration of the athletes’ seasonal training regime [66]. Furthermore, SHBG levels have not been reported to differ from sedentary, age-matched control men [65].
Prospective studies have also been conducted in which blood samples have been collected over weeks or months of endurance training regimens. Thus far, findings from such studies have been inconsistent. Some reports reveal significant reductions (decreases of 20–40%) in resting testosterone levels following 1–6 months of intensive training [66–70], whereas other studies have found no significant change in resting testosterone after 2–9 months of training [71–74]. Differences in the initial training status of the subjects, or the training dosage administered within these studies, may explain the discrepant findings. It is also possible that some of these prospective studies were too brief compared to the retrospective studies in which the men with low testosterone levels had been training for many years. To this point, landmark work by Safarinejad and colleagues [75] showed that prolonged training exposure (60 weeks) resulted in significant reductions in free and total testosterone, gonadotropins, and stimulated gonadotropin production.
Exercise-hypogonadal men also display other reproductive hormonal abnormalities in addition to low basal testosterone levels. The most frequently reported finding involves no significant elevation in resting LH to correspond with the decrease in testosterone (i.e., hypogonadotropic-hypogonadism) [40, 64, 76]. Additionally, resting levels of PRL may be decreased [40, 64]. Altered LH and PRL levels at rest in EHMC men have been interpreted by some researchers to indicate dysfunction of the hypothalamic-pituitary-testicular axis. These findings for LH and PRL have been reported in several retrospective and prospective studies [7, 40, 62, 64, 76, 77]. Interestingly, the long-term prospective study of Safarinejad and colleagues [75], noted earlier, reported a significant decline in basal LH and FSH with training exposure by 12 weeks.
There are a number of retrospective investigations in which basal, resting blood samples were collected (i.e., serially every 20 or 30 min for 4–8 h periods) in EHMC men and in sedentary control men. Results are similar to those of the isolated-sampling studies: resting total and free testosterone concentrations of the trained subjects were typically only 60–80% of those found in control men [62, 78]. As in isolated single blood-sampling studies, resting LH levels were not significantly elevated. Again, these findings have been interpreted to represent dysfunction in the hypothalamic-pituitary function.
As noted, the hormonal findings described above have primarily been found in endurance athletes (i.e., distance runners and tri-athletes). It is highly unlikely, however, that these hormonal alterations are limited to this athletic—exercise group. The prevalence of these phenomena in endurance athletes most likely represents the tendency of researchers to focus upon this group, following the initial lead of early studies conducted in the 1980s. As scientists studying exercise expand their endocrinological studies to include other athletic groups involved with some components of endurance training, it is highly likely that comparable data will come forward as is beginning to be seen in preliminary findings [79].
Exercise Responses—Testosterone, Gonadotropins, and Other Hormones
Few studies have compared whether EHMC men and normal men respond differently to exercise. Evidence suggests that in response to a single exercise bout (maximal or submaximal in nature) the direction of the hormonal changes is similar. Testosterone and PRL are increased while the gonadotropin responses are variable in both groups of men [40, 77]. In the recovery from exercise, however, the two groups differ. In normal men, the reproductive hormones display some degree of negative feedback “rebound” inhibition during the hours of recovery following exercise [40, 58]. In EHMC men, this rebound effect is diminished or eliminated. Currently, it is unclear whether this represents an adjustment in regulatory aspects of the controlling axis, or whether a temporal displacement shift has occurred in the axis of the EHMC men. Obviously, the resting, basal levels of the hormones in each of these groups are vastly different; therefore, relative changes in response to exercise must be compared. Figure 13.2 displays recovery hormonal responses of exercise-hypogonadal men and normal men as relative change following an identical exercise bout [40].


Fig. 13.2
Comparison of the percent change in hormone levels of exercise-hypogonadal (EHM) condition men versus normal, untrained men. Comparison is between a 4 h(overall mean response) period following a maximal exercise bout and that of a four period of rest in both groups of men. The plotted mean (± SE) responses of each group represent a delta value (i.e., exercise session 4 h overall response –control session 4 h overall response). Abbreviations T testosterone*, LH luteinizing hormone*, PRL prolactin*, C cortisol, E2 estradiol. The * denotes significance differences between the groups (P < 0.05). The data are based upon the findings in reference [40]
Mechanistic Studies
Some studies have attempted to elucidate the mechanism for the proposed hypothalamic-pituitary-testicular axis dysfunction in exercise-hypogonadal men. These studies have focused on examining whether the dysfunction is central (hypothalamic or pituitary) or peripheral (testicular) in nature.
Several investigators have reported that EHMC men have altered PRL and LH release when either an exogenous stimulus and, or an exercise bout are used to provoke hormone secretion [40, 52, 75, 76, 80]. Figures 13.3 and 13.4 illustrate an augmented PRL response and a reduced LH response to metoclopramide and GnRH stimulation, respectively, in EHMC men when compared to the matched sedentary controls [80]. Hyperprolactinemia is associated with decreased circulating testosterone levels [19, 81]. However, while the PRL response to metoclopramide was augmented, there is no evidence that EHMC men are chronically hyperprolactinemic. The converse has been actually been demonstrated (see earlier discussion). The reduction in LH release could also reduce testosterone levels. Reports indicate that testicular sensitivity and response to exogenous stimuli are comparable in exercise-hypogonadal men and sedentary males [40, 80, 82]. In contrast, Kujala et al. reported that the testicular response to a stimulus (human chorionic gonadotropin [hCG]) is attenuated following 4 h of exhaustive exercise [52].



Fig. 13.3
Mean (± SE) integrated area under the PRL response curve over 3 hfollowing the injection of the dopamine antagonist metoclopramide (see reference [80] for dosage). This challenge to the pituitary was performed in endurance exercise-trained male runners and in age-matched sedentary controls. Significant between group differences (P < 0.05) are denoted with an asterisk

Fig. 13.4
Mean (± SE) integrated area under the LH response curve over 3h following the injection of GnRH (gonadorelin hydrochloride) (see reference [80] for dosage). This challenge to the pituitary was performed in endurance exercise-trained male runners and in age-matched sedentary controls. Significant between group differences (P < 0.05) are denoted with an asterisk
Collectively, these findings suggest the development of both a central and a peripheral problem in the hypothalamic-pituitary-testicular axis; however, data in this area are limited and not yet definitive.
Cortisol
Researchers have demonstrated that acute pharmacological or pathological increases in cortisol secretion are associated with a decrease in circulating testosterone levels [38, 83]. Several investigators have alluded to these hormonal changes as a potential mechanism for the low testosterone levels in EHMC men [6, 27, 38, 83]. A single, acute exercise bout at high intensity (>60% of maximal aerobic capacity) could transiently increase circulating cortisol, which could bring about the observed reductions in testosterone via inhibitory effects on GnRH, LH or Leydig cell function [19]. Current evidence suggests, however, that hypercortisolemia is an unlikely mechanism for exercise-hypogonadism, since there are relatively small, transient changes in cortisol levels in response to exercise [43, 49, 50, 55, 58, 84–87], and exercise-induced changes in cortisol are well within the physiological range, regardless of whether the exercise is of moderate or high intensity. This point is illustrated in Fig. 13.5 [88]. Nevertheless, systematic research examining the role of exercise-induced cortisol changes upon testosterone production remains inadequate.


Fig. 13.5
Mean cortisol levels over 24 h in endurance athletes on three separate occasions; Filled circle control—baseline day with no exercise, Filled triangle exercise day involving two moderate intensity exercise sessions morning and afternoon, and Filled square exercise day involving two high intensity exercise sessions morning and afternoon. Significant increases (P < 0.05) in cortisol occurred on the exercise days; however, the changes were transient in nature. The data are based upon the results in reference [88]
Physiological Consequences of Low Testosterone Levels in Athletes
There is evidence that the low resting testosterone level in men doing endurance training has detrimental effects on testosterone-dependent physiological processes. However, the extent of the evidence is somewhat limited. Several reports of decreased spermatogenesis or oligospermia in EHMC men have been published [21, 75, 89–93]. One of the most well-controlled studies was by Arce et al. [90]. Some key findings from that study are displayed in Table 13.2. Other investigators have reported that endurance-trained men may have a lowered sex drive—libido, but a direct cause-and-effect linkage between a lower sex drive and circulating testosterone levels was not proven in those reports [82, 91, 94–97]. Accordingly, other factors may have affected the libido of those athletes (e.g., overall fatigue and psychological stress), but this area has not been well studied in exercising men [22, 94]. Additionally, there is some evidence that endurance training has no effect upon sperm characteristics and the spermatogenesis process [74].
Table 13.2
Semen characteristics of endurance-trained runners, resistance trained weight lifters, and sedentary controls (values are means ± SE)
Runners | Weight lifters | Controls | P value | |
---|---|---|---|---|
(N = 10) | (N = 8) | (N = 10) | ||
Totals | ||||
Volume (ml) | 4.2 ± 0.5 | 3.0 ± 0.5 | 2.5 ± 0.5 | 0.9 |
Sperm density (x106·ml−1) | 78 ± 12* | 122 ± 15 | 176 ± 25 | <0.01 |
Total sperm count (x106) | 332 ± 74 | 342 ± 36 | 376 ± 59 | 1.86 |
Normal motile count (x106) | 55 ± 11 | 104 ± 20 | 107 ± 22 | 0.09 |
Motility | ||||
Forward progressive (%) | 40.8 ± 4.7** | 58.0 ± 4.6 | 58.7 ± 2.4 | <0.01 |
Nonprogressive (%) | 5.0 ± 1.0 | 2.8 ± 1.5 | 2.0 ± 1.0 | 0.17 |
Nonmotile (%) | 54.2 ± 4.9 | 39.2 ± 3.9 | 39.3 ± 1.9 | 1.01 |
Morphology | ||||
Normal (%) | 40.2 ± 2.1† | 54.8 ± 2.9 | 47.0 ± 3.3 | <0.01 |
Large (%) | 2.7 ± 0.8 | 1.7 ± 0.9 | 2.3 ± 1.0 | 0.76 |
Small (%) | 4.3 ± 0.8 | 2.6 ± 0.7 | 2.4 ± 0.5 | 0.10 |
Amorphous (%) | 34.1 ± 2.5 | 30.1 ± 2.6 | 37.4 ± 2.8 | 0.19 |
Immature (%) | 17.2 ± 2.4** | 10.5 ± 2.1 | 10.9 ± 1.2 | 0.03 |
Round cells (x106) | 8.3 ± 1.7** | 0.6 ± 0.4 | 2.5 ± 0.9 | <0.01 |
In vitro sperm penetration of CM—Penetrak (mm) | 22 ± 5* | – | 43 ± 7 | 0.04 |
Relative to the androgenic-anabolic actions of testosterone, there are no documented detrimental effects of the lower testosterone levels (i.e., decreased protein synthesis and muscle mass development). However, this area needs more thorough examination. An additional area in need of research concerns the impact of the exercise-associated decline in testosterone levels on bone demineralization in trained men. Currently, there are no conclusive findings that endurance training results in male osteoporosis [98]. In fact, the bone mineral density of the lower extremities was increased in high mileage runners [99–101]. On the other hand, several compelling case reports have described male athletes with extremely low testosterone levels and reduced bone mineral density [102, 103].
Thus the question arises, is it necessary for endurance-trained males to supplement with testosterone-like substances in order to safeguard against loss of androgenic-anabolic processes? This question has not been addressed thoroughly in the literature. In one case study, Burge et al. [82] described a male runner with hypogonadotrophic hypogonadism who responded favorably to clomiphene citrate treatment over a 5-month period. Testosterone and gonadotrophin concentrations increased into the normal range, and the subject’s sexual function improved. Whether treatment was necessary is an issue of debate. There is little evidence that disruptions in testosterone-dependent processes are sufficient to warrant action, but physicians must use their own medical judgment on a case-by-case basis. In extreme cases, especially among men with a very low body mass index, such steps may be necessary, and a well-developed pharmacological course of therapy could be highly advantageous and efficacious (see section “Anabolic Steroid—A Banned Substance”).
Conversely, there may be beneficial physiologic adaptations from lowered testosterone levels. Some research indicates that lowering testosterone levels (i.e., but, not to hypogonadal levels) may have cardiovascular protective effects and decrease the risk of coronary heart disease [104]. A study from Germany demonstrated that a pharmacologically induced reduction in endogenous testosterone levels resulted in significant increases in high-density lipoprotein in men [105]. Whether the lowering of testosterone directly contributed to the exercise-related increase in HDL remains to be determined [106]. Nonetheless, it is important to recognize that increased physical activity promotes a healthy cardiovascular risk profile, including increased circulating HDL [107].
The Overtraining Syndrome
Several researchers have suggested that reproductive hormones in exercise-hypogonadal men are suppressed because they are “overtraining” and/or are developing the “Overtraining Syndrome.” This terminology can be confusing to non-Exercise Physiology researchers; hence some explanation is warranted. The terms “Overtraining Syndrome” and “overtraining” are frequently used interchangeably. The term “overtraining” actually refers to the process of heavier than usual exercise training, while the term “Overtraining Syndrome” refers to the product of too much of the overtraining process [2, 4, 7–9, 108–112]. The Overtraining Syndrome is a pathological condition in which an athlete experiences consistent and persistent exercise performance incompetence that does not reverse itself after a few days of rest and recovery. Furthermore, there is no underlying medical reason or explanation for the declining performance. This exercise performance impairment can manifest itself within athletic competition as well as during exercise training. Concurrent with the declining physical performance is a host of other psycho-physiological consequences that are adverse and negative in nature. Some of the most general and commonly reported consequences and symptoms are listed in Table 13.3.
Table 13.3
Consequences and symptoms of the overtraining syndrome
↓ Physical performance |
↑ Severe constant fatigue |
↑ Persistent muscle soreness |
↑ Overuse muscular-skeletal injuries |
↓ Appetite |
↑ Lethargy |
↑ Depression |
↑ Disturbed sleep patterns |
↑ Overall mood disturbances—shifts |
↑ Immune system deficits |
↑ Mental concentration difficulties |
Δ Submaximal—maximal heart rate responses to exercise (↑, ↓) |
↓ Maximal oxygen uptake |
Δ Submaximal—maximal lactate response to exercise (↑, ↓) |
One commonly reported endocrine change among overtrained athletes is suppressed circulating levels of testosterone [2, 4, 7, 9, 110–112]. Furthermore, in some cases, suppressed levels of LH, FSH, and inhibin have been reported as well as a reduced testosterone to cortisol ratio [27, 108–113]. While these changes appear nearly identical to those reported for EHMC men, it does not seem to represent the same phenomena. The hormone changes that are found in overtraining athletes appear to be only temporary. When the exercise training load of these overtraining athletes is reduced, their diets are adjusted to allow macro-nutrient—caloric balance, and increased periods of rest are incorporated into the training program, their hormonal profiles return to normal [113, 114]. Incorporation of more rest into the training of EHMC men seems to have no substantial impact upon their resting hormone levels. Neither do EHMC men display Overtraining Syndrome symptoms (see Table 13.3). Furthermore, most researchers studying the exercise-hypogonadal issue have been careful to insure that their subjects under investigation are not going through periods of intensive training; and that when they are evaluated they are well rested.
Thus, the hormonal changes found in EHMC men appear to be a more stable accommodation within the hypothalamic-pituitary-testicular axis while those in overtraining athletes are transient (i.e., returning to normal after adequate rest-recovery) and are associated with a declining physical performance.
Athletes and Anabolic-Androgenic Steroids
Anabolic-androgenic steroids are pharmacological agents that are similar to testosterone in structure [115, 116]. They have anabolic-androgenic actions like testosterone; however, these agents have been chemically modified in an effort to enhance their anabolic actions. Athletes use anabolic-androgenic steroids in an attempt to facilitate physiological development beyond that achieved with exercise training alone (e.g., increased body weight, muscular strength-power-speed or endurance) [115, 116]. These agents are used by athletes involved in many sports. However, sports such as track and field (mostly in the throwing events), weight-lifting, bodybuilding, and football report the highest prevalence of usage [103]. Regrettably, evidence points to nonathlete use being on the rise with the intent being for altering the body image [117, 118]. For the remainder of the discussion, anabolic-androgenic steroids will be referred to as AAS.
Physiologic Adaptation and Exercise Performance
It is now clearly established that testosterone and AAS supplementation increase muscle mass and maximal voluntary muscle strength [119–122]. Early studies suggested equivocal effects of AAS usage on athletic or exercise performance [119]. It is important to recognize, however, that because of ethical reasons most of those sanctioned studies did not administer AASs at the high dosages used by athletes. This prompted researchers to report no effects whereas anecdotal reports by individual users touted large, substantial effects. Those anecdotal reports were tainted by their lack of scientific control for confounding factors such as extent of training and “the placebo effect.” Nonetheless, the findings in those “non-experimental” studies suggested body mass gains of 10 or 20 kg, as well as up to 30% increases in muscle strength [120]. Several well-controlled short-term studies have found smaller, but significant, increases in body mass, lean body mass, muscular strength, and concurrent reductions in fat mass [119, 120].
AAS usage is not limited to “weight-lifting–power” athletes. Endurance athletes (e.g., distance runners and tri-athletes) have also been found to use AAS. Interestingly, relative to endurance athletes, most studies have failed to demonstrate a substantial, beneficial effect of AAS on maximal oxygen consumption (i.e., key determinate to aerobic—endurance capacity) [115–119]. It has been proposed that endurance athletes who use AAS choose to do so because they recover from exercise training bouts more rapidly. This idea seems logical since performing more exercise training is a major stimulus to improve the cardiovascular-respiratory system, and thereby aerobic performance, and androgen treatment should negate the decline in testosterone that normally follows heavy exercise.
In addition to the myogenic effects of exogenous testosterone use, androgens have also promote erythropoiesis (i.e., hence potentially increasing oxygen carrying capacity of the blood and cardiovascular function; see later section, Erythropoietic Effects) [123]. The impetus for this line of research stemmed from early studies that found following puberty (and subsequent increases in testosterone), men developed a higher red blood cell and hemoglobin mass [124]. Since then, the use of exogenous synthetic androgens has been shown to affect hematocrit by stimulating erythropoietin production, altering iron metabolism by suppressing hepcidin, and by direct effect on bone marrow cells [123, 125]. Hypogonadal men have a lowered hematocrit and hemoglobin [126], and androgen treatment can return those parameters to normal levels [127], although it is unclear whether the same effect would occur in exercise-induced hypogonadism.
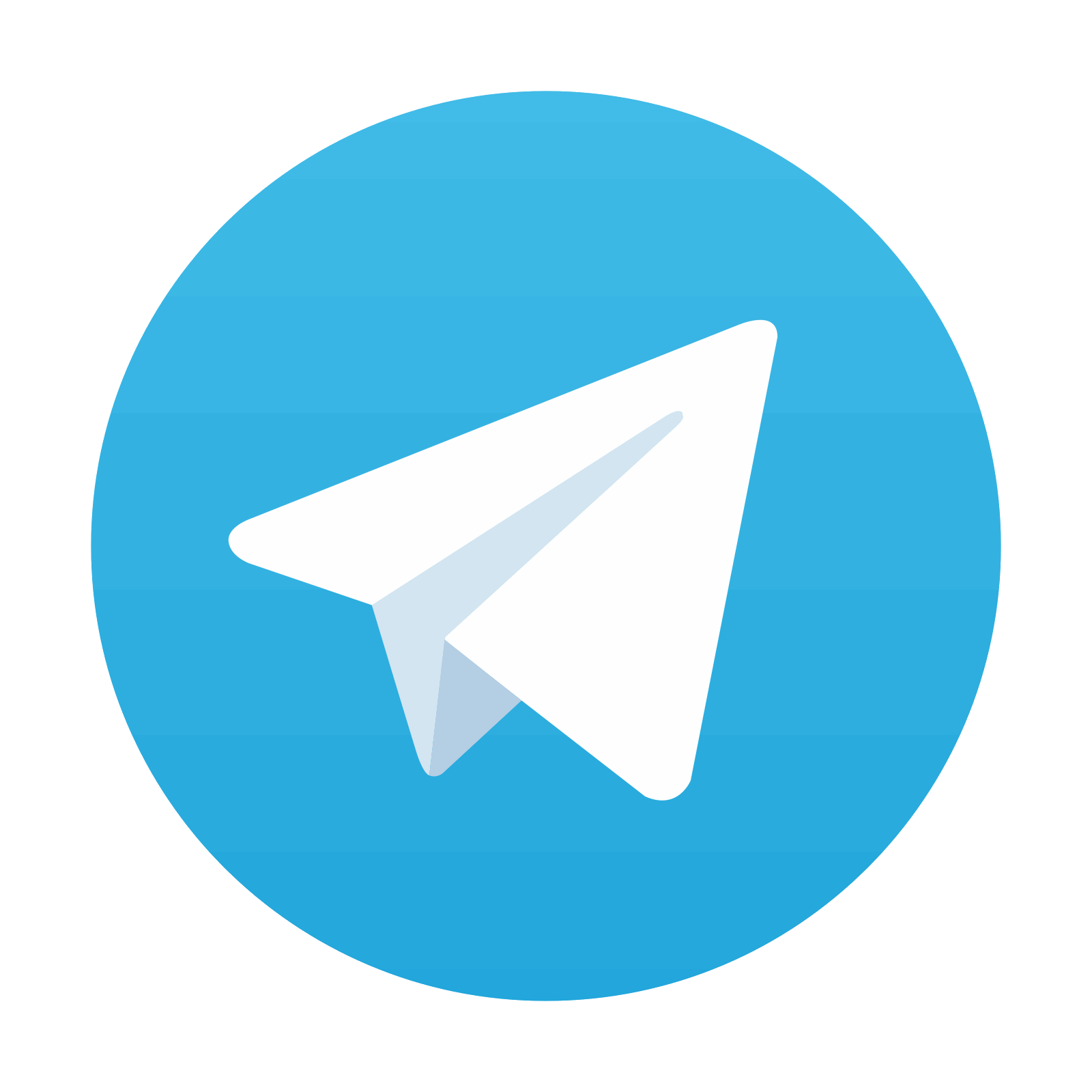
Stay updated, free articles. Join our Telegram channel

Full access? Get Clinical Tree
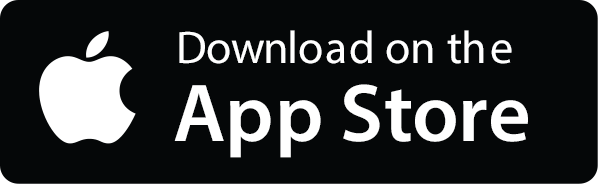
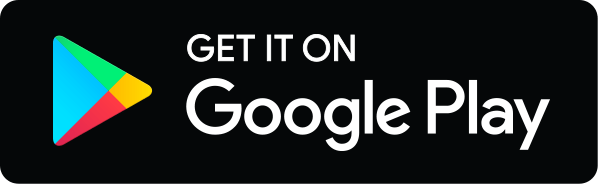