© Springer-Verlag Berlin Heidelberg 2015
Nima Rezaei (ed.)Cancer Immunology10.1007/978-3-662-44006-3_1616. Epigenetics and microRNAs in Cancer
(1)
Department of Pediatric Hematology/Oncology, Children’s Center for Cancer and Blood Diseases, Norris Comprehensive Cancer Center, University of Southern, California, Children’s Hospital Los Angeles, Los Angeles, CA, USA
(2)
Department of Pediatric Hematology/Oncology, Children’s Hospital Los Angeles, 4650 Sunset Blvd MS #57, Los Angeles, CA 90027, USA
(3)
Department of Pediatric Hematology/Oncology and Molecular Microbiology and Immunology, Children’s Hospital Los Angeles, 4650 Sunset Blvd MS #57, Los Angeles, CA 90027, USA
16.1 Introduction
MicroRNAs (miRNAs) are small noncoding RNAs (ncRNAs) which regulate gene expression by directly binding mostly, but not exclusively, to the 3′-untranslated region (3′-UTR) of target mRNAs [1]. In 1993, Victor Ambros first identified a small ncRNA, called lin-4, able to regulate the expression of a gene called lin-14 involved in the development of C. elegans [2]. In 2001, Lagos-Quintana M. et al. showed for the first time that many of these small ncRNAs (in the meantime called microRNAs) are present not only in invertebrates but also in vertebrates [3]. In 2002, Croce’s group provided the first evidence of miRNA involvement in cancer by showing that a specific cluster of miRNAs (namely, the miR-15a/16-1 cluster) is located in the frequently deleted chromosomal region 13q14 in chronic lymphocytic leukemia (CLL) [4]. In 2005 Frank Slack supported this molecular evidence of miRNA involvement by demonstrating that let-7 directly targets the RAS oncogene in lung cancer [5]. In the same year, Cimmino et al. found that the miR-15a/16-1 cluster directly targets the antiapoptotic BCL2 gene in human CLL [6]. From this time on, we assist at a plethora of studies identifying dysregulation of miRNAs in almost all types of human cancers and unraveling their contribution to human carcinogenesis by identifying which genes are modulated by the dysregulated miRNAs. Overall, these studies clearly state that aberrancies of the miRNome (defined as the full spectrum of miRNAs in a specific genome) contribute to human cancer development and can be therapeutically targeted to restore miRNA expression to normal [7]. Moreover, it has become clearer that miRNA involvement goes beyond cancer, since they are involved in a variety of biological processes, spanning from development, differentiation, apoptosis, and proliferation to senescence and metabolism [8–13].
MiRNAs are genes, like any other protein coding gene (PCG), transcribed by RNA polymerase II into a capped and polyadenylated precursor, called pri-miRNA [14, 15]. A double-stranded RNA-specific ribonuclease called Drosha, in conjunction with its binding partner DGCR8 (DiGeorge syndrome critical region gene 8, or Pasha), cleaves the pri-miRNA into a hairpin-shaped RNA precursor (pre-miRNA), about 70–100 nucleotides (nt) long [16]. Transferred to the cytoplasm by Exportin 5, the pre-miRNA is cleaved into an 18–24 nt duplex by a ribonucleoproteic complex, composed of a ribonuclease III (Dicer), and TRBP (HIV-1 transactivating response RNA binding protein). Finally, the duplex interacts with a large protein complex called RISC (RNA-induced silencing complex), which includes proteins of the Argonaute family (Ago1-4 in humans), which drives one strand of the duplex (the so-called mature miRNA) mainly, but not exclusively, to the 3′-UTR of the target mRNAs. Overall, miRNAs exert its effect by modulating the expression of the target mRNAs either by mRNA cleavage or by translational repression. In 2007, Vasudevan et al. discovered that miRNAs can also increase the expression of target mRNAs [17]. Each miRNA can target several different transcripts. For instance, it has been demonstrated that a cluster of two miRNAs (namely, miR-15a and miR-16) can affect the expression of about 14 % of the human genome in a leukemic cell line [18]. In addition, the same mRNA can be targeted by several miRNAs [19].
Epigenetics is defined as all heritable changes in gene expression not associated with concomitant alterations in the DNA sequence. In a traditional sense, gene epigenetic regulation usually includes DNA promoter methylation and chromatin histone modifications which are catalyzed by specific enzymes, overall indicated as effectors of the epigenetic machinery. However, if we consider the above definition, also miRNA gene regulation sensu stricto represents a component of epigenetics. Interestingly, it has been discovered that there is a two-way correlation between miRNAs and other epigenetic mechanisms: miRNAs can regulate the expression of effectors of the epigenetic machinery and miRNA genes undergo the same epigenetic regulatory mechanisms of any other PCG. These two main aspects of miRNome-epigenome cross-regulation and their implications in human carcinogenesis will be the main focus of this chapter.
16.2 MiRNAs Regulate Effectors of the Epigenetic Machinery
In 2007, Fabbri et al. provided the first evidence that miRNAs can affect the expression of epigenetically regulated PCG in cancer by directly targeting key effectors of the epigenetic machinery, such as DNA methyltransferases (DNMTs) [20]. The miR-29 family (composed of miR-29a, miR-29b, and miR-29c) can directly silence the expression of de novo DNMT3A and DNMT3B in non-small cell lung cancer (NSCLC), leading to a global hypomethylation status of cancer cells and re-expression of tumor suppressor genes (TSGs) such as FHIT and WWOX, whose expression is silenced in NSCLC by promoter hypermethylation. As a result of the re-expression of these TSGs, NSCLC cells undergo apoptosis both in vitro and in an in vivo xenograft model [20]. Subsequently, Garzon et al. showed that in addition to directly targeting de novo DNMTs, miR-29b is also capable of targeting the maintenance DNMT1, even though in an indirect way: by directly silencing Sp1, a transactivator of DNMT1 [21]. These combined effects of miR-29s on all three major DNMTs highlight their relevance for epigenetic processes and explain the profound effects of their restoration on the global methylation status of cells. MiRNAs such as the miR-29 family, able to directly target effectors of the epigenetic machinery, have been called “epi-miRNAs.” In mouse embryonic stem (ES) cells, two independent groups have shown that members of miR-290 cluster directly target RBL2, an inhibitor of DNMT3 genes [22, 23]. ES Dicer null cells are characterized by no expression of the miR-290 cluster, overexpression of RBL2, and disruption of de novo methylation pathway, leading to increased telomere recombination and aberrant telomere elongation. Restoration of the miRNA cluster reverted this phenotype [23, 22]. Interestingly, the regulatory effect of miR-290 cluster on de novo DNMTs was not observed in human embryonic kidney 293 cells following Dicer knockdown, suggesting that miR-290 targeting effect on DNMT3s might be cell- and/or species-specific [22].
Another important family of epi-miRNAs is the miR-148a/b-152 family. In 2008, Duursma et al. showed that miR-148a and miR-148b can indeed bind to the coding region (not the 3′-UTR) of DNMT3b mRNA, affecting the expression of this gene [24]. This seminal study also concluded that by binding to this unusual site, miR-148 family might be responsible for the several different splice variants of DNMT3b [24]. A role for the miR-148a/b-152 family was further confirmed in cholangiocarcinoma, where it was shown that these miRNAs, in addition to miR-301, can directly target DNMT1, and their expression is silenced by IL-6, which is involved in cholangio-cancerogenesis [25]. This paper provided the first evidence of a correlation between epi-miRNAs, inflammation, and cancer. In 2010, Das et al. showed that all-trans-retinoic acid (ATRA)-treated neuroblastoma cells undergo downregulation of MYCN, hence leading to overexpression of MYCN repressed miRNAs such as miR-152, miR-26a/b, and miR-125a/b [26]. They also showed that these miRNAs are epi-miRNAs in this model, since they downregulate DNMT1 and DNMT3B expression, leading to re-expression of epigenetically silenced NOS1, which promotes neural cell differentiation. Also, the expression of miR-152 was normally downregulated with concurrent increase of DNMT1 expression in HBV-induced HCCs [27]. More recently, Wang et al. identified miR-342 as another epi-miRNA involved in colon carcinogenesis [28]. They showed that the expression of miR-342 is inversely correlated to DNMT1 levels in colorectal cancer (CRC) tissues and cell lines, and that this miRNA targets DNMT1, leading to reactivation of epigenetically silenced TSGs such as ADAM23, Hint1, RASSF1A, and RECKS. Functionally, restoration of miR-342 resulted in a reduction of DNMT1 expression, reduced cell proliferation, and invasiveness in CRC cells and inhibition of tumor growth and lung metastasis formation in nude mice [28]. In 2010, viral epi-miRNAs have been shown to control the epigenetic machinery of host cells through DNMTs [29]. MiR-K12-4-5p, a Kaposi sarcoma-associated herpesvirus (KSHV) miRNA, was found to regulate the expression of DNMT1, 3A, and 3B indirectly, by targeting the expression of Rbl2, a known repressor of DNMT1, 3A, and 3B transcription. Ectopic expression of miR-K12-4-5p reduces Rbl2 protein expression and increases DNMT1, 3A, and 3B mRNA levels in 293 cells, thus affecting the overall epigenetic reprogramming of the host cell [29].
Epi-miRNAs are also involved in regulating the expression of histone deacetylases (HDACs) and Polycomb Repressive Complex (PRC) genes. For instance, HDAC4 is a direct target of both miR-1 and miR-140 [30, 31], while miR-449a binds to the 3′-UTR region of HDAC1 [32]. HDAC1 is upregulated in several kind of cancers, and miR-449a re-expression in prostate cancer cells induces cell-cycle arrest, apoptosis, and a senescent-like phenotype by reducing the levels of HDAC1 [32]. Recently, Jeon et al. showed that miR-449a,b regulate HDAC1 expression by directly targeting its 3′UTR transcript, indicating that this might be one of the reasons for the low miR-449a, b expression and the high expression of HDAC1 in lung cancer [33]. MiR-140 has also been shown to be involved in chemoresistance mechanisms by targeting HDAC4 [34]. Inhibition of endogenous miR-140 by locked nucleic acid (LNA)-modified anti-miRNAs partially sensitized resistant colon cancer stemlike cells to 5-FU treatment by increasing HDAC4 levels, leading to a G1 and G2 phase arrest [34]. Low expression of miR-9 along with high expression levels of HDACs (HDAC4 and 5) were discovered in Waldenstrom macroglobulinemia (WM) [35]. Mir-9 targets HDAC4 and HDAC5 in WM cells. Overexpression of miR-9 causes downregulation of HDAC4, 5, leading to an upregulation of acetylated-histone-H3 and acetylated-histone-H4. This provides evidence that the loss of miR-9 might be responsible for upregulation of HDAC4 and HDAC5 in WM cells, contributing to the pathogenesis of WM disease [35].
EZH2 is the catalytic subunit of the Polycomb Repressive Complex 2 (PRC2) and is responsible for heterochromatin formation by trimethylating histone H3 lysine 27 (H3K27me3), leading to the silencing of several TSGs. Varambally et al. showed that in prostate cancer cell lines and primary tumors, the expression of miR-101 decreases during cancer progression, inversely correlating with an increase of EZH2. These findings are suggestive of a role as epi-miRNA for miR-101, a hypothesis which was tested and confirmed by showing that miR-101 directly targets EZH2 both in prostate and in bladder cancer models [36, 37]. Moreover, miR-101-mediated suppression of EZH2 inhibits cancer cell proliferation and colony formation, revealing a TSG role for miR-101, mediated by its modulatory effects on cancer epigenome [37]. The inverse correlation between miR-101 and EZH2 was also observed in glioblastoma [38], gastric cancer [39], and NSCLC [40]. In prostate cancer it has been shown that miR-101 can be inhibited by androgen receptor and HIF-1α/HIF-1β [41]. Ectopic expression of miR-26a targets EZH2 in Burkitt’s lymphoma, leading to reduced cell proliferation, increased percentage of cells in G1-phase, and increased apoptosis in Raji and Namalwa cells [42]. Intriguingly, the authors also found that c-Myc negatively regulates miR-26a, therefore maintaining high EZH2 expression levels in cells and significantly contributing to c-Myc-induced tumorigenesis [42]. In 2009, Juan et al. analyzed a regulatory double-negative feedback loop between miR-214 and EZH2 in controlling PcG-dependent gene expression during differentiation [43]. PcG proteins suppress the transcription of miR-214 in undifferentiated skeletal muscle cells (SMC). Ectopic expression of miR-214 directly targets EZH2, increases myogenin expression, and promotes muscle differentiation [43]. EZH2 is also highly expressed in nasopharyngeal carcinoma (NPC) patients and correlates with a higher risk of relapse [44]. MiR-26a, miR-98, and miR-101, whose expression is consistently downregulated in human NPC specimens when compared to normal nasopharyngeal epithelial tissue samples, have been shown to directly target EZH2 [44], suggesting a prognostic role for these three miRNAs in NPC. Recently, there has been an extensive series of studies unraveling the central role of miR-101 in the regulation of EZH2, in several types of cancer. In hepatoma tissues, it was shown that miR-101 and miR-29c are downregulated, but their expression can be restored (leading to reduced levels of EZH2, EED, and H3K27me3 proteins) after treatment with TPA (12-O-tetradecanoylphorbol 13-acetate), which is dependent on protein kinase C (PKC) and ERK pathways in HepG2 cells [45]. Also, Smiths et al. have established a pro-angiogenic effect of miRNA-101 working together with EZH2 and VEGF during the process of angiogenesis [46]. The group analyzed the expression of miR-101 in endothelial cells derived from glioma patients and found it to be low. VEGF downregulates the expression of miR-101 resulting in increased protein expression of EZH2 and induces the elongation of endothelial cells leading to a pro-angiogenic response. Transfection with pre-miR-101, or EZH2 siRNA, or treatments with DZNep, a small inhibitor of EZH2 methyltransferase activity, reverses this process in HBMVECs controls, providing a network between VEGF/miR-101/EZH2 proteins toward pro-angiogenic response in endothelial cells [46]. A summary of the described epi-miRNAs is provided.
Overall, these studies indicate that epi-miRNAs can modulate several key effectors of the epigenetic machinery, which indirectly affects the expression of epigenetically regulated genes. Considering that inactivation of TSGs by epigenetic mechanisms represents one of the main strategies adopted by cancer cells to promote their oncogenic phenotype, it is of the utmost importance to completely dissect these mechanisms, since they could provide new molecular targets for anticancer treatments.
16.3 MiRNAs Are Epigenetically Regulated in Several Types of Human Cancers
As previously anticipated, the relationship between miRNome and epigenome is bidirectional. Not only do miRNAs regulate the expression of effectors of the epigenetic machinery, but they also undergo the same epigenetic regulation of any other PCG.
By treating bladder cancer cell lines with both a DNA demethylating agent (5-aza-2′-deoxycytidine, 5-AZA) and an HDAC inhibitor (4-phenylbutyric acid), Saito et al. found that about 5 % of all human miRNAs increased their expression levels [47]. MiR-127 was the most upregulated after this treatment, and its re-expression led to direct targeting and downregulation of the oncogene BCL-6, inducing a tumor suppressor function. MiR-127 is part of a cluster which includes miR-136, miR-431, miR-432, and miR-433 and is embedded in a CpG island region; however, miR-127 is the only member of the cluster whose expression increases upon treatment with the two epigenetic drugs [47]. Moreover, when each drug was used alone, no variation in miR-127 expression was observed [47], suggesting that both DNA methylation and histone modifications affect the epigenetic regulation of miR-127. This seminal work shows that indeed miRNAs undergo epigenetic regulation, that it is a complex epigenetic regulation (involving both methylation and histone modifications), and that there are differences among miRNAs which even belong to the same cluster. Lujambio et al. created a double knockout (DKO) for DNMT1 and DNMT3B in the CRC cell line HCT-116 and compared miRNA expression profile of DKO and wild-type cells. About 6 % analyzed miRNAs were re-expressed in the DKO cells [48]. Among them, miR-124a (embedded in a CpG island heavily methylated in this cell line) was re-expressed, reducing the levels of its direct target gene CDK6 and impacting on the phosphorylation status of CDK6-downstream effector Rb protein [48]. Prosper’s work has identified a signature of 13 miRNAs embedded in CpG islands, with high heterochromatic markers (such as high levels of K9H3me2 and/or low levels of K4H3me3) in acute lymphoblastic leukemia (ALL) patients [49, 50]. Among these, miR-124a was methylated in 59 % of ALLs, and its promoter hypermethylation was associated with higher relapse rate and mortality rate vs. non-hypermethylated cases; hence, miR-124a promoter methylation status was an independent prognostic factor for disease-free and overall survival [50]. Finally, supporting Lujambio’s results, also in ALL the impact of miR-124a in the CDK6-Rb pathway was confirmed by showing that miR-124a directly silences CDK6 [50]. Hypermethylation of miR-124a promoter is also involved in the formation of epigenetic field defect which is a gastric cancer predisposing condition characterized by accumulation of abnormal DNA methylation in normal-appearing gastric mucosa, mostly induced by H. pylori infection [51]. These findings also suggest that miR-124a promoter hypermethylation is an early event in gastric carcinogenesis. MiR-107, another epigenetically controlled miRNA, targets CDK6 in pancreatic cancer as well and impacts this oncogenic pathway [52]. In HCT-116 cells, deficient for DNMT1 and DNMT3B, Bruckner et al. showed increased expression of let-7a-3, an miRNA normally silenced by promoter hypermethylation in the wild-type cell line [53]. In lung adenocarcinoma, primary tumors let-7a-3 promoter was found hypomethylated with respect to the normal counterpart [53], whereas hypermethylation of let-7a-3 promoter was described in epithelial ovarian cancer, paralleled the low expression of insulin-like growth factor-II expression, and was associated with a good prognosis [54]. Therefore, DNA methylation could act as a protective mechanism by silencing miRNA with oncogenic function. Also, the miRNA-200 family participates in the maintenance of an epithelial phenotype, and loss of its expression can result in epithelial to mesenchymal transition (EMT). Furthermore, the loss of expression of miR-200 family members is associated with an aggressive cancer phenotype. Vrba et al. found that hypermethylation of the miR-200c/141 CpG island is closely linked to their inappropriate silencing in cancer cells, and the epigenetic regulation of this cluster appears evolutionarily conserved, since similar results were obtained in mouse [55]. Interestingly, no variation in miRNA expression was observed in lung cancer cells treated with either demethylating agents or HDAC inhibitors or their combination [56]. Another miRNA which is under epigenetic control is miR-1. In hepatocarcinoma, miR-1 is frequently silenced by promoter hypermethylation [57]. However, in DNMT1 null HCT-116 cells (but not in DNMT3B null cells), hypomethylation and re-expression of miR-1-1 were observed [57], revealing a key role for the maintenance DNMT in the regulation of this miRNA. Han et al. observed that neither 5-AZA nor DNMT1 deletion alone can recapitulate miRNA expression profile of DKO DNMT1/DNMT3B HCT-116 cells [58]. Also, Lehmann et al. found that in breast cancer cell lines, 5-AZA re-activates miR-9-1 (hypermethylated in up to 86 % of primary tumors), but not miR-124a-3, miR-148, miR-152, or miR-663 (hypermethylated as well) [59]. Previously, Meng et al. observed that in malignant, but not in normal cholangiocytes, 5-AZA induces re-expression of miR-370 [60]. Overall, these results indicate that the epigenetic control of miRNAs is both cancer specific and miRNA specific. More recently, Chang and Sharan reported that BRCA1 recruits the HDAC2 complex to the miR-155 promoter, which is consequently silenced epigenetically through the deacetylation of H2A and H3 histones [61]. The study also showed the upregulation of miR-155 in BRCA1-deficient or BRCA1-mutant human tumors. The knockdown of miR-155 in a BRCA1 mutant tumor cell line attenuates in vivo tumor growth. However, a knockdown of BRCA1 results in a two- to threefold increase in miR-155 levels in vitro. In contrast, a 50 to 150-fold increase in miR-155 in human breast cancer cell lines or tumor samples was observed, suggesting that this increase may not be caused only by BRCA1 loss; other transcription factors may activate the miR-155 promoter after it is epigenetically activated due to the loss of BRCA1 [61]. Mazar et al. studied which miRNAs were re-expressed upon treatment of a melanoma cell line with demethylating agents [62]. Among the 15 re-expressed miRNAs, miR-375 and miR-34b were also involved in melanoma progression [62]. Liu et al. [63] found that miR-182 was significantly upregulated in human melanoma cells after combined treatment with 5-AZA and trichostatin A. Genome sequence analysis revealed the presence of a prominent CpG island 8–10 kb upstream of miR-182, but methylation analysis showed that this genomic region was exclusively methylated in melanoma cells, not in normal human melanocytes. Since miR-182 has been shown to harbor oncogenic properties, this finding raises a possible concern for melanoma patients treated with epigenetic drugs [63]. MiR-31 maps at 9p21, a genomic region frequently deleted in solid cancers including melanoma. Asangani et al. [64] found recurrent downregulation of miR-31 in melanoma primary tumors and was associated with genomic loss or epigenetic silencing by DNA methylation and EZH2-mediated histone methylation. Moreover, miR-31 overexpression resulted in downregulation of EZH2 and a derepression of its target gene rap1GAP. The increased expression of EZH2 was associated with melanoma progression and poor overall survival [64].
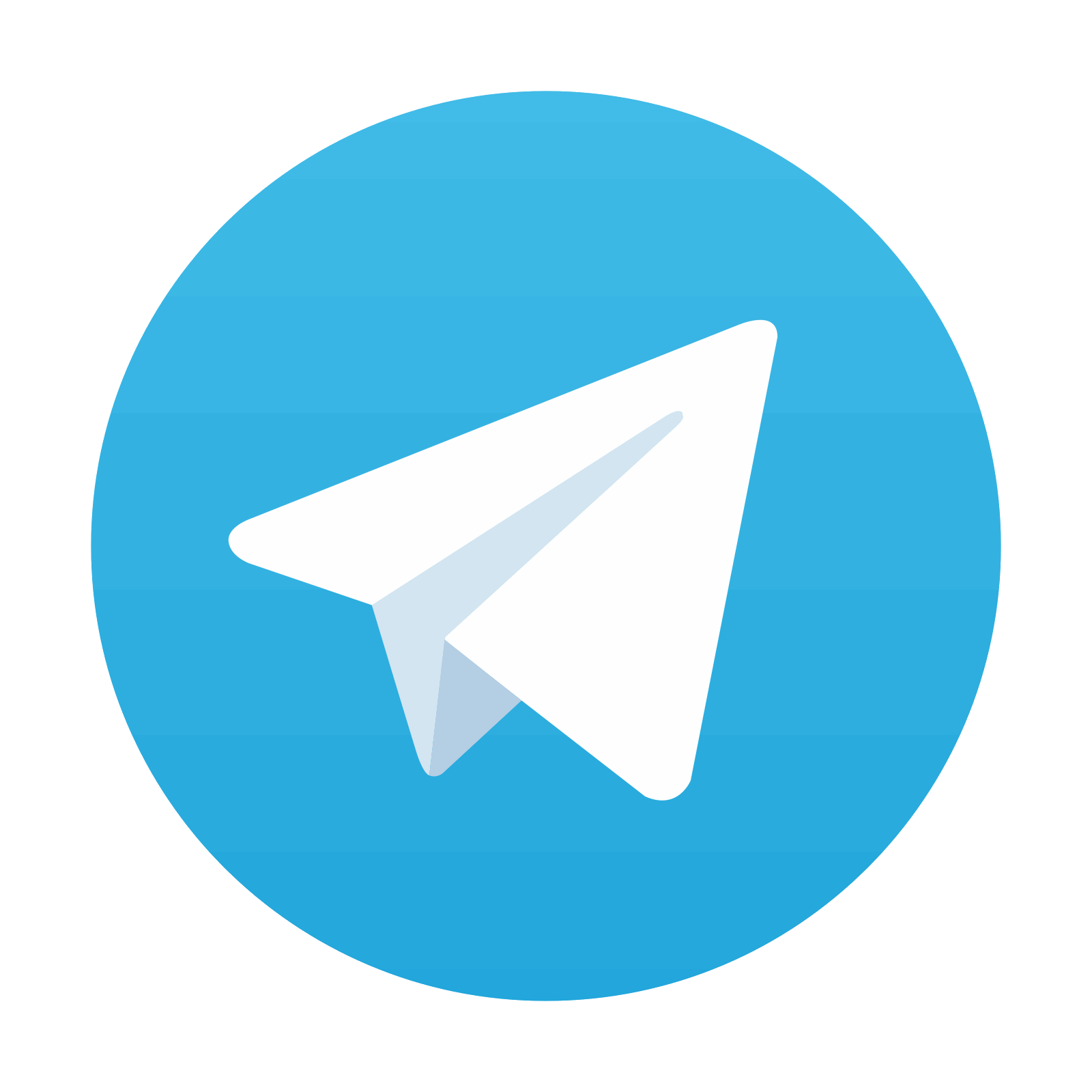
Stay updated, free articles. Join our Telegram channel

Full access? Get Clinical Tree
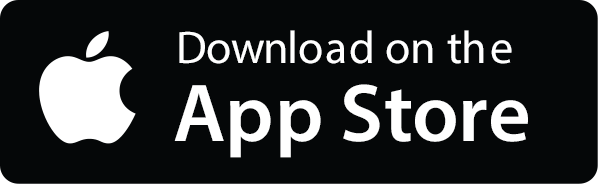
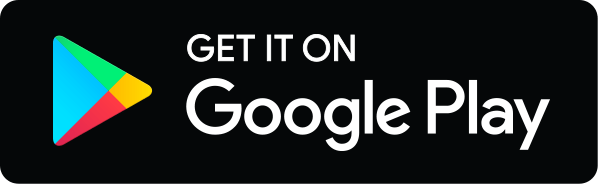