THYROID CANCER
Thyroid cancer is the most common endocrine malignancy. It has the highest incidence in the United States and is increasing worldwide. In 2014, approximately 63,000 new cases of thyroid carcinoma were diagnosed in the United States, accounting for 4% of all new malignant disease. Three of four all new thyroid cancer diagnoses are made in women, corresponding to the threefold higher rates seen in women between 2007 and 2011 (1). Thyroid cancer occurs less frequently in children compared to adults, with a peak incidence of around 50 years. Despite this, overall long-term survival remains favorable (2). Histologic types (Table 43-1) include those that derive from the follicular epithelial cells (papillary and follicular), which account for the majority of thyroid cancers, and from the parafollicular C cells (medullary) (2). Other thyroid tumors, including primary lymphomas of the thyroid, which are usually metastases from other primary sites, are also encountered, although rarely.
EVALUATION OF SOLITARY THYROID NODULES
Thyroid cancer usually presents as a nodule identified on physical examination or discovered incidentally on imaging studies performed for unrelated reasons. However, most thyroid nodules are benign, with about 10% to 15% found to be malignant on biopsy (3). The main diagnostic challenge is accurately differentiating benign from malignant disease in order to ensure appropriate definitive therapy and avoid unnecessary treatments.
Clinically palpable nodules are found in approximately 5% of the population (4). Benign and malignant nodules are almost always clinically indistinguishable. Features indicating increased likelihood of carcinoma are summarized in Table 43-2. Initial evaluation and management of patients presenting with thyroid nodules is detailed in Fig. 43-1.
Age <20 years |
Presence of cervical lymphadenopathy |
History of radiation to the head and neck during childhood |
Family history of medullary thyroid cancer or MEN types 2A and 2B |
Hard fixed nodule |
Recent nodule growth |
Hoarseness of voice (indicating invasion of recurrent laryngeal nerve) |
Thyroid ultrasonography with fine-needle aspiration (FNA) and cytologic examination is the modality of choice for evaluating nodules with suspicious characteristics (5). Papillary, medullary, and anaplastic carcinomas can be readily diagnosed by FNA or biopsy, but distinguishing benign from malignant follicular lesions proves more difficult. Histologic examination showing capsular or vascular invasion is necessary to classify a lesion as malignant. Because follicular adenoma and carcinoma cannot be differentiated cytologically, they are grouped as “indeterminate or suspicious follicular neoplasms.” The rate of carcinoma for suspicious follicular neoplasms is about 20%. The incidence of malignancy increases with larger nodule size, male sex, and increasing age. Testing for molecular markers should be considered for patients with indeterminate or suspicious follicular neoplasm on cytology. Fifteen to 25% of the time, the FNA will yield “inadequate diagnostic material,” and this necessitates repeat aspiration. The majority (85%-95%) of thyroid nodules are benign. Radionuclide scans usually show malignant lesions as hypofunctioning or “cold,” although 85% of “cold” nodules are still benign.
DIFFERENTIATED THYROID CANCER
Differentiated thyroid cancer (DTC) includes papillary, follicular, and poorly differentiated histology types and composes about 90% of all thyroid cancers (6). The factors associated with increased risk of thyroid carcinoma are summarized in Table 43-3.
Exposure to ionizing radiation, especially during childhood |
Familial adenomatous polyposis (Gardner syndrome) |
Carney complex |
Werner syndrome |
Cowden syndrome (phosphatase and tensin homolog [PTEN]-hamartoma tumor syndrome) |
Pendred syndrome |
Familial papillary thyroid cancer |
Traditionally, exposure to ionizing radiation, family history, and genetic syndromes have been the main risks associated with DTC (7,8). However, a recent meta-analysis of 21 observational studies suggests an association with obesity (adjusted relative risk [RR], 1.33; 95% confidence interval [CI], 1.24-1.42) (9). On average, thyroid tumors are recognized ten years following radiation exposure, but can be seen as long as 30 years. Malignancy occurs in up to 30% of cases with head or neck irradiation. Exposure to external sources of radiation after the Chernobyl nuclear accident led to a 3- to 75-fold increase in the incidence of papillary thyroid carcinoma (PTC) in fallout regions, especially in younger children (7). Familial PTC is reported in 5% of all patients with PTC and may portend a more aggressive disease course (9a). Additionally, DTC has also been associated with other tumors, particularly breast and renal cancer (10).
Understanding the follicular cell tumorigenesis pathways is central to the development of novel therapies to treat thyroid cancer. The MAPK pathway is the main oncogenic propagator of PTC. BRAF and Ras genes in this pathway normally code for growth and function in normal and tumor cells. Chromosomal rearrangements of the gene encoding the transmembrane tyrosine kinase receptors ret and trk have been implicated as an early step in the development of these tumors. Mutations in either BRAF (40%-60%) or Ras (10%-15%) or RET/PTC rearrangements (10%-15%) are present in most DTCs (11,12) (Fig. 43-2)
Activating ret mutations may be the result of ionizing radiation and were the most common mutations found in the Chernobyl radiation-induced thyroid carcinomas (13,14). BRAF mutation has been implicated in more aggressive disease and greater mortality from PTC (15). In addition, when present in combination with BRAF, a recent novel driver, telomerase reverse transcriptase (TERT) mutation, which is implicated in advanced thyroid cancer, results in the most aggressive PTC with the highest recurrence rate (16,17). Of follicular thyroid cancers, 30% are driven by Pax8-peroxisome proliferator-activating receptor (PPAR)-γ rearrangements, and 10% to 15% are driven by Ras mutations (12).
Thyroid tumors are dependent upon angiogenesis, which is important for tumor cell growth, promotion and development of metastases (18). Vascular endothelial growth factor (VEGF), an important proangiogenic factor, binds to VEGF receptors. This then activates MAP-kinase signalling and promotes further tumor growth. Vascular endothelial growth factor receptors play a contributory role in the development and progression of thyroid cancer (11).
Thyroid cancer is subdivided into well-differentiated neoplasms characterized by slow growth and high curability and a small group of poorly differentiated tumors with poor outcome. There are four major sub-types of thyroid cancer which are based on morphology and biological behavior. This classification is advantageous as it relates morphology to methods of treatment and prognosis.
Histologic characteristics of conventional PTCs include numerous papillae lined by cuboidal to low columnar follicular cells with enlarged irregular nuclei with longitudinal grooves, intranuclear cytoplasmic pseudoinclusions, and solitary or multiple marginally placed micronucleoli (19) (Fig. 43-3). Follicular variant of PTC and other PTC variants are also recognized. Follicular tumors, although frequently encapsulated, can exhibit vascular and capsular invasion microscopically; it is this invasion that, when identified histopathologically, distinguishes benign neoplasms from malignant follicular neoplasms (Fig. 43-4). Hürthle cell carcinomas are considered a type of follicular cancer. Tall cell variants, columnar cell variants, Hürthle cell variant, and insular type are histologic subtypes that may portend a worse prognosis.
The TNM (tumor, node, metastasis) method may be most useful for prediction of disease-free survival and is generally used in our institution. Tumor size and presence of extrathyroidal invasion carry prognostic importance and thus should consistently be included in the pathologist’s synoptic report. Anaplastic thyroid carcinoma by convention is always stage IV (Tables 43-4 and 43-5).
Primary Tumor (T) | |
Note: all categories may be subdivided into (a) solitary tumor and (b) multifocal tumor (the largest determines the classification). | |
TX | Primary tumor cannot be assessed |
T0 | No evidence of primary tumor |
T1 | Tumor 2 cm or less in greatest dimension limited to the thyroid |
T2 | Tumor more than 2 cm but not more than 4 cm in greatest dimension limited to the thyroid |
T3 | Tumor more than 4 cm in greatest dimension limited to the thyroid or any tumor with minimal extrathyroid extension (eg, extension to sternothyroid muscle or perithyroid soft tissues) |
T4a | Tumor of any size extending beyond the thyroid capsule to invade subcutaneous soft tissues, larynx, trachea, esophagus, or recurrent laryngeal nerve |
T4b | Tumor invades prevertebral fascia or encases carotid artery or mediastinal vessels |
All anaplastic carcinomas are considered T4 tumors | |
T4a | Intrathyroidal anaplastic carcinoma—surgically resectable |
T4b | Extrathyroidal anaplastic carcinoma—surgically unresectable |
Regional Lymph Nodes (N) | |
Regional lymph nodes are those of the central compartment as well as lateral cervical and upper mediastinal lymph nodes. | |
NX | Regional lymph nodes cannot be assessed |
N0 | No regional lymph node metastasis |
N1 | Regional lymph node metastasis |
N1a | Metastasis to level VI (pretracheal, paratracheal, and prelaryngeal/Delphian lymph nodes) |
N1b | Metastasis to unilateral, bilateral, or contralateral cervical or superior mediastinal lymph nodes |
Distant Metastasis (M) | |
MX | Distant metastasis cannot be assessed |
M0 | No distant metastasis |
M1 | Distant metastasis |
Separate stage groupings are recommended for papillary or follicular, medullary, and anaplastic (undifferentiated) carcinomas. | |||
Papillary or Follicular (<45 Years) | |||
Stage I | Any T | Any N | M0 |
Stage II | Any T | Any N | M1 |
Papillary or Follicular (≥45 Years) | |||
Stage I | T1 | N0 | M0 |
Stage II | T2 | N0 | M0 |
Stage III | T3 | N0 | M0 |
T1/T2/T3 | N1a | M0 | |
Stage IVA | T4a | N0/N1a | M0 |
T1/T2/T3/T4a | N1b | M0 | |
Stage IVB | T4b | Any N | M0 |
Stage IVC | Any T | Any M | M1 |
Medullary Carcinoma | |||
Stage I | T1 | N0 | M0 |
Stage II | T2 | N0 | M0 |
Stage III | T3 | N0 | M0 |
T1/T2/T3 | N1a | M0 | |
Stage IVA | T4a | N0/N1a | M0 |
T1/T2/T3/T4a | N1b | M0 | |
Stage IVB | T4b | Any N | M0 |
Stage IVC | Any T | Any N | M1 |
Anaplastic Carcinoma | |||
All anaplastic carcinomas are stage IV. | |||
Stage IVA | T4a | Any N | M0 |
Stage IVB | T4b | Any N | M0 |
Stage IVC | Any T | Any N | M1 |
Preoperative ultrasound of the entire neck (not just of the thyroid) is indicated to help identify the presence of nodal metastases and help the surgeon to perform the appropriate surgery. Total thyroidectomy is the preferred initial surgical procedure for most patients with DTC at our institution (Fig. 43-5). Arguments for total thyroidectomy rather than lobectomy are that: (1) papillary foci are seen in bilateral lobes in 60% to 85% of patients (20) and (2) 5% to 10% of recurrences of PTC after a unilateral lobectomy arise in the contralateral lobe (21). A third reason that supports total thyroidectomy is that treatment with radioiodine and the specificity of serum thyroglobulin (Tg) concentrations as a tumor marker become most efficacious.
FIGURE 43-5
Initial approach to management of differentiated thyroid cancer. CT, computed tomography; FNA, fine-needle aspiration; FTC, follicular thyroid carcinoma; HCC, Hürthle cell carcinoma; PTC, papillary thyroid carcinoma; Tg, thyroglobulin. Data from Tuttle RM, Sabra MM. Selective use of RAI for ablation and adjuvant therapy after total thyroidectomy for differentiated thyroid cancer: a practical approach to clinical decision making. Oral Oncol. 2013;49(7):676-683.
Consideration for lobectomy is reasonable in patients with no or low risk with localized small tumors (5). Neck dissection should be performed on patients with identifiable nodal disease, because their presence impacts recurrence. Calcium and parathyroid hormone levels should be monitored postoperatively due to the risk of hypoparathyroidism from either vascular damage intraoperatively or inadvertent removal.
Iodine-131 (131I) is used as adjuvant therapy for thyroid carcinoma; iodine is preferentially taken up and trapped by the thyroid follicular cells and malignant counterparts. Iodine-131 destroys cells of follicular origin by first becoming concentrated in the cell where β rays are released, and the high-energy electrons spewed induce radiation cytotoxicity; simultaneously, γ rays are released that allow for detection of the emission by a camera. Therefore, postoperative examination with radioiodine scanning allows the identification of residual regional or distant foci of disease, and radioiodine can be used therapeutically to ablate such tumor deposits. The rationale for using 131I as adjuvant therapy is as follows: (1) it destroys any residual microscopic foci of disease, (2) it increases specificity of subsequent 131I scanning for detection of recurrent or metastatic disease by elimination of uptake by residual normal tissue, and (3) it improves the value of measurements of serum Tg as a serum marker; hence, any elevation in Tg would be representative of recurrent or metastatic disease and not residual normal thyroid tissue (5,6). Combined retrospective data suggest that radioiodine ablation reduces long-term, disease-specific mortality in patients with primary tumors >4 cm in diameter or larger, those with multicentric disease, or those in whom there is evidence of soft tissue invasion at presentation (22,23). Low-risk patients (Fig. 43-5) may not benefit from radioiodine, and selective use is advocated in these patients. The use of postoperative Tg measured 1 to 2 months after surgery is a useful tool to gauge residual or metastatic disease. Undetectable stimulated or unstimulated Tg indicates very low likelihood of residual disease. Nonstimulated Tg levels <5 ng/mL or stimulated Tg levels <10 ng/mL are also reassuring (24). However, the decision to give 131I should not rely solely on the level of Tg.
Postoperatively, patients who require 131I therapy should be started on liothyronine (synthetic T3) at 25 mg twice daily and discontinued 2 weeks prior to the radioiodine scan. Lower doses are given to elderly patients and patients with coronary artery disease. During this time, patients should avoid foods rich in iodine. Urinary iodine concentrations can be checked to assess total-body iodine content prior to the radioiodine therapy. For maximum radioiodine uptake, the thyroid-stimulating hormone (TSH) should be allowed rise to >30 μIU/mL because this facilitates uptake of iodine by follicular cells. Increased iodide stores decrease radioiodine uptake. For patients in whom a preoperative contrast computed tomography (CT) was done, radioiodine therapy should be avoided for at least 3 months. A pretreatment scan using a 2- to 5-mCi 123I or 131I radioiodine scan for localization of uptake prior to ablation is recommended but not mandatory. Twenty-four to 96 hours after dosing, a whole-body scan showing <5% uptake is presumed to be normal residual tissue. An uptake of more than 5% indicates excessive thyroid tissue and warrants consideration for further surgical resection. The dose of radioactive iodine is determined by extent of residual disease: 30 to 100 mCi for adjuvant ablation, approximately 150 mCi for nodal disease, and 200 mCi or more for metastatic disease outside the lungs (24). A posttreatment scan is performed to assess for further uptake of radioactive iodine that was not previously seen on the pretreatment scan (ie, regional or distant metastases). The posttreatment scan is a more sensitive technique to detect metastatic disease, because the ability to demonstrate radioactive iodine–avid lesions is directly proportional to the amount of radioactive iodine given (Fig. 43-6).
Short-term complications, although rare, include radiation thyroiditis, neck edema, sialoadenitis, and tumor hemorrhage. These occur more often in the presence of bulky disease. Long-term complications, which increase with cumulative doses, include xerostomia, nasolacrimal duct obstruction (25), pulmonary fibrosis (if pulmonary metastasis is present and treated at high doses), and secondary malignancies such as acute myelogenous leukemia (26). There are no reports of congenital abnormalities in children conceived after radioactive iodine treatment; however, most physicians recommend waiting for 6 months before conceiving. Radioactive iodine should not be given to pregnant women due to the potential teratogenic effects for the fetus’s growth and thyroid development; all women of childbearing age must have a negative pregnancy test prior to treatment.
Thyroid hormone replacement targeting TSH suppression has been shown to increase disease-free survival two- to threefold, especially in high-risk patients. It minimizes potential TSH-stimulated growth of thyroid cancer cells. Oversuppression of TSH to undetectable levels can present morbid consequences including osteopenia, atrial fibrillation, and possible cardiac hypertrophy. Moderate TSH suppression (subnormal but not undetectable) during follow-up was associated with better outcomes in all stages, including those with distant metastatic disease (27). For high risk patients, moderate TSH suppression continued for the first 3 years after initial diagnosis may be indicated.
External beam radiotherapy (EBRT) has a limited role in the treatment of PTC. Our institutional review of postoperative conformal EBRT in high-risk DTC patients showed durable locoregional control in those with residual microscopic disease after initial surgery. However, patients with gross residual disease had significantly worse outcomes, and EBRT in these instances should be avoided (28). Guidelines recommend consideration for EBRT in patients >45 years old with gross non–iodine-avid macroscopic disease in whom further surgery would not be beneficial (5).
Following initial therapy, patients with DTC need lifelong monitoring using both clinical and radiographic data. In high-risk patients, follow-up radioiodine scan 12 months after initial radioiodine ablation should be considered for reassessment of disease burden. The TSH should be allowed to increase to a value >30 μIU/mL by either withdrawal of thyroid hormone or using recombinant human TSH (rhTSH). Thyrotropin alfa, an rhTSH, administered as two injections on 2 consecutive days may be used in lieu of standard thyroid hormone withdrawal to increase thyrotropin concentrations and for adequate stimulation of both radioiodine uptake for scanning and serum Tg concentrations. The use of rhTSH is of particular benefit in the patient in whom endogenous TSH levels cannot rise due to hypopituitarism or in whom the clinician prefers to avoid prolonged hypothyroidism and its resultant complications due to concurrent medical problems. Radioiodine scanning beyond this first follow-up scan needs to be individualized and is no longer routine for all thyroid cancer patients including high-risk patients.
Ultrasonography of the neck (thyroid bed and cervical neck compartments) with FNA of suspicious accessible lesions is used 6 to 12 months postoperatively as part of routine follow-up.
Other non–radioiodine imaging techniques include CT of the neck and chest, chest radiographs, fluorodeoxyglucose (FDG) positron emission tomography (PET) or PET, and magnetic resonance imaging (MRI). Magnetic resonance imaging and CT of the neck play important roles in the detection of recurrent disease; they are not as sensitive as ultrasound but are much less operator dependent. Chest CT shows both micronodular and macronodular pulmonary metastases. Fluorodeoxyglucose-PET/CT imaging is useful in patients with a Tg >10 ng/mL who have negative radioiodine imaging but is not routinely used in follow-up of other thyroid cancer patients (5). Patients with DTC with little to no iodine activity generally have higher glucose metabolism and positivity on FDG-PET scans. Lack of iodine uptake denotes tumor dedifferentiation. Although PET is sensitive in detecting metastatic disease, it is not specific for thyroid cancer, and caution should be exercised when evaluating recurrent disease (Fig. 43-7). The use of CT imaging in conjunction with FDG-PET improves the sensitivity for small subcentimeter metastatic disease seen with DTC.
Thyroglobulin is a unique protein synthesized only by the thyroid follicular cells (both benign and differentiated malignant tissue) and, therefore, is a good biochemical test to assess for the presence of residual, recurrent, or metastatic disease. Dedifferentiated tumors lose the ability to secrete Tg, and it cannot be used as a tumor marker. Following total thyroidectomy with or without radioiodine ablation, the Tg falls to its nadir usually within 3 months, but may take as long as 2 years. Subsequent Tg measurements (stimulated or unstimulated) are used to monitor for disease recurrence and should be repeated in the same laboratory to avoid erroneous misinterpretations of interassay variability (29). Simultaneously Tg and Tg antibody (TgAb) should always be measured together because TgAb can falsely lower the Tg concentrations in immunometric assays. These antibodies, which are present in approximately 25% of patients, interfere with the assay’s ability to bind to Tg. Thyroglobulin measurements by radioimmunoassay or liquid chromatography tandem mass spectrometry are more resistant to interference by TgAb and are used when antibody presence is detected. The median time to TgAb disappearance after total thyroidectomy and radioiodine ablation is about 3 years (30). Therefore, increases in TgAb after the nadir has been reached should alert the clinician to the possibility of recurrent disease. Although in general the diagnostic accuracy of serum Tg is higher after TSH stimulation than during thyroxine treatment, an unstimulated Tg measured by second-generation assays may be used to follow up patients with stimulated Tg of <2 ng/mL with no evidence of recurrent disease on imaging (31).
Distant metastases are evident in about 15% of patients with DTC. Half of these patients have notable metastases at the time of initial presentation (12). The most common sites of metastasis, in decreasing order of frequency, are the lungs, bones, and other soft tissues (Figs. 43-8A, 43-8B, and 43-9). Older patients have a higher risk for distant metastases. For resectable locoregional and isolated metastasis, surgery is preferred.
In those with iodine-avid unresectable disease or distant metastasis, 131I treatment should be recommended, and higher doses (150-200 mCi) are typically used. Although this treatment may be repeated 6 to 12 months later, caution and careful monitoring for side effects should be exercised. Moderate TSH suppression should also be continued in these patients. Metastases at different sites require management by multimodality approaches. Bone lesions may be surgically resected or treated with 131I treatment or EBRT (32). In follicular thyroid carcinoma, where the lesions are highly vascular, arterial embolization has been used anecdotally with successful reduction in pain at our institution. In addition, intravenous bisphosphonates (pamidronate or zoledronic acid) and denosumab are prescribed for painful bony metastases with some success. Experience at our institution also showed improved median survival for patients with one or more brain metastases after surgical resection. Stereotactic radiosurgery is also an option. We suggest a multidisciplinary approach and discussion with the surgeon, radiation oncologist, and endocrinologist about the risks and benefits of each modality in the management of metastatic disease.
In patients with progressive metastatic disease that does not respond to standard therapy, long-term overall survival is <10%. For this subset of patients, the understanding of the molecular model for thyroid cancer and the discovery of targeted therapies have led to a new era of treatment (12). Consequently, over the last 5 years, two tyrosine kinase inhibitors (TKIs), sorafenib (2013) and lenvatinib (2015), have been approved by the US Food and Drug Administration (FDA) for the treatment of locally recurrent or metastatic, progressive DTC refractory to radioactive iodine treatment. Previously recommended cytotoxic therapies with agents such as doxorubicin have had poor outcomes with significant toxicities (12). The TKIs target intercellular signaling pathways (MAPK pathway in the tumor cells) but also play a role in the surrounding tumor vasculature (12). Trials evaluating the efficacy of the approved drugs have shown better progression-free survival (PFS) than in the placebo groups (5 months with sorafenib and 14.7 months with lenvatinib) (33,34). The recently approved TKI lenvatinib had an overall response rate of 64.8% versus 1.5% in the placebo group (odds ratio, 28.87; 95% CI, 12.46-66.86). However, it is important to note the deleterious effects of these drugs, most commonly hypertension, gastrointestinal effects such as diarrhea, altered taste and stomatitis, palmar-plantar erythrodysesthesia syndrome (hand and foot syndrome), and weight loss.
Indications for therapy include patients with progressive or symptomatic unresectable disease that is refractory to 131I treatment. Patients with asymptomatic stable or very slowly progressive disease on thyroid hormone suppression may be closely monitored. Prior to initiation of therapy, a comprehensive evaluation to ascertain appropriate selection of patients should be conducted. This should include a candid discussion with the patient and family about the benefits and risks of these agents. Furthermore due to the life-threatening adverse effects of these drugs, only clinicians who are well versed with use of these agents should initiate and monitor these patients. We have previously published an adverse event monitoring tool used at our institution to guide clinicians initiating patients on these agents (35).
As in adults, PTC followed by follicular thyroid cancer occur most frequently in children. Exposure to radiation is the major risk factor. It is not unusual for children with PTC to present with multifocal disease with lymph node involvement, extrathyroidal extension, and pulmonary metastasis (36). Despite this, children overall have high cure rates, with 10-year survival of almost 100%. Those diagnosed before the age of 10 years, however, seem to have a higher risk of recurrence and death. The treatment of thyroid cancer in children mirrors that of adults. Total thyroidectomy (rarely lobectomy) with nodal dissection done at a high-volume center due to higher complication rates is recommended (36). Postoperative universal radioiodine therapy is no longer advocated, and careful selection based on risk of recurrence, residual or distant disease remaining after surgery, and Tg levels is used to determine the need for therapy (36). Moderate TSH suppression (0.1-0.5 μIU/mL) with relaxation when there is no evidence of recurrent disease is suggested. Lifelong surveillance is essential in monitoring children for recurrent disease because recurrence may occur 20 to 30 years later. For the few children with progressive radioiodine-refractory DTC, referral to a center with pediatric endocrine neoplasia expertise for consideration for systemic therapy is recommended.
MEDULLARY THYROID CARCINOMA
Medullary thyroid carcinomas (MTCs) are derived from C cells (calcitonin-secreting cells) that are part of neural crest origin (see Table 43-1). The majority—80% of patients with MTC have sporadic disease. The other 20% have an inherited form that occurs as an autosomal dominant trait as part of the multiple endocrine neoplasia (MEN) clinical syndromes, MEN type 2A or type 2B or familial MTC (37). In MEN 2A, MTC occurs in association with pheochromocytoma and multigland parathyroid tumors; MTC is usually the first manifested disease of the three components of this syndrome. In MEN 2B, MTC occurs in association with pheochromocytoma and mucosal neuromas (Figs. 43-10A and 43-10B) or neurofibromas and marfanoid habitus. Familial MTC is a variant of MEN 2A where only MTC is clinically evident (38). Most patients with sporadic MTC present in the fifth or sixth decade of life with a male-to-female ratio of 1.4:1.
The most common clinical presentation of sporadic MTC is a solitary thyroid mass found incidentally during routine examination. Other symptoms such as secretory diarrhea and facial flushing may also be seen with hormone overproduction. Fine-needle aspiration should be performed for suspicious nodules, and once MTC is suspected, calcium, calcitonin, and carcinoembryonic antigen (CEA) levels should be obtained, with screening for pheochromocytoma and a thorough history and physical examination also performed. Because about 6% of patients with sporadic MTC carry a germline RET mutation, genetic counseling and testing are offered to all patients with newly diagnosed apparent sporadic disease (39). If pheochromocytoma is confirmed, appropriate control of catecholamine hypersecretion and removal should precede thyroid surgery.
Medullary thyroid cancer occurs as a solid mass or cluster of C-cell hyperplasia interspersed between normal thyroid follicles and can be visualized with calcitonin immunostaining. (Fig. 43-11). It appears as variable amounts of fibrosis as well as deposits of amyloid in 60% to 80% of tumors. Notably, even the smallest visible tumors can be associated with metastases.
Metastases to cervical and mediastinal lymph nodes are found in about 50% of the patients at the time of initial presentation. Distant metastases to the lungs, liver, bones, and adrenal glands most commonly occur late in the course of the disease (Fig. 43-12).
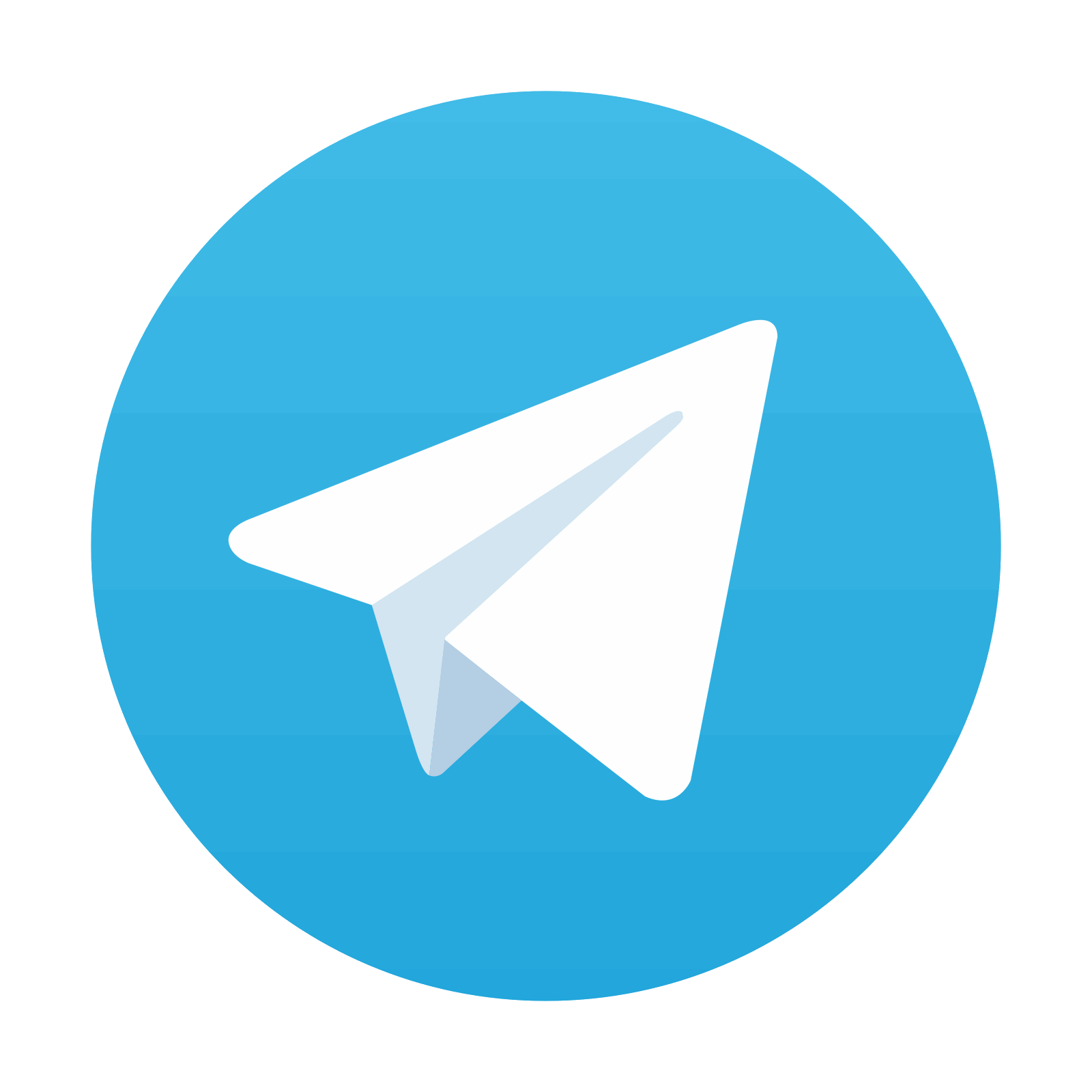
Stay updated, free articles. Join our Telegram channel

Full access? Get Clinical Tree
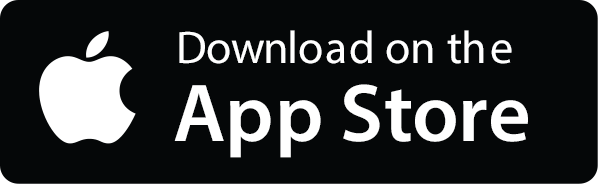
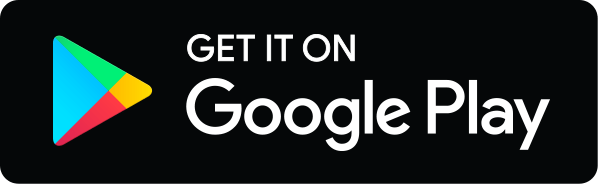