EPIDEMIOLOGY
Breast cancer is the second most common cause of death for women and is the most common cause of death for women age 45 to 55. In 2015, it is estimated that 231,840 American women would be diagnosed with breast cancer and that 40,290 would die from this disease, making breast cancer the second most common cause of cancer-related morality in the United States, with lung cancer being the most common (1).
In the early 1980s, the rates of breast cancer diagnosis rose sharply, likely related to increased mammographic screening, because it was the incidence of stage 0 and I carcinomas that rose most sharply. Data from the Surveillance, Epidemiology, and End Results (SEER) program of the National Cancer Institute demonstrate that although the incidence of breast cancer has been stable since the late 1980s, there has been an increase in the percentage of breast cancers that are hormone receptor positive, which is thought to be due either changes in receptor assays or an increased use of hormone replacement therapy by women (2,3). The incidence of primary breast cancer then decreased around 2003, shortly after the publication of the Women’s Health Initiative (WHI) results, which prompted many healthy postmenopausal women to stop using hormone replacement therapy (4).
Breast cancer incidence has long varied in different regions of the world. Incidence is highest in Northern Europe and North America and lowest in Asia and Africa. Data suggest that this variability is due not only to environmental factors but also to lifestyle. This is supported by the observation that breast cancer incidence is higher in second-generation Asian immigrants in the United States (5).
Breast cancer overall mortality rates had been stable for more than 50 years prior to 1989. Starting in the 1990s, there has been a steady decrease in breast cancer deaths every year. Mortality rates declined by 1.4% per year from 1989 to 1995 and by 3.2% per year thereafter. This is thought to be due in part to increased use of mammography, resulting in earlier diagnosis, and the use of effective treatments. Mortality rates continue to be higher for African American women. This is due in part to disparities in health care access that exist both for diagnosis as well as treatment (6).
RISK FACTORS
Although it is known that family history is an important risk factor for breast cancer, only 25% of newly diagnosed patients have a positive family history. The Gail model was the first to incorporate the number of first-degree relatives into a comprehensive model of breast cancer risk assessment (7). Claus then assessed the estimated risk of breast cancer based on the number of familial cases and their ages of diagnosis (8). It is now well known that the risk for each patient with a positive family history is affected by the age of the family member at diagnosis, the total number of first-degree relatives affected, and the patient’s age. Based on data from a large meta-analysis, the risk of breast cancer for a patient with one affected first-degree relative increased 1.80-fold; if there were two affected first-degree relatives, that risk increased 2.93-fold. This risk was then further modified by the age of the patient, such that a woman’s risk of breast cancer prior to age 40 was increased to 5.7-fold if one relative was diagnosed prior to age 40 (9).
The overall prevalence of specific genetic mutations accounting for breast cancer is rare, accounting for only 5% to 10% of all cases. Risk can be further subdivided based on a patient’s history. The most commonly studied mutations are on the BRCA1 and BRCA2 genes, although multiple other mutations exist on genes such as p53, ATM, CHEK2, PTEN, MLH1, MSH2, and PALB2 (10). In a study that analyzed 10,000 individuals, excluding those with Ashkenazi ancestry, the prevalence of BRCA1 and BRCA2 mutations varied, with a low of 2.9% if the patient and all first- or second-degree relatives had no prior history of breast cancer or ovarian cancer at less than 50 years of age. A maximum prevalence of 81.3% was noted if the patient and any first- or second-degree relative had breast cancer diagnosed at less than 50 years of age and ovarian cancer at any age (11). Because genetic testing often leads to complicated medical decisions both for the patient and other family members, it is important to determine whom it is most appropriate to screen by taking into account population-dependent positive and negative predictive values of the test, using statistical models.
There has been a rapid increase in the literature concerning the epidemiology, natural history, and treatment of ductal carcinoma in situ (DCIS) and lobular carcinoma in situ (LCIS). (See detailed information in Chapter 30).
With DCIS, the 10-year risk of invasive breast cancer is 5% in the contralateral breast. Lobular carcinoma in situ has been regarded as a risk factor for ipsilateral and contralateral breast cancer. Recent research supports that LCIS is a direct precursor of both invasive lobular and ductal carcinoma. For patients diagnosed with LCIS, the risk of developing breast cancer in either breast is 1% a year (12).
A later age of menarche is protective. One study has reported that for every 2-year delay in menarche, there was a 10% reduction in breast cancer risk (13).
There is a favorable risk reduction associated with earlier age of pregnancy. Women who give birth for the first time at age 35 have a 1.6-fold higher risk of breast cancer compared with women who were 26 to 27 at time of first birth. Women who are over age 30 at the time of first birth are at higher risk than nulliparous women (14).
Late menopause is associated with a higher risk of breast cancer. Oophorectomy before age 40 will decrease the lifetime risk of breast cancer by 50% (15).
Breast cancer is the second most common cancer associated with pregnancy, with its incidence being 1 in 3,000. The incidence of pregnancy-associated breast cancer is likely related to the delay of childbirth until after age 30. There are controversial data from two reports suggesting that pregnancy might cause a transient rise in breast cancer risk. However, a clearly documented decreased risk of breast cancer occurs 10 to 15 years after childbirth (16). (See detailed information in Chapter 30.)
Most studies have not shown an increased risk of breast cancer with oral contraceptive use (17,18). However, a meta-analysis showed a significant but small increase in relative risk of breast cancer (19). A concern about the meta-analysis is that follow-up was limited.
The WHI showed that the relative risk of breast cancer was increased to 1.26 for women who took combined treatment with estrogen and progesterone for a mean of 5.2 years as compared to placebo (20). Although long-term hormone replacement therapy was associated with a higher risk of breast cancer, short-term use did not seem to significantly increase the risk of breast cancer.
STAGING OF BREAST CANCER
The 2010 seventh edition of the Cancer Staging Manual published by the American Joint Committee on Cancer (AJCC) was modified from the prior staging criteria, published in 2002 (Tables 27-1 and 27-2) (21).
Primary Tumor (T) | |
---|---|
TX | Primary tumor cannot be assessed |
T0 | No evidence of primary tumor |
Tis | Carcinoma in situ |
Tis (DCIS) | Ductal carcinoma in situ |
Tis (LCIS) | Lobular carcinoma in situ |
Tis (Paget) | Paget disease of the nipple with no tumor |
Note: Paget disease associated with a tumor is classified according to the size of the tumor. | |
T1 | Tumor ≤20 mm in greatest dimension |
T1mi | Microinvasion ≤1 mm in greatest dimension |
T1a | Tumor >1 mm but ≤5 mm in greatest dimension |
T1b | Tumor >5 mm but ≤10 mm in greatest dimension |
T1c | Tumor >10 mm but ≤20 mm in greatest dimension |
T2 | Tumor >20 mm but ≤50 mm in greatest dimension |
T3 | Tumor >50 mm in greatest dimension |
T4 | Tumor of any size with direct extension to |
(a) chest wall or | |
(b) skin, only as described below | |
T4a | Extension to chest wall, not including pectoralis muscle adherence/invasion |
T4b | Ulceration and/or ipsilateral satellite nodules and/or edema (including peau d’orange) of the skin, which do not meet the criteria for inflammatory carcinoma |
T4c | Both T4a and T4b |
T4d | Inflammatory carcinoma with typical skin changes involving a third or more of the skin of the breast |
Regional Lymph Nodes (N) | |
NX | Regional lymph nodes cannot be assessed (eg, previously removed) |
N0 | No regional lymph node metastases |
N1 | Metastases in movable ipsilateral axillary level I, II axillary lymph node(s) |
N2 | Metastases in ipsilateral level I, II axillary lymph nodes that are clinically fixed or matted; or in clinically detected ipsilateral internal mammary nodes in the absence of clinically evident axillary lymph node metastases |
N2a | Metastases in ipsilateral level I, II axillary lymph nodes fixed to one another (matted) or to other structures |
N2b | Metastases only in clinically detected ipsilateral internal mammary nodes and in the absence of clinically evident level I, II axillary lymph node metastases |
N3 | Metastases in ipsilateral infraclavicular (level III axillary) lymph node(s) with or without level I, II axillary lymph node involvement; or in clinically detecteda ipsilateral internal mammary lymph node(s) with clinically evident level I, II axillary lymph node metastases; or metastases in ipsilateral supraclavicular lymph node(s) with or without axillary or internal mammary lymph node involvement |
N3a | Metastases in ipsilateral infraclavicular lymph node(s) |
N3b | Metastases in ipsilateral internal mammary lymph node(s) and axillary lymph node(s) |
N3c | Metastases in ipsilateral supraclavicular lymph node(s) |
Regional Lymph Nodes (pN) | |
pNX | Regional lymph nodes cannot be assessed (eg, previously removed or not removed for pathologic study) |
pN0 | No regional lymph node metastases histologically Note: Isolated tumor cell clusters (ITC) are defined as small clusters of cells not greater than 0.2 mm, or single tumor cells, or a cluster of fewer than 200 cells in a single histologic cross-section. ITCs may be detected by routine histology or by IHC methods. Nodes containing only ITCs are excluded from the total positive node count for purposes of N classification but should be included in the total number of nodes evaluate |
pN0(i–) | No regional lymph node metastases histologically, negative IHC |
pN0(i+) | Malignant cells in regional lymph node(s) no greater than 0.2 mm (detected by H&E or IHC including ITC) |
pN0(mol–) | No regional lymph node metastases histologically, negative molecular findings (RT-PCR) |
pN0(mol+) | No regional lymph node metastases histologically, positive molecular findings (RT-PCR) |
pN1 | Micrometastases; or metastases in 1–3 axillary lymph nodes; and/or in internal mammary nodes with metastases detected by sentinel lymph node biopsy but not clinically detected |
pN1mi | Micrometastases (>0.2 mm and/or >200 cells, but none >2.0 mm) |
pN1a | Metastases in 1–3 axillary lymph nodes, at least one metastasis greater than 2.0 mm |
pN1b | Metastases in internal mammary nodes with micrometastases or macrometastases detected by sentinel lymph node biopsy but not clinically detecteda |
pN1c | Metastases in 1–3 axillary lymph nodes and in internal mammary lymph nodes with micrometastases or macrometastases detected by sentinel lymph node biopsy but not clinically detected |
pN2 | Metastases in 4–9 axillary lymph nodes; or in clinically detectedb internal mammary lymph nodes in the absence of axillary lymph node metastases |
pN2a | Metastases in 4–9 axillary lymph nodes (at least one tumor deposit >2.0 mm) |
pN2b | Metastases in clinically detectedb internal mammary lymph nodes in the absence of axillary lymph node metastases |
pN3 | Metastases in 10 or more axillary lymph nodes; or in infraclavicular (level III axillary) lymph nodes; or in clinically detected ipsilateral internal mammary lymph nodes in the presence of one or more positive level I, II axillary lymph nodes; or in more than three axillary lymph nodes and in internal mammary lymph nodes with micrometastases or macrometastases detected by sentinel lymph node biopsy but not clinically detected, or in ipsilateral supraclavicular lymph nodes |
pN3a | Metastases in 10 or more axillary lymph nodes (at least one tumor deposit >2.0 mm); or metastases to the infraclavicular (level III axillary lymph) nodes |
pN3b | Metastases in clinically detectedb ipsilateral internal mammary lymph nodes in the presence of one or more positive axillary lymph nodes; or in more than three axillary lymph nodes and in internal mammary lymph nodes with micrometastases or macrometastases detected by sentinel lymph node biopsy but not clinically detected |
pN3c | Metastases in ipsilateral supraclavicular lymph nodes |
Distant Metastases (M) | |
M0 | No clinical or radiographic evidence of distant metastases |
cM0(i+) | No clinical or radiographic evidence of distant metastases, but deposits of molecularly or microscopically detected tumor cells in circulating blood, bone marrow, or other nonregional nodal tissue that are no larger than 0.2 mm in a patient without symptoms or signs of metastases |
M1 | Distant detectable metastases as determined by classic clinical and radiographic means and/or histologically proven larger than 0.2 mm |
The changes were based on continuing developments in breast cancer diagnosis and management. Specifically, isolated tumor cells and micrometastases in axillary lymph node staging and M0(i+) for tumor cells in circulation and bone marrow were added. The AJCC also recommended that all specimens have a histologic tumor grade and description of estrogen receptor (ER), progesterone receptor (PR), and human epidermal growth factor receptor 2 (HER2) status.
PROGNOSTIC FACTORS
There is interest in the assessment of prognostic factors in breast cancer. About 30% of patients with node-negative disease will die from a breast cancer–related cause. Thus, there is a great thrust of research to determine markers that could further identify which patients would benefit most from available adjuvant treatment.
With the growing array of articles in this field, it is important to distinguish predictive prognostic factors. A predictive factor is one that can provide information on the likelihood of response to a given therapeutic intervention. A prognostic factor is one that can provide information on outcome at the time of diagnosis, independent of treatment (22). Lymph node status is an example of a prognostic factor; ER status is an example of a prognostic and predictive factor.
Prognosis is still determined in large part by histopathology. Multiple studies showed that the most powerful prognostic factor is the extent of disease in the axillary lymph nodes (23,24). Other important pathologic factors are hormone receptor status, HER2 status, histologic grade, tumor type, and lymphovascular invasion.
More than 30 years ago, it was established that the number of involved lymph nodes could be used to predict disease-free survival (DFS) and overall survival (OS). The 5-year DFS was 62% with 1 to 3 positive axillary lymph nodes, 58% for 4 to 9 positive lymph nodes, and 29% for ≥10 positive lymph nodes (24).
Nuclear or histologic grade describes the degree of tumor differentiation and is based on a pathologist’s assessment of nuclear size and shape, number of mitoses, and degree of tubule formation. Although a nuclear grade of 1 (most differentiated) to 3 (least differentiated) is reported with every breast cancer pathology report, its use in predicting outcome is still debated (25). This is in part secondary to interobserver variation in the classification of differentiation. The Nottingham combined grading system seems to be most useful because of its semiquantitative approach and is currently recommended by the College of American Pathologists.
Estrogen receptor and PR positivity correlate with prolonged DFS and OS. The importance of hormone receptor status has been documented more consistently in node-positive than in node-negative disease. Immunohistochemical assays have now become the favored approach because they can be used with a variety of specimens. A “positive” specimen is defined as at least 1% of positive cells (26).
This can be evaluated by a variety of methods including mitotic figure count, S-phase fraction (the fraction of cells synthesizing DNA) as determined by flow cytometry, thymidine labeling index, and monoclonal antibodies to antigens in proliferating cells. A high S-phase fraction is usually associated with poor differentiation and lack of ER positivity. Antibodies to Ki-67 can be used to determine a proliferative rate that corresponds with the S-phase fraction. A recent meta-analysis showed a positive correlation between high Ki-67 and poor prognosis (27).
The HER2/neu oncogene codes for a 185-kDa transmembrane glycoprotein that has intracellular tyrosine kinase activity and is a member of the family of epidermal growth factor receptors. This group of receptors has an important role in the activation of epidermal signal transduction pathways controlling for epithelial growth and differentiation. Overexpression of the HER2/neu oncogene is present in up to 30% of invasive breast cancers.
The current standard is to perform either fluorescence in situ hybridization (FISH) by single or dual probe or immunohistochemistry (IHC). Breast cancer is defined as HER2-positive overexpressed if IHC is noted to be 3+, which is defined as >10% of membrane staining. By FISH, for single probe, the average copy number is ≥6.0 signals per cell. For dual probe, the HER2/CEP17 ratio should be ≥2.0 or if HER2 copy number is ≥6.0 signals/cell regardless of HER2/CEP17 ratio. Equivocal results include IHC 2+ or dual probe FISH HER2/CEP17 ratio <2.0 and an HER2 copy number between 4.0 and 6.0 signals/cell. These results should be confirmed with the other approved diagnostic tests. If results continue to be equivocal, repeat testing or a new biopsy should be considered (28).
Prior to the introduction of HER2-targeted therapy, overexpression of HER2/neu was associated with shorter DFS and OS (29). A large single-institution study reviewed all women with T1a and T1b disease diagnosed between 1990 and 2002. Multivariate analysis of 965 patients showed that patients with HER2-positive tumors had 5.09 times the rate of recurrence and 7.81 times the rate of distant recurrence at 5 years compared to patients with hormone receptor–positive, HER2-negative tumors (30). The predictive response with HER2 has been demonstrated in prospective randomized controlled trials (discussed later in the “Adjuvant Therapy” section) (31,32).
DNA microarrays classify breast cancer into five major subtypes defined as luminal A, luminal B, basal, HER2 positive, and normal-appearing breast tissue. In a retrospective analysis, these subtypes were associated with differing prognoses (33). At present, microarrays for these classifications are too expensive to be performed routinely. Cheaper and more cost-effective diagnostics may change the approach.
ADJUVANT THERAPY
After definitive local therapy is completed, it is important to plan for adjuvant systemic therapy. The use of chemotherapy, hormonal therapy, and targeted therapy before or after definitive local therapy has had a significant effect on the management and outcomes of breast cancers. All women with node-positive disease and a significant percentage of node-negative women with tumors that are hormone receptor negative or >1 cm in size benefit from chemotherapy. The choice of agents to be used for chemotherapy and hormonal therapy should be guided by the patient’s age, concomitant medical issues, positive or negative axillary lymph node involvement, and the status of the hormone receptors and HER2. Estimation of risk of recurrence and death should be assessed. Adjuvant! Online is a validated model that estimates DFS and OS based on age, comorbidity, tumor size and grade, hormone receptor status, and number of involved lymph nodes (34). Additionally, Oncotype Dx, Mammaprint, and other assays can help stratify risk for hormone receptor–positive, node-negative patients (35).
Studies in the 1960s and 1970s evaluated whether single-agent chemotherapy after local therapy had any benefit compared with observation alone. The single agents studied included cyclophosphamide, thiotepa, and melphalan. Most reports documented that single agents have modest or no effect on DFS. Subsequently, the focus shifted to polychemotherapy, with most trials evaluating variations of the three-drug regimen of cyclophosphamide, methotrexate, and 5-fluorouracil (CMF) or similar anthracycline-containing regimens (36,37).
These polychemotherapy regimens clearly showed a greater benefit in DFS and OS, but it was often unclear to clinicians which regimens were superior or equivalent. The Oxford Overview in 1998 helped clarity this issue by reviewing data from about 18,000 women in 47 trials that compared polychemotherapy or no chemotherapy, about 6,000 women in 11 trials that compared longer versus shorter polychemotherapy, and about 6,000 women in 11 trials of anthracycline-containing regimens versus CMF (38). The final interpretation concluded that adjuvant polychemotherapy for patients under 50 years of age resulted in an absolute improvement in 10-year survival of 7% to 11%, whereas the overall 10-year survival benefit was 2% to 3% for patients age 50 to 69 years.
The 1998 Oxford Overview further demonstrated that anthracycline-containing regimens were superior to CMF. There was a statistically significant 12% reduction in risk of recurrence for anthracycline-containing regimens, a 2.7% decrease in mortality, and a 3.2% decrease in relapse. The information gained from this important systematic analysis began a shift toward administration of anthracycline-based regimens for adjuvant therapy of breast cancer.
Official recommendations have been made based on the above data. These were presented at the National Institutes of Health Consensus Development Conference in 2000 and suggested an anthracycline be included as part of breast cancer adjuvant therapy. Several studies have investigated the role of HER2/neu in the positive response to anthracyclines. Both the Cancer and Leukemia Group B (CALGB) 8082 and National Surgical Adjuvant Breast and Bowel Project (NSABP) B-11 studies have shown a benefit in DFS in patients who overexpressed HER2/neu and received anthracycline therapy (39,40).
Evaluation of 5-fluorouracil, doxorubicin, and cyclophosphamide (FAC) began in 1974 at the University of Texas MD Anderson Cancer Center (MDACC). For 1,107 women with node-positive disease, the results were favorable. The 10-year DFS was 72% for patients with 1 to 3 positive nodes, 55% for patients with 4 to 10 positive nodes, and 36% for those with >10 positive nodes (41).
Investigations addressing accelerating the delivery of anthracyclines in a dose-dense manner are discussed later.
The role of taxanes for breast cancer treatment was first investigated in metastatic disease. In randomized trials, paclitaxel and docetaxel improved response rates, duration of response, and OS (42). These positive results prompted their investigation in early-stage breast cancer. Several major studies contributed to the current use of taxanes in the adjuvant setting. The CALGB 9344 study evaluated the addition of paclitaxel to doxorubicin and cyclophosphamide (AC) and showed improvements versus placebo in DFS and OS at 69 months of 70% versus 65% and 80% versus 77%, respectively.
The use of docetaxel was evaluated by the Breast Cancer International Research Group (BCIRG) 001 trial, which compared TAC (docetaxel plus AC) versus FAC for the adjuvant treatment of node-positive breast cancer. There was a significant difference in DFS and a trend in OS suggesting docetaxel could reduce the risk of recurrence of breast cancer in the adjuvant setting compared to the standard FAC regimen. However, with the higher rates of myelosuppression and febrile neutropenia seen with TAC, the use of this regimen requires extensive supportive care, including utilization of granulocyte colony-stimulating growth factor (43,44)
The Eastern Cooperative Oncology Group (ECOG) E1199 trial attempted to define the more effective adjuvant taxane and the optimal schedule of administration (45). All patients received a standard dose and schedule of doxorubicin and cyclophosphamide and were randomized to receive paclitaxel (175 mg/m2) every 3 weeks for four cycles, paclitaxel (80 mg/m2) every week for 12 doses, docetaxel (100 mg/m2) every 3 weeks for four cycles, or docetaxel (35 mg/m2) every week for 12 doses. Weekly paclitaxel compared to every 3 weeks was better, with an odds ratio of 1.27 for DFS (P = .006) and 1.32 for OS (P = .01). No significant difference existed between paclitaxel and docetaxel. Paclitaxel every 3 weeks was no longer recommended after this trial (Fig. 27-1).
FIGURE 27-1
Results of Eastern Cooperative Oncology Group E1199: Optimal adjuvant taxane and optimal schedule of administration. D, docetaxel; DFS, disease-free survival; HR, hazard ratio; OS, overall survival; P, paclitaxel. (Data from Sparano JA, Wang M, Martino S, et al. Weekly paclitaxel in the adjuvant treatment of breast cancer. N Engl J Med. 2008;358:1663-1671.)
The US09735 trial was a randomized study that compared four cycles of standard-dose AC with four cycles of docetaxel (75 mg/m2) and cyclophosphamide (TC) (600 mg/m2). Most patients (84.3%) were younger than 65 years old, and 48% were node negative. At a median follow-up of 7 years, there was a significant difference in DFS between TC and AC (81% vs 75%; hazard ratio, 0.74). Additionally, there was a significant difference in OS (87% vs 82%). Febrile neutropenia in older patients was 8% with TC and 4% with AC (46). This indicates that TC is a treatment option but should be used with caution in higher risk cancers given the large percentage of young node-negative patients in the study.
The correlation between the endocrine system and breast cancer was first recognized more than 100 years ago. Beatson first described bilateral oophorectomy in treating inoperable cases of breast cancer (47). However, the true understanding of the biological mechanisms that cause estrogen to stimulate the growth of hormone receptor–positive tumors is more recent. Jensen first identified the ER and led subsequent cloning of ER and PR. This knowledge has enabled the development of multiple therapies. Many of these therapies have varying mechanisms of action, but all have the common goal of decreasing estrogen availability for hormone receptor–positive malignancies.
In the late 1970s, tamoxifen was shown to be effective for the treatment of metastatic breast cancer. This form of treatment was well received, because data from trials showed that patients experienced fewer side effects than they did with traditional chemotherapy or with old fashioned endocrine therapy (high-dose estrogens, androgens, adrenalectomy, or hypophysectomy). The proven efficacy of tamoxifen in the metastatic setting enabled its study for adjuvant use.
Tamoxifen was the first targeted drug to be used as an endocrine treatment for early breast cancer. An early placebo-controlled trial of tamoxifen as adjuvant therapy for early breast cancer, NATO, showed that 2 years of tamoxifen treatment reduced treatment failure at 21 months compared with control (14.2% vs 20.5%, respectively) (48). Since then, the efficacy of tamoxifen in the adjuvant treatment of primary breast cancer has been demonstrated repeatedly.
Since tamoxifen became available over 35 years ago, a large number of trials investigated its efficacy and tolerability in the treatment of primary breast cancer. Although some individual trials are too small to justify firm conclusions, a meta-analysis has increased confidence in the effectiveness of tamoxifen in improving DFS and OS. Additionally, large cooperative group (NSABP B-14) and international randomized trials (Stockholm and Scottish trials) of tamoxifen versus placebo have demonstrated a clear benefit in DFS and OS (49,50,51).
An overview of 55 trials studying adjuvant tamoxifen for 1, 2, or 5 years versus no treatment in patients with primary breast cancer showed that tamoxifen treatment produced highly significant benefits in terms of both recurrence of first events and mortality in the hormone receptor–positive population. The reductions in recurrence were 21%, 28%, and 50%, and the reductions in death rate were 14%, 18%, and 28% for 1, 2, and 5 years of tamoxifen treatment, respectively (P < .00001 for each). Tamoxifen treatment for 1, 2, and 5 years also reduced the incidence of contralateral breast cancer by 13%, 26%, and 47%, respectively (52). The benefits occurred almost exclusively in the hormone receptor–positive population. Tamoxifen improves the 10-year survival of women who have ER-positive or ER-unknown tumors.
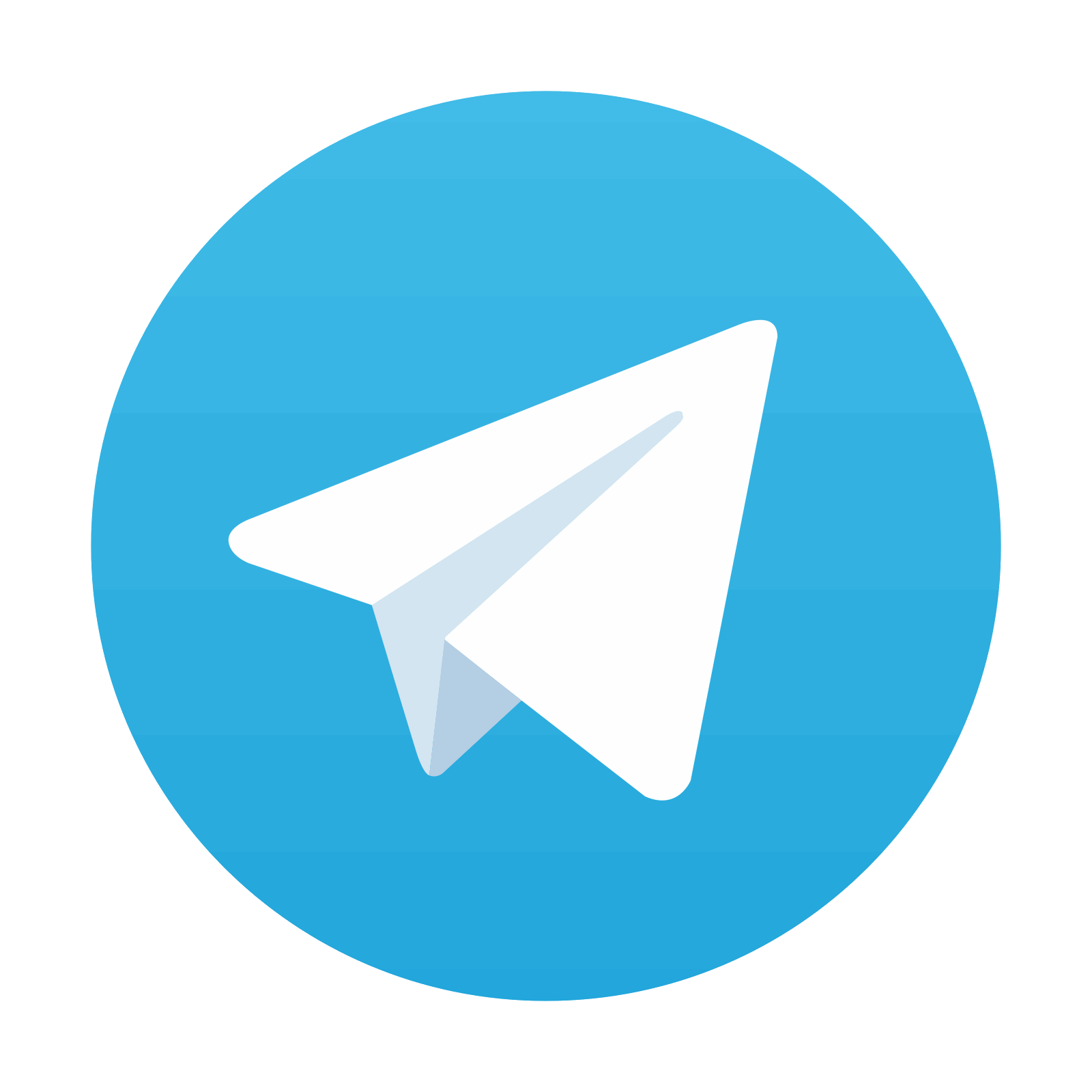
Stay updated, free articles. Join our Telegram channel

Full access? Get Clinical Tree
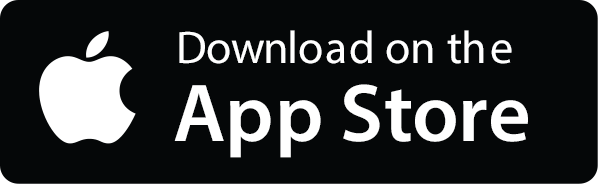
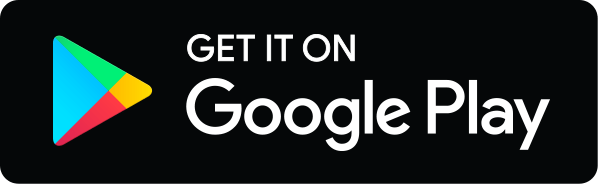