Disorders of the Spleen
Matthew R. Porembka
Majella Doyle
William C. Chapman
The spleen is a small, purplish, sponge-like organ found in the left upper abdominal quadrant.1 Although there is no mention of the spleen in the Bible, it receives considerable attention in the Talmud and post-Talmudic literature. Classically identified as the seat of laughter, there has been much debate over whether the spleen is truly necessary.2 It is said that Galen called it an “organ full of mystery” as early as the second century A.D. Although we have come to understand much about the structure and function of the spleen since those times, much of what intrigued Galen still remains a mystery today. The spleen has been classified into different organ systems including the circulatory system, the hematopoietic system, the mononuclear phagocyte system, or the lymphatic system. However, it is probably more accurate to describe the spleen as a clearinghouse for circulating cellular elements—a place where multiple organ systems converge in both structure and function.
DEVELOPMENT AND ANATOMY
The spleen is derived from embryonic mesoderm. The developing organ first becomes apparent during the fifth week of gestation as mesodermal cells coalesce between the leaflets of the dorsal mesogastrium, posterior to the developing stomach. As development continues, independent lobules enveloped by mesogastrium become evident. In time, they fuse to form a multilobulated mass that eventually differentiates into a well-formed organ by late fetal life.3 Occasionally, a stray lobule may fail to fuse with the others, develop independently, and give rise to an accessory spleen, a functioning mass of splenic tissue set apart from the body of the organ proper. The mesogastrium enveloping the lobules eventually gives rise to the organ’s capsule and trabecular skeleton, and the posterior and anterior attachments give rise to the primary supporting structures: the splenorenal and gastrosplenic ligaments, respectively.4 The colonization of the cellular elements of the spleen occurs first with the erythroid and myeloid progenitors, followed by the first hematopoietic stem cells. Finally, lymphoid-tissue-inducer cells are observed and provide the necessary local cellular signals to trigger the unique architecture of the splenic cellular elements. This development is highly similar to that of lymph nodes.5
Gross Anatomy
Grossly, the spleen may appear in a variety of shapes. It may be wedge-shaped (44%), tetrahedral (42%), or triangular (14%), depending on its relationships with the stomach, left kidney, pancreas, and colon. The average adult spleen measures 13 to 15 cm in length, 8 to 10 cm in width, and 4 cm in thickness, with a weight of 150 g and a corresponding blood volume of ˜300 mL.4 However, the size of the spleen varies with age, immunologic status, and nutritional status.1
The nonperfused organ appears purplish in color with a solid, sponge-like texture throughout. It is covered by a fibrous connective tissue capsule that is 1.5 mm thick and composed of collagen and elastin fibers.1 This capsule surrounds all but the hilum of the spleen.4 Continuous with the capsule, involutions penetrate the body of the organ to form trabeculae, the fibrous supporting skeleton of the spleen.3 Finally, a serosal membrane, derived from peritoneum, covers the organ externally and adheres to the capsule. This mesothelial membrane covers the entire organ except at its hilum and the reflections of its primary supporting ligaments.1
In the majority of people, the spleen is divided into the superior lobe and inferior lobe, but further division into subsegmental lobes can be seen.6 Avascular planes separate each lobe and they are defined by discrete, nonanastomosing circulatory pathways.1, 3, 6
Location and Relationships
The spleen is located in the left upper quadrant of the abdominal cavity, posteriorly, at the level of the ninth to eleventh thoracic vertebrae. The superior, or diaphragmatic, surface of the organ is convex in shape, smooth, and related to the left hemidiaphragm; whereas the inferior, or visceral, surface is somewhat triangular and rests on the splenic flexure of the colon.1, 4
The medial portion of the spleen is concave and divided by a longitudinal ridge into a gastric surface anteriorly and a renal surface posteriorly. The gastric surface contains the hilum of the organ and relates to the fundus of the stomach and the tail of the pancreas. The tail of the pancreas touches the spleen in 30% of cases and is within 1 cm of it in 73% of the population, contributing to the risk of pancreatic injury in patients undergoing splenectomy.7 The renal surface borders the superolateral surface of the left kidney and left adrenal gland. Figure 65.1 shows the gross appearance of the spleen as well as its relationship to the adjacent pancreatic tail and branch vessels of the splenic artery.
The spleen is supported by a number of suspensory ligaments: the splenorenal posteriorly, the gastrosplenic anteromedially, the splenophrenic superiorly, and the splenocolic inferiorly.1 The splenorenal and gastrosplenic ligaments provide the majority of support to the organ and are considered primary. The splenorenal ligament supports the organ posteriorly and serves as a conduit for the organ’s neurovascular bundle, whereas the gastrosplenic ligament stabilizes the spleen anteromedially to the greater curvature of the stomach and contains the short gastric and gastroepiploic vessels. The other ligaments of the spleen play a secondary role in the support of the organ and are generally
avascular.1, 4 However, in certain pathologic states, such as portal hypertension, significant collateral circulation through these planes may occur.
avascular.1, 4 However, in certain pathologic states, such as portal hypertension, significant collateral circulation through these planes may occur.
Vascular Supply
The spleen is a highly perfused organ. Although it represents only ˜0.2% of the total body weight, it receives > 5% of the total cardiac output.8 The main blood supply to the spleen is the highly variable splenic artery, which delivers 250 to 300 ml of arterial blood per minute. It arises as a branch of the aorta’s celiac trunk in the midline and follows a tortuous course to the left, enveloped within the splenorenal ligament, to supply the organ.1
The splenic artery is a highly unpredictable vessel that displays variations in course and dimension. In 95% of the population, it travels along the upper border of the pancreas, but it may also travel in front of, within, or behind the pancreatic tissue. Along its tortuous course, the splenic artery gives rise to the left gastroepiploic artery and the short gastric arteries, both of which run within the gastrosplenic ligament. At its terminal end, the splenic artery branches into a number of smaller arteries, or segmental branches, before penetrating the hilum of the organ. These smaller arteries are also highly variable but are most often four in number and include the superior polar, superior middle, inferior middle, and inferior polar splenic arteries. The segmental branches penetrate the organ by traveling within the trabeculae (sheaths of fibrous connective tissue derived from the involution of the spleen’s fibrous capsule) and ramify within the body of the organ. Additional sources of arterial blood to the spleen include direct tributaries and collateral circulation provided by branches of the pancreatic and short gastric arteries.1, 4
Venous drainage is facilitated primarily by the splenic vein. It is formed by the coalescence of the segmental splenic veins, as they leave the hilum of the organ, and the left gastroepiploic vein. Occasionally, short gastric veins may also participate. The splenic vein courses toward the midline along the superior border of the pancreas, where it joins the superior mesenteric vein to form the portal vein. Along the way, the splenic vein receives venous tributaries from the pancreas and, in 60% of cases, also receives the inferior mesenteric vein.1, 4
Lymphatics
The spleen, which is considered by many to be a lymphatic organ, has no afferent lymphatics, yet a significant amount of lymph fluid is expressed by the organ and drains via efferent vessels that form around the arterioles. The lymphatics travel with the neurovascular bundle through the trabeculae, exiting at the hilum.3, 9 In turn, the lymphatic fluid is directed toward the nodes of the splenic hilum, the splenic artery, and the pancreas (the pancreaticosplenic nodes), and eventually drains to the nodes of the celiac axis.4
Innervation
The spleen is innervated by fibers of the sympathetic nervous system which originate at the level of T-6 to T-8 and pass with the greater thoracic splanchnic nerve to the celiac ganglion.15 From there, they pass to the organ via the arterial tree, with resulting vasomotor function.1 It is believed that, similar to other mammals, the autonomic nervous system regulates changes in spleen volume, resulting in the expulsion of stored red blood cells during times of physiologic need.18, 25
Of interest are studies that relate the sympathetic innervation of the spleen to the immune system.10, 11, 12 These studies suggest that spleen innervation also supplies branches to the lymphocytic tissue of the white pulp, and that the innervation is plastic and able to be remodeled depending on the host’s current immune state.13 In this respect, these investigators argue, the central nervous system is hard-wired to the immune system and may mediate the observed immunosuppressive effects of stress.
Accessory Spleens
An accessory spleen is a functioning lobule of splenic tissue set apart from the body of the spleen proper. It is an anatomic variant present in 10% to 30% of the general population and appears to be found with greater frequency in patients with hematologic disorders. Figure 65.2 shows a typical example of an accessory spleen.
These supernumerary organs arise during embryologic development when an encapsulated lobule of precursor cells fails to fuse with others forming the spleen proper ; they are solitary in ˜88% but can be multiple in 10% of cases.14 In the majority of cases, accessory spleens receive their blood supply from a tributary of the splenic artery and are found most often near the hilum of the organ or within one of the primary supporting ligaments. However, they may be found within secondary supporting ligaments or within the greater omentum or even in as remote a location as the pelvis of the female or the scrotum of the male. They may be mistaken for malignancy on imaging studies, such as computed tomography (CT) or endoscopic ultrasound.
Because accessory spleens perform the same functions as the spleen proper, they are subject to the same pathologic conditions that affect the parent organ. Therefore, they may enlarge after splenectomy, causing a relapse of the disease process for which the spleen was removed. The presence of an unrecognized accessory spleen can account for failure of certain surgical procedures such as splenectomy for immune thrombocytopenic purpura (ITP).15, 16, 17, 18 In patients who continue to have thrombocytopenia after splenectomy, a search for a missed accessory spleen should be considered. Diagnosis may be made using abdominal sonography, CT, magnetic resonance imaging, nuclear scintigraphy, or Doppler sonography.19
Splenosis
In addition to accessory spleens, extrasplenic tissue may occur via traumatic autotransplantation in the form of splenosis. This is the migration and subsequent proliferation of dislodged splenic
tissue, and it is reported to occur in up to 75% of patients who undergo splenectomy for traumatic injury. Rarely, splenosis may be found in the gynecologic patient or in the thorax after rupture of the spleen and left hemidiaphragm.20, 21, 22 Histologic examination of splenetic tissue reveals the same elements of the parent organ: white pulp, red pulp, and marginal zones.20 It follows, therefore, that splenetic tissue should preserve at least some splenic function. Indeed, it has been observed that postsplenectomy patients with splenetic tissue do retain the ability to clear erythrocytes of undesirable inclusion bodies. However, it has not been demonstrated that this provides sufficient protection from postsplenectomy infectious complications, and the practice of splenic tissue reimplantation after splenectomy has been abandoned.23
tissue, and it is reported to occur in up to 75% of patients who undergo splenectomy for traumatic injury. Rarely, splenosis may be found in the gynecologic patient or in the thorax after rupture of the spleen and left hemidiaphragm.20, 21, 22 Histologic examination of splenetic tissue reveals the same elements of the parent organ: white pulp, red pulp, and marginal zones.20 It follows, therefore, that splenetic tissue should preserve at least some splenic function. Indeed, it has been observed that postsplenectomy patients with splenetic tissue do retain the ability to clear erythrocytes of undesirable inclusion bodies. However, it has not been demonstrated that this provides sufficient protection from postsplenectomy infectious complications, and the practice of splenic tissue reimplantation after splenectomy has been abandoned.23
Wandering Spleen
Wandering spleen refers to migration of the spleen from its normal location in the left upper quadrant. With an incidence of <0.5% in splenectomy series, the major complication of an ectopic spleen is torsion, either acutely or chronically. Signs and symptoms include vague or chronic abdominal discomfort and a tender abdominal mass; and CT assessment of viability leads to the decision of splenectomy versus splenopexy.
Microscopic Anatomy
Splenic tissue is supported by a scaffold of trabeculae, a dense, fibrous connective tissue skeleton that gives rise to an intrasplenic meshwork of collagen fibers. Vascular elements enter the spleen at the hilum, branch through the trabeculae, and penetrate the body of the organ to supply the microcirculation.1 As the arterial elements enter the parenchyma, they become surrounded by an aggregate of lymphoid tissue, which follows vessels in a sheathlike distribution. Aggregates of lymphoid tissue are collectively called the white pulp. White pulp may be arranged in this coaxial fashion, or it may appear as isolated follicles within the parenchyma of the organ. The balance of splenic tissue beneath the capsule is known as the red pulp. It is composed of vascular, circulatory, and mononuclear phagocytic elements. Additionally, an intermediate region can be observed at the junction between the white and red pulp; it is known as the marginal zone. Nerves and lymphatics follow the distribution of the larger vessels throughout the body of the organ.
White Pulp
The white pulp structure resembles that of a lymph node, with organized T and B cell compartments surrounding branching arterial vessels. The organization of the cellular elements within the white pulp is created and maintained by local chemokine gradients specific to T and B cells.3, 9 White pulp may be observed in one of two arrangements: a periarteriolar lymphatic sheath (PALS) or a lymphoid follicle. The PALS is a collection of T lymphocytes that surrounds intraparenchymal arterioles and follows these vessels in a coaxial fashion for several millimeters throughout their terminal distribution. Within the PALS, T cells interact with dendritic cells and passing B cells. The lymphoid follicles are spherical collections of B lymphocytes. These follicles are usually distributed along the length of the PALS in an eccentric fashion and house activated B cells undergoing clonal expansion.9
Red Pulp
The red pulp represents approximately three-fourths of the splenic volume and is composed primarily of vascular elements surrounded by a fibrocellular reticulum that contains mononuclear phagocytic cell lines and circulating elements of blood in transit.1 The chief vascular component of the red pulp is the splenic sinus, a preliminary venous element whose structure is unique to this organ. The sinus is composed of an incomplete lining of elongated endothelial cells surrounded by a highly fenestrated basement membrane. The structure is supported externally by reticulin fibers wrapped in a transverse fashion. These stress fibers also have actin- and myosin-like filaments which regulate the porosity of the sinus. The unique architecture of the splenic sinus has been compared to the structure of a wooden barrel, with the elongated endothelial cells resembling the planks or staves and the external reticulin fibers representing the hoops.3
The splenic sinuses permeate the surrounding fibrocellular reticulum that supports them. Two-dimensional histologic observation reveals an intervening reticulum arranged in cords called splenic cords or cords of Billroth.1 These cords actually are a three-dimensional meshwork of densely packed elements (fibroblasts, collagen fibers, and cells of the mononuclear phagocytic lines). Terminal arterioles and capillaries deliver circulating blood to this meshwork that percolates through the cord toward the sinuses. Thus, circulating elements of blood in transit are also packed within the cords: erythrocytes, platelets, macrophages, lymphocytes, plasma cells, and granulocytes.1, 3, 9
Marginal Zone
The marginal zone is a transitional region between the white and red pulp and contains both lymphocytic and mononuclear phagocytic elements. Within this transitional zone, three distinct regions can be identified. Moving from white to red pulp are the marginal sinus, the marginal zone proper, and the perimarginal cavernous sinus plexus.3
The marginal sinus is a vascular sinus that surrounds the white pulp and is a region where arterioles terminate into an anastomosing complex of vascular spaces. External to the marginal sinus is the marginal zone. This transitional region contains elements of both white and red pulp and is where much of the circulating blood is presented to lymphocytic and mononuclear phagocytic cells.3, 24 Figure 65.3 illustrates a schematic view of the microcirculation within the spleen.
Many different cell types reside in the marginal zone. Its foundation is a framework of reticular fibroblasts which is continuous with the reticular fibroblasts of the red pulp and the cells lining the marginal sinus. The marginal zone macrophage, metallophilic macrophage, and B cell are the key cell types in this transitional zone. The marginal zone B cell is a specialized subset of B cells that differ phenotypically and functionally from follicular B cells and are considered a bridge between the innate and adaptive immune systems.25 Interactions between these cells are key in the marginal zone’s organization and integrity. Many transient cells
pass though this area as they circulate into the red pulp and back into the blood stream. Diapedesis of lymphocytes and dendritic cells occurs through a barrier cell layer from the marginal sinus into the white pulp; however, the exact mechanism of this localization remains unknown.25, 26
pass though this area as they circulate into the red pulp and back into the blood stream. Diapedesis of lymphocytes and dendritic cells occurs through a barrier cell layer from the marginal sinus into the white pulp; however, the exact mechanism of this localization remains unknown.25, 26
Microvasculature
As the splenic artery approaches the body of the organ, it divides into a series of branches, the proper splenic arteries. On average, four proper splenic arteries then enter the organ at the hilum and branch into trabecular arteries, so named because they run within the framework of the fibrous connective tissue skeleton, the trabeculae. Trabecular arteries leave the fibrous trabeculae and penetrate the parenchyma by branching into central arteries that immediately become surrounded by a cuff of white pulp, the PALS.3, 9
As the central arteries pass through the white pulp, they give off lateral branches at right angles, most of which terminate in and supply the marginal sinuses, and others that terminate within the marginal zone or red pulp.9, 27 The right angle by which these lateral tributaries branch off from the central artery is thought to mediate a plasma-skimming effect which functions to hemoconcentrate the blood with a greater number of cellular elements.27, 28, 29 This skimming effect may also help regulate plasma volume. Central arteries continue from the white pulp sheath through the marginal zone and eventually terminate in the red pulp, giving off additional lateral branches along the way that are known as penicillar arterioles.1 The penicillar arterioles, which are named for their resemblance to Penicillium molds, terminate in one of three fashions: They may circle back and supply the marginal sinus; they may terminate in the red pulp, supplying the splenic cords; and a minority may terminate directly into the venous sinuses for direct venous return. Eventually, the central artery itself terminates within the red pulp.24, 27, 29
Circulating elements of splenic blood flow begin their return by first entering the splenic sinuses, the preliminary venous vessels. Flow may enter the sinuses directly from arterial connections in a closed circulatory fashion. Alternatively, plasma and blood cells may reach the sinuses only after percolating through the reticulum of the parenchyma in an open circulatory fashion. Eventually, the venous sinuses coalesce and drain into trabecular veins that give rise to the segmental veins and, finally, the splenic vein.24, 27, 29
The exact details of splenic microcirculation remain elusive despite much research since its first description in 1901. The currently accepted models that describe the flow of blood through the vessels of the spleen discuss this in terms of the pathway taken, or route, and the kinetics, or speed of flow. Closed circulation implies that blood passes directly from artery to capillary to vein. In terms of the spleen, blood traveling along a closed circulatory path follows the terminal arterioles to capillaries and, eventually, into the venous sinuses. Indeed, such direct connections have been observed.24, 27, 29
The term open circulation implies that cells and plasma circulate outside of endothelium-lined vascular channels. As it pertains to the spleen, circulating blood may follow one of two open pathways: It may leave the endothelium-lined marginal sinus, traverse the white pulp, and drain into the open-ended sinuses of the perimarginal cavernous sinus plexus27; or it may leave penicillar arterioles that terminate in stroma of the red pulp, traverse the splenic cords, and reenter the venous sinuses through their fenestrations. Indeed, the unique vascular channels and microarchitecture of the spleen can support these open circulatory pathways, which also have been observed.9, 24, 29
Another model for discussing splenic microcirculation describes it in terms of kinetics or speed of flow.1, 27 Accordingly, intrasplenic circulation may be fast, intermediate, or slow, traversing the organ in seconds, minutes, or hours.1 Fast flow occurs along paths of low resistance, traverses the organ in seconds, and accounts for ˜90% of splenic flow.24 Pathways that support fast flow include the open circulation of the marginal zone and, to a lesser extent, the closed circulation of direct arterial, capillary, and sinus connections.27
Elements that flow at an intermediate speed cross the circulation within minutes rather than seconds and represent ˜9% of flow across the organ. These elements are thought to follow the open circulation through the cords where red cell processing— pitting and culling—occurs.
Finally, slow-flowing elements may take more than a day to traverse the organ and represent 1% of splenic flow. This is thought to represent a pool of maturing reticulocytes, which travel to the spleen from the bone marrow and undergo maturation before being released into the circulation.27 The role of the spleen in this function is well documented and supported by the fact that asplenic patients have a high concentration of immature reticulocytes in their peripheral circulation.30
SPLENIC FUNCTION
The functions of the spleen are probably best understood in terms of its unique structure. Composed of several different tissue types, it lies at the crossroads between the arterial supply and venous return. In this respect, the spleen functions as a unique lymphoid organ where elements of the circulatory, reticuloendothelial, and immune systems interact. Thus, it is ideally suited to play a critical role in the surveillance of circulating blood.
The spleen functions as a filter of the circulating blood, a coordinator of the immune response, and a reservoir for circulating cells and platelets. Additionally, the spleen may have a number of other responsibilities, including hematopoiesis, hemoglobin degradation and iron recovery, and plasma volume regulation.
Filter Function
One of the primary functions of the spleen is to filter blood. Elements removed from circulation by the spleen include aging or abnormal red cells, intraerythrocyte inclusions, and foreign particulate matter.3
Culling
The removal of aging or abnormal red blood cells by the spleen occurs within the cords of Billroth and is known as culling. Cells bound for destruction become trapped within the reticulum meshwork and, as their splenic transit time increases, they succumb to phagocytosis by resident macrophages.
Another theory contends that as erythrocytes age, they lose significant amounts of cytoplasmic membrane. Senescent cells therefore lack the deformability necessary to negotiate the meshwork and ultimately become trapped. This theory applies to red cells that are morphologically abnormal secondary to congenital disorders such as sickle cell anemia and hereditary spherocytosis. Finally, it has been proposed that as erythrocytes age, they expose surface antigens that are recognized by self-directed antibodies. Once opsonized with antibody, senescent cells are easily trapped by cordal macrophages.3, 9, 30, 31
Pitting
In addition to culling, the spleen also functions to remove intraerythrocyte inclusions from circulating red cells. The ability of the spleen to clear these inclusions while maintaining the integrity of the red cell itself is known as pitting and is an exclusive function of splenic tissue.
Undesirable intracellular elements removed by the spleen include circulating particulate matter, Heinz bodies (denatured hemoglobin), Howell-Jolly bodies (nuclear remnants), and Pappenheimer bodies (iron granules). Pitting occurs as cells within the cords attempt to reenter the circulation through the splenic sinuses. To do this, they must pass through slit-like fenestrations of the sinus endothelium. As this occurs, the deformable portion of the cell bends to negotiate the opening, whereas the inclusion, which is nondeformable, is unable to pass through the narrow passage; thus, it is left behind to be phagocytized by resident macrophages. The passage of red cells through the slits is mediated by active changes within the sinus endothelial cell stress fibers. These cells have been shown to have cytoskeletons that include the contractile protein components actin and myosin.3, 22,31 Figure 65.4 is a schematic representation of the process of pitting.
Evidence that supports the role of the spleen in culling and pitting is found in the fact that, after splenectomy, asplenic and hyposplenic patients lose their ability to clear damaged red cells and intraerythrocyte inclusion bodies from the circulation. These patients display peripheral blood smears with an abnormal variety of erythrocytes, many with intracellular inclusions. In fact, determination of the percentage of pitted erythrocytes is a wellestablished method of assessing splenic function.30, 31
Clearance of Particulate Matter
Another important filtering function of the spleen is its ability to remove particulate matter from the circulation. As blood travels through the meshwork of the cords, foreign particles are exposed to splenic macrophages that clear them by phagocytosis. Experiments with injected carbon particles have confirmed this process and have identified the cells chiefly responsible for this function: The macrophages surrounding the splenic sinuses.
Immune Function
Because the spleen is composed of lymphocytic tissue, circulatory elements, and mononuclear phagocytic cell lines, it is ideally suited to play a coordinating role in the immune response. The organ’s function in this respect includes its role in the nonspecific as well as the specific arm of the immune response, making it an important organ for immune homeostasis. The nonspecific immune functions of the spleen include the clearance of pathogens, the clearance of opsonized erythrocytes and platelets, and the production of complement. The ability of the spleen to clear blood-borne microorganisms and debris combined with its highly organized lymphoid compartment makes the spleen the most important organ for antibacterial and antifungal immune reactivity.
The spleen plays a significant role in the removal of bloodborne pathogens from the circulation. Once coated with complement, bacteria and viruses become circulating immune complexes. Although the liver clears some of these complexes, many others are delivered to the spleen and eliminated by marginal zone cells expressing specific receptors including pattern-recognition receptors, C-type lectin SIGNR1, and type 1 scavenger receptor MARCO.32 Marginal zone metallophilic macrophages also function to concentrate pathogens in the spleen, leading to their opsonization, and produce high levels of interferon-α and interferon-β
after viral exposure.26 Marginal zone B cells are specialized to detect circulating pathogens, after which they rapidly differentiate into immunoglobulin (Ig) M producing plasma cells or antigen presenting cells capable of CD4+ T cell activation.26 The contribution of the spleen to this process is supported by the fact that asplenic patients display a higher level of circulating immune complexes than do spleen-competent subjects.
after viral exposure.26 Marginal zone B cells are specialized to detect circulating pathogens, after which they rapidly differentiate into immunoglobulin (Ig) M producing plasma cells or antigen presenting cells capable of CD4+ T cell activation.26 The contribution of the spleen to this process is supported by the fact that asplenic patients display a higher level of circulating immune complexes than do spleen-competent subjects.
The ability of the spleen to clear encapsulated bacteria is especially significant. Because these organisms have the ability to evade antibody and complement binding, their clearance depends on prolonged contact between pathogen and macrophage. It is well recognized that asplenic or hyposplenic patients are prone to a syndrome of fulminant septicemia, most often involving encapsulated bacteria: Overwhelming postsplenectomy sepsis.31
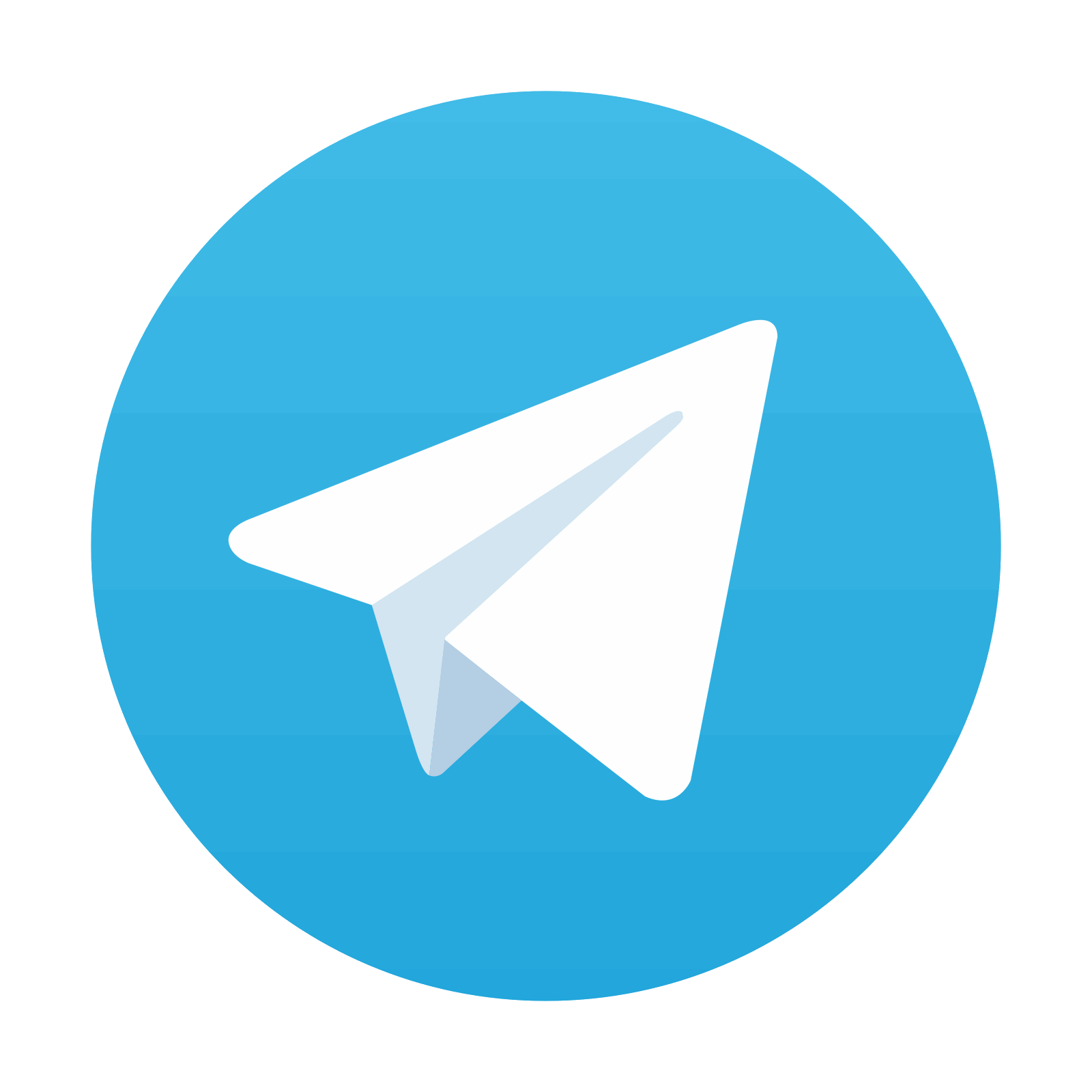
Stay updated, free articles. Join our Telegram channel

Full access? Get Clinical Tree
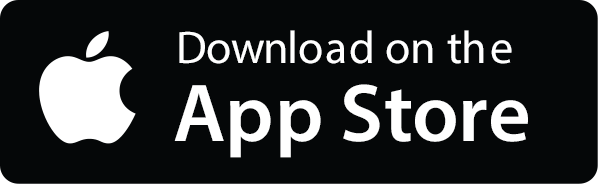
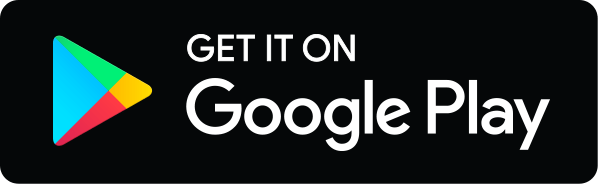