INTRODUCTION
SUMMARY
The clonal myeloid neoplasms result from acquired driver and cooperating mutations within a multipotential marrow cell, or sometimes, perhaps, a stem cell. Translocations, inversions, duplications (e.g., trisomy, tetrasomy), and deletions of chromosomes can result in (1) the expression of fusion genes that encode oncogenic fusion proteins or (2) the overexpression or underexpression of genes that encode molecules critical to the control of cell growth, programmed cell death, cell differentiation and maturation, or other regulatory pathways. Gene sequencing has also identified relevant somatic mutations in cases without an overt cytogenetic abnormality. The different mutations may result in phenotypes that range from mild impairment of the steady-state levels of blood cells, insignificant functional impairment of cells, and a modest effect on longevity to severe cytopenias and death in days, if the disorder is untreated. The somatically mutated multipotential cell from which the clonal expansion of neoplastic hematopoietic cells derives acquires the features of a stem cell and retains the ability, with varying degrees of imperfection, to differentiate and mature into each blood cell lineage. A particular disease in this spectrum of phenotypes may have altered blood cell concentrations and cell structural and functional abnormalities, and these may range from minimal to severe, involving several blood cell lineages. The effect on any one lineage occurs in an unpredictable way, even in subjects within the same category of disease. The resulting phenotypes are, therefore, innumerable and varied. In polycythemia vera or essential thrombocythemia, differentiation and subsequent maturation of unipotential progenitor cells results in blood cells nearly normal in appearance and function, but their level in the blood is excessive. Moreover, overlapping features are common, such as thrombocytosis as a feature of polycythemia vera, essential thrombocythemia, primary myelofibrosis, and chronic myelogenous leukemia. The clonal (refractory) anemias may be accompanied by functionally insignificant or very severe neutropenia or thrombocytopenia or sometimes thrombocytosis. These findings reflect the unpredictable expression of the mutant multipotential hematopoietic cell’s differentiation and maturation capabilities for which the genetic explanations are not well defined. The mutant cell of origin takes on the features of a (leukemic) stem cell, responsible for sustaining the disease process. Tight relationships between the genetic alteration and phenotype occur in only a few circumstances, and even these are imperfect, for example, t(9;22)(q34;q11)(BCR-ABL1; p210) with chronic myelogenous leukemia and t(15;17)(q22;q21) (PML-RARα) with acute promyelocytic leukemia. However, most patients can be grouped into a classic diagnostic designations listed in Table 83–1. The mutant stem cells that maintain the clone may undergo further somatic mutations over time resulting in a more aggressive phenotype, notably acute leukemia, usually of the myeloid type. An important feature of the clonal myeloid diseases is the potentially reversible suppression of normal (polyclonal) stem cells by the clonally expanded neoplastic cells. The coexistence of normal polyclonal stem cells and their competition with the neoplastic clone forms the basis for the remission-relapse pattern seen in acute myelogenous leukemia after intensive chemotherapy and the reappearance of polyclonal, normal hematopoiesis in patients with chronic myelogenous leukemia after tyrosine kinase BCR-ABL inhibitor therapy. This reciprocal relationship between the leukemic clonal and polyclonal normal stem cells may be mediated by the effects of the mass of neoplastic cells (inhibitory cytokine elaboration) and/or to the effect of the neoplastic clone on the stem cell niche and the resulting disturbance of stromal cell support for normal stem cell function.
I. Minimal-deviation neoplasms (no increase in blast cells [<2%] are evident in marrow) A. Underproduction of mature cells is prominent 1. Clonal (refractory sideroblastic or non-sideroblastic) anemiaa (Chap. 87) 2. Clonal bi- or tricytopeniaa (Chap. 87) 3. Paroxysmal nocturnal hemoglobinuria (Chap. 40) B. Overproduction of mature cells is prominent 1. Polycythemia verab (Chap. 84) 2. Essential thrombocythemiab (Chap. 85) II. Moderate-deviation neoplasms (very small proportions of leukemic blast cells present in marrow) A. Chronic myelogenous leukemia (Chap. 89) 1. Philadelphia (Ph) chromosome-positive, BCR rearrangement positive (~90%) 2. Ph chromosome-negative, BCR rearrangement positive (~6%) 3. Ph chromosome-negative, BCR rearrangement negative (~4%) B. Primary myelofibrosisb (chronic megakaryocytic leukemia) (Chap. 86) C. Chronic eosinophilic leukemia (Chaps. 62 and 89) 1. PDGFR rearrangement-positive 2. FGFR1 rearrangement-positive D. Chronic neutrophilic leukemia (Chap. 89) 1. CSF3R-rearrangement-positive 2. CSF3R and SETBP1-rearrangement positive 3. JAK2V617F-rearrangement positive E. Chronic basophilic leukemia (Chap. 89) F. Systemic mastocytosis (chronic mast cell leukemia) (Chap. 63) 1. KITD816V mutation-positive (~90%) 2. KITV560G mutation-positive (rare) 3. FILIPI-PDGFRα III. Moderately severe-deviation neoplasms (moderate concentration of leukemic blast cells present in marrow) A. Oligoblastic myelogenous leukemia (refractory anemia with excess blasts)a (Chap. 87) B. Chronic myelomonocytic leukemia (Chap. 89) 1. PDGFR rearrangement positive (rare) C. Atypical myeloproliferative disease (syn. atypical chronic myelogenous leukemia) D. Juvenile myelomonocytic leukemia (Chap. 89) IV. Severe-deviation neoplasms (leukemic blast or early progenitor cells frequent in the marrow and blood) A. Phenotypic variants of acute myelogenous leukemia (Chap. 88) 1. Myeloblastic (granuloblastic) 2. Myelomonocytic (granulomonoblastic) 3. Promyelocytic 4. Erythroid 5. Monocytic 6. Megakaryocytic 7. Eosinophilicc 8. Basophilicd 9. Mastocytice 10. Histiocytic or dendriticf B. High-frequency genotypic variants of acute myelogenous leukemia [t(8;21), Inv16 or t(16;16), t(15;17), or (11q23)]g C. Myeloid sarcoma D. Acute biphenotypic (myeloid and lymphoid markers) leukemiah E. Acute leukemia with lymphoid markers evolving from a prior clonal myeloid disease |
Acronyms and Abbreviations
ABL1, Abelson murine leukemia viral oncogene homologue 1; ALL, acute lymphocytic leukemia; AML, acute myelogenous leukemia; BCR, breakpoint cluster gene; CALR, calreticulin gene; CD, cluster of differentiation; CEPBA, CCAAT/enhancer-binding protein α gene; CML, chronic myelogenous leukemia; FGFR, fibroblast growth factor receptor; FLT-3, FMS-like tyrosine kinase 3; G-banding, Giemsa banding; GPI, glycosylphosphatidylinositol; inv, inversion; JAK2, Janus kinase 2; miRNA, microribonucleic acid; MPL, myeloproliferative leukemia virus gene; NPM1, nucleophosmin 1 gene; PDGFR, platelet-derived growth factor receptor; PNH, paroxysmal nocturnal hemoglobinuria; t, translocation; WHO, World Health Organization.
A wide array of clonal (neoplastic) syndromes or diseases can result from somatic mutations in a multipotential hematopoietic progenitor cell (Table 83–1). This mutated neoplastic cell behaves like a hematopoietic stem cell (albeit, a cancer or leukemia stem cell), in that it is self-replicating, can differentiate, and feed progenitor cells into the various hematopoietic lineages. These leukemic, unipotential progenitors can undergo varying degrees of maturation to phenocopies of mature blood cells. Strong circumstantial evidence has existed for a myelogenous leukemia stem cell for approximately 60 years. This concept has been buttressed by experimental verification of such cells by transplantation of the human disease into immunodeficient mice1 and by techniques to isolate and characterize their stem cell phenotype.2,3 Although most attention has been given to the leukemic stem cell in acute myelogenous leukemia (AML) and chronic myelogenous leukemia (CML), it is very likely that a similar cell underlies (initiates and sustains) each of the phenotypically distinctive clonal myeloid diseases.
The clonal myeloid diseases can be grouped, somewhat arbitrarily, by their degree of malignancy, using the classic terminology of experimental carcinogenesis, which considers the degree of loss of differentiation and maturation potential and the rate of progression of the disease. Thus, myeloid malignancies can be viewed in the spectrum of minimally to severely deviated neoplasms (leukemias). The term deviation refers to the relationship of the disease in question to normal cellular differentiation and maturation and the regulation of cell population homeostasis (birth and death rates). This terminology has been used here to array the diagnostic categories of clonal hematopoietic diseases into a framework related to their pathogenesis for the reader. This approach is an effort to encourage thinking about these somewhat arbitrary diagnostic categories in pathobiologic terms and not just as a list of conditions or by epiphenomena such as disturbed morphology of blood cells that is shared to varying degrees in all categories of these disease (e.g., the dysmorphia of primary myelofibrosis is as dramatic as that of clonal cytopenias or oligoblastic myelogenous leukemia, so-called myelodysplastic syndromes.)
MINIMAL-DEVIATION CLONAL MYELOID DISORDERS
The neoplasms in this category in Table 83–1 retain a higher degree of differentiation and maturation capability and permit median life spans measured in decades without treatment or with minimally toxic treatment approaches.4 Use of the term minimal-deviation should not be construed as indicating these conditions do not have morbidity, shorten life, and have other consequences to the patient. The term is used relative to AML, in which differentiation and maturation and regulation of cell proliferation and cell death are profoundly disturbed, and in which expected life span is measured in days to weeks, if untreated. The minimal-deviation clonal myeloid diseases include one group in which late precursor apoptosis (ineffective myeloproliferation) is characteristic (the clonal cytopenias) and one group in which proliferation is exaggerated and cellular maturation approximates normal (effective myeloproliferation).
The clonal (refractory) anemias and bi- and tricytopenias are characteristic of this category. Cytopenias resulting from exaggerated apoptosis of marrow late precursors (referred to as “ineffective hematopoiesis”) are a principal feature of this subgroup of clonal hematopoietic multipotential cell diseases. A common additional characteristic is variable dysmorphogenesis of blood cells. The blood cell abnormalities, characteristic of the clonal anemias, bicytopenias, or pancytopenia, include abnormalities of (1) red cell size (macrocytosis, anisocytosis), shape (poikilocytosis), and cytoplasm (basophilic stippling), (2) neutrophil nuclear or organelle structure (cytoplasmic hypogranulation, nuclear hypolobulation or hyperlobulation and condensation), and (3) platelet variation in size (megathrombocytes) and granulation (hypogranulation or abnormal granulation). These structural changes are the result of neoplasia. Abnormal maturation of blood cells may also leads to biochemical and functional alterations of the cells, such as disturbed hemostasis, despite adequate platelet numbers, and dysfunctional phagocytes. Dysmorphic changes in marrow precursors are evident, also (Chap. 87). Ineffective erythropoiesis, the intramedullary, apoptotic death of late erythroblasts before they reach full maturation and release, is a common feature, a major factor in development of anemia. Ineffective granulopoiesis and thrombopoiesis also occur, resulting in varying degrees of neutropenia and thrombocytopenia, despite a cellular marrow.
There is no clinical distinction in the presenting manifestation or the course of clonal anemia with less than 15 or equal to or greater than 15 percent pathologic sideroblasts in the marrow,5 not surprisingly, as there is no pathobiologic basis for this arbitrary boundary. Therefore, this distinction nonsideroblastic vis-à-vis sideroblastic clonal (refractory) anemia has no nosologic or clinical utility, although the World Health Organization (WHO) has retained it.6 Indeed, the clonal anemias frequently have some degree of pathologic sideroblasts in the marrow, and, thus, usually have some degree of sideroblastic erythropoiesis. Another important feature of these syndromes is that there is no quantitative evidence of leukemic blast cells in marrow or blood. If marrow blasts are elevated above the normal upper limit of 2 percent, the disorder should be considered oligoblastic myelogenous leukemia (synonym: refractory anemia with excess blasts; see “Moderately Severe-Deviation Disorders” below).
The WHO has defined “AML” as having equal to or greater than 20 percent leukemic blast cells in marrow; whereas, a marrow with fewer blasts (5 to 20 percent) is referred to as refractory anemia with excess blasts (e.g., one of the myelodysplastic syndromes). The use of an arbitrary boundary of 20 percent blasts has no pathobiologic basis.7,8 In addition, the use of less than 5 percent of blasts as a threshold to distinguish clonal anemia (refractory anemia) from oligoblastic myelogenous leukemia (refractory anemia with excess blasts) is an anachronism that dates back approximately 60 years to a time when supportive care was inadequate for children undergoing newly developed multidrug chemotherapy for acute lymphoblastic leukemia (ALL). At that time, the mid-1950s, there was no accessibility to platelet transfusions. There were very limited antibiotic options and no antifungal agents. There were no venous access devices. The risk of death during prolonged posttherapy marrow aplasia was substantial and it was not yet evident that intensive antileukemic therapy would produce a net benefit to the children so treated. In children with ALL, there were often occasional residual atypical lymphoid cells in the marrow after treatment. To deal with these circumstances an arbitrary threshold of less than 5 percent atypical lymphoid cells (suspected blasts) was used as a measure of successful induction therapy to avoid an unnecessarily long period of posttreatment–induced aplasia.7 That boundary, however, was not intended to be a threshold to be used at the time of diagnosis. The normal myeloblast percentage is a very tightly regulated variable (mean: 1.0; SD: 0.4). In severe inflammatory states with leukemoid reactions, the marrow myeloblast percent is usually decreased because in this circumstance precursor cell expansion in the postblast cell myelocyte pool is greater. Three or 4 percent blast cells in the marrow at the time of presentation or suspected relapse should not be considered “normal” and is usually evidence of leukemic hematopoiesis. Indeed, in the presence of an established clonal myeloid disorder (e.g., clonal anemia), any percentage of blast cells, no matter how low the percentage is presumably part of the clone and thus “leukemic.” Not surprisingly, sophisticated multicolor flow analysis has found immunophenotypic abnormalities in such blast cells indicating that they are not “normal” blasts.9
In no other cancer is the diagnosis defined by the proportion of cancer cells in histologic or cytologic examinations. Thus, using equal to or greater than 20 percent blasts as the basis for diagnosis of AML versus myelodysplasia represents an aberration in cancer diagnosis and has no pathophysiologic basis.7,8 Indeed, studies have shown that there are no differences in the presenting hematologic findings or a series of prognostic genetic markers, for example, the FMS-like tyrosine kinase 3 (FLT-3) gene mutation in patients with 10 to 19 percent versus 20 to 30 percent marrow leukemic myeloblasts at the time of diagnosis.7 The patient’s disease features are the same regardless of whether they have 10 or 30 percent blast cells in the marrow at diagnosis; prognosis was correlated with patient age at diagnosis and the cytogenetic risk category and not the blast count. Moreover, several phenotypes of AML may have less than 20 percent blasts at diagnosis (e.g., acute promyelocytic leukemia, acute monocytic leukemia, acute myelomonocytic leukemia, and others).
The term hematopoietic dysplasia, later simplified to myelodysplasia, has become ensconced as the category into which clonal anemia, clonal multicytopenia, and oligoblastic myelogenous leukemia (refractory anemia with excess blasts) have been grouped. In strict pathologic terms, a dysplasia is a polyclonal, and thus nonmalignant, change in the cells of a tissue.8,10 These myeloid syndromes are clonal, often have aneuploid or pseudodiploid cells in the clone, are the result of the expansion of a somatically mutated cell, and can be associated with significant morbidity and premature death; thus, they are neoplasias not dysplasias. They demonstrate clonal (genomic) instability, and each has a propensity to evolve into AML at a rate that far exceeds the incidence of the disease in the general population. The term myelodysplasia was proposed at a conference in Paris in 1976 at a time when prominent dysmorphogenesis and cytopenias were thought to be the singular abnormalities and arguments existed as to whether some of these syndromes without increased blast cell percentages represented a preneoplastic (preleukemic) condition.11 They have long been established as neoplastic (a spectrum of minimal-deviation to severe-deviation leukemias)—indeed, those with overt leukemic hematopoiesis (quantitatively increased leukemic blast cell counts), which made up approximately 50 percent of cases, were known at the time to be neoplasms—but the terminology has not been rectified.
Polycythemia vera (Chap. 84) and essential thrombocythemia (Chap. 85) are clonal myeloid disorders so named because of the overaccumulation of red cells, and often neutrophils, and platelets in the blood in polycythemia, and of platelets, and to a lesser extent neutrophils, in thrombocythemia.12 Each cell lineage is affected in each disorder, reflecting a multipotential hematopoietic cell origin, but the magnitude of the effect on each lineage differs. The decrease in red cell production in essential thrombocythemia usually is slight to mild. Polycythemia vera and essential thrombocythemia do not show morphologic evidence of leukemic hematopoiesis; the proportion of blast cells in the marrow is never increased above normal, and blast cells are never present in the blood. Hematopoietic differentiation and maturation are maintained. These are minimal-deviation neoplasms. These disorders do not have a specific cytogenetic abnormality, but approximately 95 percent of cases of polycythemia and approximately 50 percent of cases of essential thrombocythemia have an acquired mutation in the Janus kinase 2 (JAK2) gene. In thrombocythemia, 25 percent of patients have wild-type JAK2 genes and mutations in the calreticulin (CALR) gene. A few percent of patients with thrombocythemia have nonmutated JAK2 and CALR but a mutation in the myeloproliferative leukemia virus gene (MPL; Chaps. 84 and 85).13,14 Several studies of comparative survival of the chronic myeloproliferative neoplasms have been reported.4,15,16,17,18,18a In the most comprehensive study of survival as of this writing, patients with essential thrombocythemia have only slightly decreased survival than expected over 10 years of observation, but this widens somewhat over longer periods. The difference in survival of patients with primary myelofibrosis is dramatically less than expected for age- and gender-matched unaffected persons and the survival of patients with polycythemia vera is intermediate (Table 83–2).18a
Primary myelofibrosis (Chap. 86) and CML (Chap. 89) classically share the features of overproduction of granulocytes and platelets and impaired production of red cells. In contrast to the minimally deviated clonal myeloid neoplasms, CML and primary myelofibrosis may have a small to moderate proportion of leukemic blast cells in marrow and blood. The most constant feature in primary myelofibrosis is the abundance of neoplastic, dysmorphic megakaryocytes and the resultant predisposition to marrow reticulin and collagen fibrosis, osteosclerosis, extramedullary fibrohematopoietic tumors, splenomegaly, and teardrop-shaped red cells (dacryocytes) in every oil immersion field on the blood film. The megakaryocytic abnormalities are so dominant and consistent in this disorder that it could be considered chronic megakaryocytic leukemia.19 The cells in this disorder have no specific cytogenetic change, but approximately 50 percent of cases carry a mutation in the JAK2 gene and approximately one-third have wild-type JAK2 but a mutation in the CALR gene (Chap. 86).13,14 These two mutations give primary myelofibrosis a genetic kinship with polycythemia vera and essential thrombocytosis. They are often referred to as “the myeloproliferative neoplasms,” but virtually all clonal myeloid diseases are fundamentally myeloproliferative as the term refers, principally, to marrow hematopoiesis. The clinical behavior of primary myelofibrosis is, in most cases of a progressive neoplasm with morphologic evidence, of lower-level leukemic hematopoiesis and with a median survival significantly less than polycythemia vera or essential thrombocythemia. Primary myelofibrosis is another misnomer perpetuated in the WHO classification. The fibrosis is secondary to cytokines released by neoplastic (leukemic) megakaryocytes (an epiphenomenon) and it is the only cancer in the medical lexicon named after connective tissue fibers and not the cells in which the cancer arises.19
In contrast to primary myelofibrosis, CML has a rearrangement of the breakpoint cluster (BCR) gene on chromosome 22. The shortening of the long arm of chromosome 22 gives it the designation of the Philadelphia chromosome, now called the Ph chromosome. It can be identified by Giemsa (G)-banding cytogenetic studies in approximately 90 percent of patients with CML. This mutation is caused by and is a reflection of the translocation t(9;22)(q34;q11)(BCR-ABL1 [Abelson murine leukemia viral oncogene homologue 1]). The BCR-ABL1 fusion in CML cells can be found in virtually all cases studied by fluorescence in situ hybridization or the polymerase chain reaction. Only approximately 4 percent of patients with a phenotype indistinguishable from BCR-rearrangement–positive CML do not have the rearrangement (see Table 83-1 and Chap. 89). An unrelenting increase in the white cell (granulocyte) count, anemia, splenomegaly, and a progressive course are common features of CML. Blast cells are very slightly increased in marrow and in the blood in patients with these two disorders, although this is a function of time of diagnosis in relation to the time of onset. CML, if untreated, has a very high propensity to progress through clonal evolution to acute leukemia.
Primary myelofibrosis terminates in acute leukemia in approximately 15 percent of patients. Median life span in these disorders is measured in years, but is significantly decreased compared to age- and gender-matched unaffected cohorts. Therapy is required in all cases of CML, and in most, but not all, cases of primary myelofibrosis at the time of diagnosis. Both diseases can be cured by allogeneic hematopoietic stem cell transplantation. Median life span is projected to be increased by decades in CML as a result of the introduction of tyrosine kinase inhibitors, which results in involution of the malignant clone, restoration of polyclonal normal hematopoiesis, and a reduction in the risk of transformation to an accelerated phase of the disease and to acute leukemia in many patients (Chap. 89).20 A significant median prolongation of life (e.g., approximately median 2 years) result from JAK inhibitors in poor-prognosis primary myelofibrosis (Chap. 86).
Chronic neutrophilic leukemia, chronic eosinophilic leukemia, systemic mastocytosis, and chronic basophilic leukemia are included in this category. Chronic basophilic leukemia is a rare disease (Chap 89).21 Chronic neutrophilic leukemia is uncommon but well described and defined (Chap. 89). Chronic neutrophilic leukemia is associated with a mutation in the colony-stimulating factor 3 receptor gene (CSF3R) alone (approximately 30 percent of cases), or a combination of mutated CSF3R and a SET binding protein gene (SETBP1) mutation (approximately 60 percent of cases) or the JAK2V617F mutation alone (approximately 10 percent of cases). Chronic eosinophilic leukemia represents cases previously called hypereosinophilic syndrome with evidence of clonal hematopoiesis involving eosinopoiesis. Some cases are associated with a rearrangement of the platelet-derived growth factor receptor-β (PDGFR-β) gene (these are indicted in Table 83–1) because they are specifically responsive to the tyrosine kinase inhibitor imatinib mesylate or to a congener (Chaps. 62 and 89). Chronic clonal eosinophilia also may be associated with a PDGFR-α gene rearrangement, but histopathologic examination of the marrow also may be consistent with systemic mastocytosis with eosinophilia, with sheets of spindle-shaped mast cells and intense eosinophilia in blood and marrow. This rearrangement is usually the result of a FIP1L1–PDGFR-α fusion gene. Identification of this fusion gene in cases of mastocytosis with eosinophilia is important because of the sensitivity of the gene product to imatinib mesylate (or a congener). The mutation is inferred by a deletion in the CHIC2 gene found using fluorescence in situ hybridization, which narrowly separates FIP1L1 and PDGFR-α at chromosome 4q band 12. The cryptic deletion involving CHIC2 is too small to be seen on standard G-banding. A clonal myeloid syndrome that includes eosinophilia and a translocation between 8p11, at the site of the tyrosine kinase domain of the fibroblast growth factor receptor-1 (FGFR1) gene, and several different partner chromosomes, is not responsive to imatinib mesylate. Systemic mastocytosis may have several types of KIT gene mutation; KITV560G is sensitive to imatinib mesylate and KITD816V is insensitive to imatinib but may be responsive to second-generation tyrosine kinase inhibitors. PDGFR-α mutations also may be present in the cells of patients with systemic mastocytosis and be responsive to imatinib mesylate (or a congener).22
These disorders fall into a group that progresses less rapidly than acute leukemia and more rapidly than CML.23,24 They have a predisposition to develop with a granulocytic and monocytic phenotype, either morphologically or cytochemically. These diseases include oligoblastic myelogenous leukemia (refractory anemia with excess blasts), chronic myelomonocytic leukemia, and juvenile myelomonocytic leukemia. Occasional patients have an atypical or unclassifiable syndrome. The “unclassifiable syndrome” designation is used for uncommon cases that do not fall into a classical or easily classifiable designation and usually are seen in patients older than age 70 years.
The subacute syndromes produce more morbidity than do the chronic syndromes, and patients have a shorter life expectancy. These are leukemic states that have low or moderate concentrations of leukemic blast cells in marrow and often blood, anemia, often thrombocytopenia, and usually prominent monocytic maturation of cells (Chap. 88). The oligoblastic myelogenous leukemias compose approximately 50 percent of the cases that have been grouped under the title myelodysplastic syndromes. In all other malignancies, the presence of tumor cells determines the diagnosis, such as carcinoma of the colon or the uterine cervix, whether in situ, invasive, or metastatic. Use of the percentage of tumor (leukemic blast) cells as a threshold for the diagnosis of leukemia versus “dysplasia” is not consistent with usual practice; hence, the preference for oligoblastic myelogenous leukemia rather than myelodysplasia for patients with a quantitative increase in blast cells (>2 percent blasts), cytopenias, and dysmorphic cell maturation.8 Moreover, CML, chronic neutrophilic leukemia, chronic myelomonocytic leukemia, acute promyelocytic leukemia, and several other subtypes of AML invariably have fewer than 20 percent blasts in the marrow. Thus, the criteria used in the WHO classification system for clonal myeloid diseases have internal inconsistencies that can be dealt with by experts but are confusing to the less experienced and are not unifying.
A group of clonal myeloid diseases are referred to as atypical myeloproliferative disease or atypical CML (aCML) in the WHO classification. They are usually seen in older patients (>65 years), have a relatively low myeloblast percentage in marrow (<5 percent) and blood, and have an elevated white cell count ranging between 15 and 100 × 109/L, but which may be higher. They have anemia and thrombocytopenia and often splenomegaly. The blood and marrow usually have a progressively increasing proportion of promyelocytes and myelocytes as well as neutrophils, superficially simulating the appearance of CML, hence the use of the designation “aCML.” These cases never have a rearrangement in the BCR gene, are not responsive to tyrosine kinase inhibitors, and have a poor prognosis with a median survival of approximately 15 to 20 months. Because the granulocytic series often has some dysmorphia (e.g., acquired Pelger-Huët nuclear anomaly), the WHO seems reluctant to call it an atypical myeloproliferative disorder, which should be done as aCML is an inadvisable term. Their inconsistency is evident in the classification of primary myelofibrosis as a myeloproliferative disorder despite florid dysmorphia of all three major lineages. Dysmorphia is a feature of most neoplastic cells, of considerable diagnostic utility, of interest cytologically, but an epiphenomenon not central to the pathobiology of the neoplasm. Atypical myeloproliferative disease (aCML) has a relatively high frequency of CSF3R gene mutations, akin to chronic neutrophilic leukemia. Because the mutant gene is thought to cause dysregulation evidenced by myeloproliferation and exaggerated neutrophil counts, it underlines the preferred terminology.
Morphologic, histochemical, immunocytologic, and cytogenetic characteristics of cells in the blood and marrow provide the major basis for the diagnosis and classification of AML and its subtypes (Chaps. 11 and 88). Correlation among observers and between the morphologic method of classification and the monoclonal antibody reactivity-dependent classification of AML is imperfect.25,26,27 The approach that uses morphology, immunocytochemistry, and the immunophenotype is the most inclusive because virtually all cases can be placed into a morphologic subtype. Because immunophenotyping is a standard procedure in most clinical hematopathology laboratories, the results are readily available. Classification by cytogenetics is more limited because approximately 45 percent of cases of AML do not have a discernible cytogenetic abnormality by G-banding and many cases have different infrequent abnormalities, making this approach complex. Hundreds of unique patterns of cytogenetic abnormalities have been reported in cells of patients with AML, including unbalanced structural abnormalities, such as loss of part or all of chromosome 5 or 7, numerical abnormalities, such as an additional chromosome 8 (e.g., trisomy 8), or unbalanced and balanced structural abnormalities, such as translocation between chromosomes 8 and 21 or 15 and 17, or between chromosome 11 and many chromosome partners, or any one of numerous other abnormalities involving other chromosomes.28 Despite this heterogeneity, knowing the cytogenetic alteration is useful for estimating the probability of entering a sustained remission (risk category). For example, AML patients whose cells contain t(8;21), t(15;17), t(16;16), or inv(16) (approximately 20 percent of cases considering all age groups) are more likely to enter a prolonged remission or be cured with therapy. The cytogenetic findings may influence the drugs used for remission-induction therapy. Notably, patients with t(15;17) AML (approximately 7 percent of all new AML cases in the United States and twice that frequency in China) uniquely require use of all-trans-retinoic acid and arsenic trioxide to result in the best long-term outcome and, in many cases, a cure. Thus, combining light microscopy of blood and marrow with immunocytochemistry and cell-flow analysis immunophenotyping to designate the phenotypic subtype, supplemented by cytogenetics or molecular diagnostic methods, currently is the best approach to categorization of the AML subtype. The polymerase chain reaction may be particularly useful for determining subclinical (minimal) residual disease and monitoring therapy in cases in which an appropriate genetic marker is available, such as the t(8;21) or t(15;17) (Chaps. 88 and 89).
Gene expression profiling using chips containing tens, hundreds, or thousands of relevant genes can be used to further genotype and subclassify AML into prognostic groups.29,30 One would predict, based on cytogenetics, a large and diverse group of gene expression profiles for cases of AML. In one study of 200 cases of AML, some of 270 mutated genes among nine genes families (i.e., transcription factor, tumor-suppressor, signaling pathway, nucleophosmin encoder, DNA-methylation–related, chromatin-modifying, myeloid transcription factor, cohesion complex, and spliceosome-complex genes) were found in at least two cases.31 Genetic analysis is currently most useful in analyzing cases with prior stratification by some relevant variable. For example, a study of patients with AML who have normal karyotypes by standard cytogenetic methods (e.g., G-banding) has identified two groups by hierarchical gene clustering with significantly different survival after current therapy.32 Patients with AML whose cells contain a FLT-3 internal tandem duplication also can be stratified into more discriminating prognostic groups using hierarchical gene cluster analysis.33
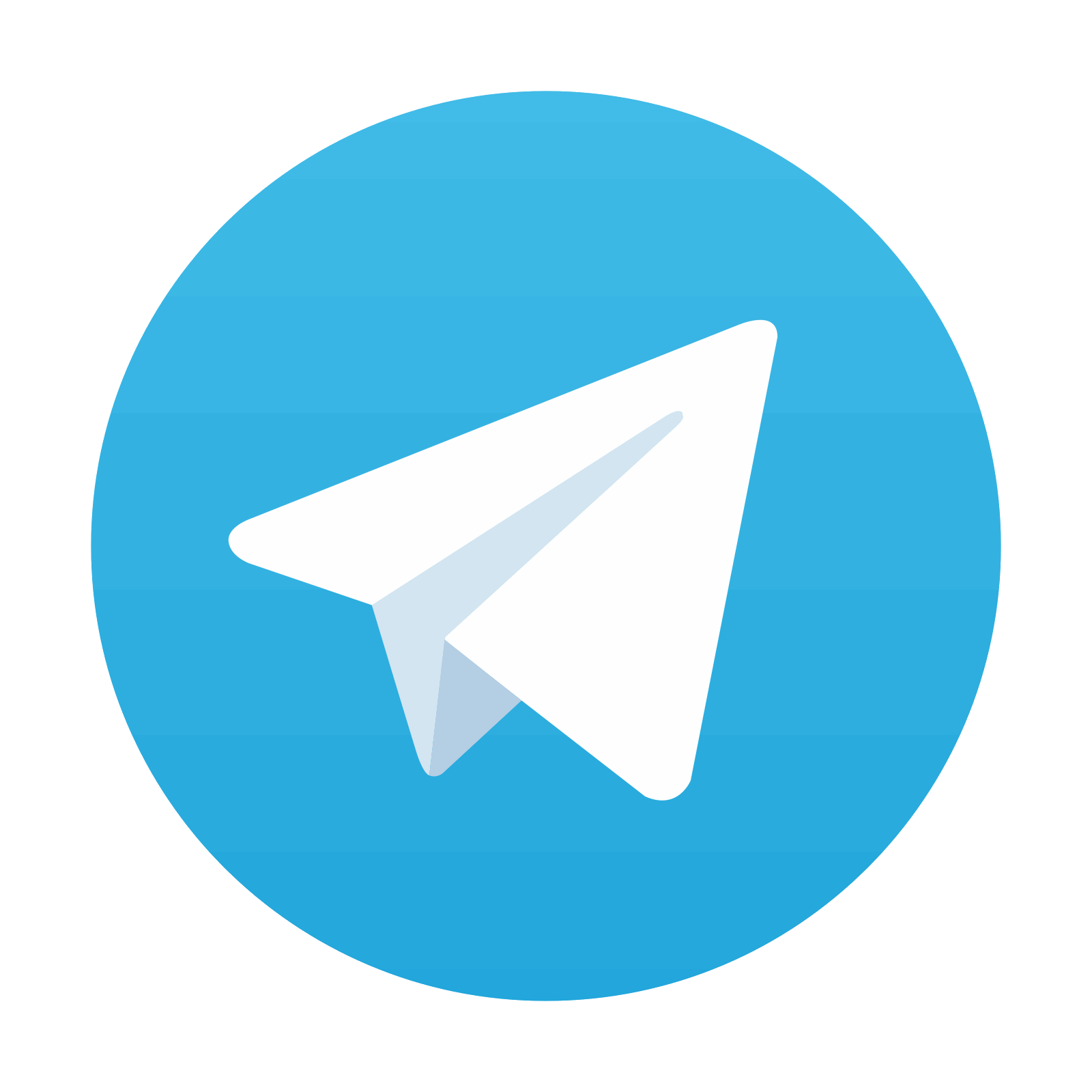
Stay updated, free articles. Join our Telegram channel

Full access? Get Clinical Tree
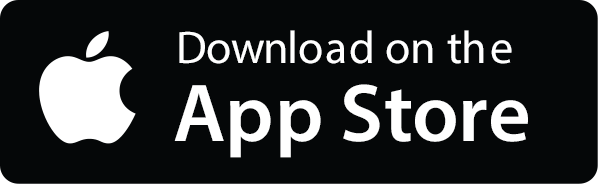
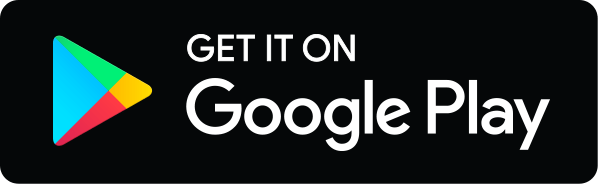
