CIRCADIAN RHYTHMS
Part of “CHAPTER 6 – ENDOCRINE RHYTHMS“
BASIC MECHANISMS
There is virtually neither a tissue nor a function in the human organism that does not exhibit regular changes from day to night. Distinct patterns of secretion recur at 24-hour intervals for essentially all hormones. Much of the temporal variability and organization of endocrine release ultimately results from the activity of two interacting time-keeping mechanisms in the central nervous system: endogenous circadian rhythmicity and sleep-wake homeostasis (Fig. 6-1). In mammals, endogenous circadian rhythmicity is generated by a pacemaker or group of oscillators located in the paired suprachiasmatic nucleus (SCN) of the hypothalamus.1 Sleep-wake homeostasis is an hourglass-like mechanism relating the amount and quality of sleep to the duration of prior wakefulness.2
The endogenous nature of human circadian rhythms has been established by experiments in which subjects were isolated in a soundproof chamber with no time cues available. Under those conditions, the subjects show free-running rhythms with periods, which deviate slightly from 24 hours and vary from one individual to another. In humans, the endogenous “free-running” period is usually slightly longer than 24 hours. In the past few years, enormous progress has been made in elucidating the molecular and genetic mechanisms underlying the generation of circadian rhythms in mammals. After the discovery of the first mammalian circadian clock gene, called Clock,3,4 a number of other mammalian clock genes were rapidly identified and sequenced.5 Intense efforts are now under way to understand how these genes and their protein products interact with each other through a series of activational and inhibitory pathways to produce the precise periodicity of the circadian signal generated in the SCN.
Environmental agents, primarily the light-dark cycle (i.e., photic cues), affect the expression and properties of the circadian signal. Light exposure in the later part of the subjective night and in the early part of the subjective day (e.g., around dawn) generally advances the phase of the circadian oscillation. In contrast, light exposure in the later part of the subjective day and during the early part of the subjective night (e.g., around dusk) delays circadian phase. Light-dark information reaches the SCN via a direct afferent pathway from the retina as well as an indirect projection via the intergeniculate leaflet of the thalamus.1 There also is good evidence to indicate that nonphotic cues are also capable of altering circadian function. Many of the non-photic stimuli that induce phase shifts in the circadian clock appear to do so by either stimulating physical activity or activating the pathways by which information about the activity-rest state of the organism reaches the clock.1 Phase-shifts may occur when activity pathways are stimulated during the normal rest period or, vice-versa, when rest is enforced during the normal active period. As is the case for light, the magnitude and direction of the phase-shifts depend on their timing relative to the internal circadian clock. There is now substantial evidence to indicate that the Raphe nuclei of the brainstem are involved in mediating the effects of activity on the circadian clock.
Sleep-wake homeostasis is thought to involve a putative neural sleep factor (factor “S”) that rises during waking and decays exponentially during sleep,2 and regulates the timing, amount, and intensity of the deeper stages of sleep. These stages of deep sleep correspond to stages III and IV of non– rapid-eye-movement (REM) sleep, usually referred to as slow-wave (SW) sleep because of the appearance of well-defined waves in the frequency range 0.5 to 4.0 Hz in the electroencephalogram. The neuroanatomical and neurochemical basis of sleep-wake homeostasis has not been entirely elucidated but appears to involve the basal forebrain cholinergic region and adenosine, a neuromodulator of which the extracellular concentrations in this region increase during sustained wakefulness and decrease during sleep.6 The mechanisms controlling sleep propensity and maintenance in the human appear to be somewhat different from those occurring in most other mammalian species. Indeed, human sleep is generally consolidated in a single 7-to
9-hour period, whereas fragmentation of the sleep period in several bouts is the rule in most other mammals. Possibly as a result of this consolidation of the sleep period, the wake-sleep and sleep-wake transitions in the human are associated with physiologic changes—and, in particular, endocrine changes—that are more marked than those observed in animals. Humans are also unique in their capacity to maintain wakefulness despite an increased pressure to sleep. While decrements in mood and cognitive function associated with such behaviors have long been recognized, these behaviors have been found to be associated with endocrine and metabolic alterations that may result in long-term adverse health consequences.6a
9-hour period, whereas fragmentation of the sleep period in several bouts is the rule in most other mammals. Possibly as a result of this consolidation of the sleep period, the wake-sleep and sleep-wake transitions in the human are associated with physiologic changes—and, in particular, endocrine changes—that are more marked than those observed in animals. Humans are also unique in their capacity to maintain wakefulness despite an increased pressure to sleep. While decrements in mood and cognitive function associated with such behaviors have long been recognized, these behaviors have been found to be associated with endocrine and metabolic alterations that may result in long-term adverse health consequences.6a
The pathways by which circadian rhythmicity, sleep-wake homeostasis, and their interactions modulate hormonal release are largely unknown. At the level of the central nervous system (see Fig. 6-1), humoral and/or neural signals originating from the hypothalamic circadian pacemaker and from brain regions involved in sleep regulation affect the activity of the hypothalamic structures responsible for the pulsatile release of neuroendocrine factors (e.g., corticotropin-releasing hormone [CRH], growth hormone–releasing hormone [GHRH], etc.), which stimulate or inhibit intermittent secretion of pituitary hormones. It appears that stimulatory or inhibitory effects of sleep on endocrine release are primarily associated with SW sleep, rather than REM sleep. Theoretically, the modulation of neuroendocrine release by sleep and circadian rhythmicity could be achieved by modulation of pulse amplitude, modulation of pulse frequency, or a combination of both. The data available so far seem to indicate that circadian rhythmicity of pituitary hormonal release is achieved primarily by modulation of pulse amplitude without changes in pulse frequency, whereas sleep-wake and REM/non-REM transitions affect pulse frequency. Pituitary hormones that influence endocrine systems not directly controlled by hypothalamic factors probably mediate, at least partially, the modulatory effects of sleep and circadian rhythmicity on these systems (e.g., counterregulatory effects of growth hormone [GH] and cortisol on glucose regulation).
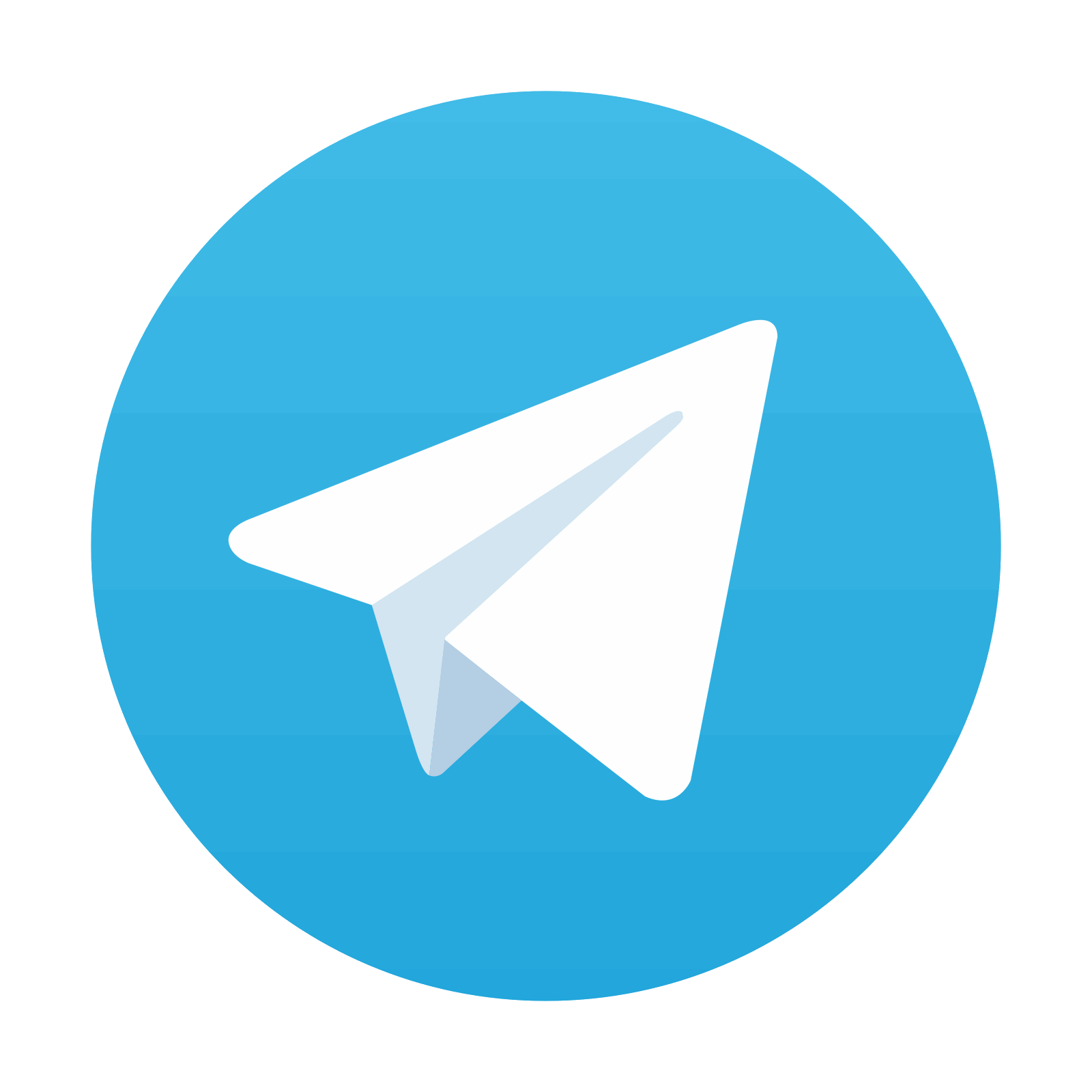
Stay updated, free articles. Join our Telegram channel

Full access? Get Clinical Tree
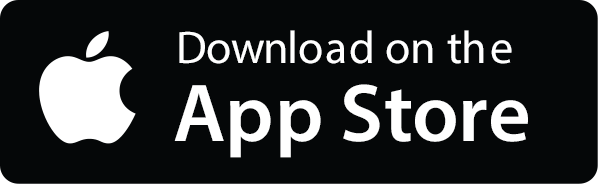
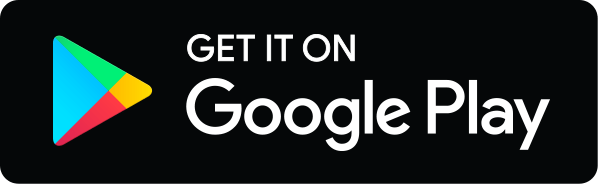