Cardiac complications
Michael S. Ewer, MD, MPH, JD, LLM, MBA Steven M. Ewer, MD
Thomas Suter, MD
Overview
Patients with malignant diseases often have coexisting cardiovascular disorders or may face serious cardiovascular complications in the course of their disease. The disorders may result from underlying conditions such as atherosclerosis, hypertension, or valvular abnormalities, or they may result directly from cancer or its treatment (Table 1). In addition, cardiovascular disorders that are unusual in patients not afflicted with cancer may be more common in the cancer patient and sometimes are unsuspected. Furthermore, cardiovascular diseases common in the general population must not be overlooked in patients with cancer; the presentation of such entities may be unusual, and the diagnosis often is more complex. Increased clinical scrutiny is therefore necessary in this vulnerable population. Multiple clinical problems may also coexist and defy a simple illumination because of the complex interactions between the malignancy, its treatment, and the cardiovascular system; what affects one often alters the presentation and course of the others. This chapter will look at some of the more common cardiovascular complications of cancer and its treatment, and will also address some of the dilemmas encountered in cancer patients with concomitant cardiac conditions.
Table 1 Important cardiovascular complications of cancer
|
Evaluation of the cardiovascular system in the cancer patient
The evaluation of the cardiovascular system in patients with cancer begins with a detailed history and a complete physical examination. Signs or symptoms suggestive of heart failure, dysrhythmia, ischemia, or pericardial disease—all of which are common in the cancer patient—trigger a more rigorous cardiovascular assessment.1 The individual approach should be targeted to include the specific clinical entities that are enumerated in Table 1. If clinical assessment of the patient indicates possible cardiac disease, further evaluation may include an electrocardiogram, chest X-ray, and echocardiogram. Additional imaging techniques or invasive modalities such as coronary angiography and electrophysiologic evaluation may be required for specific instances. The cardiac ultrasound or echocardiogram has enjoyed the widest usage in evaluating cancer patients. It plays an important role in evaluating cardiac contractile dysfunction and assessing the integrity of cardiac structures.2 A complete echocardiographic evaluation involves several complementary techniques, each of which provides information about cardiac abnormalities from a different vantage point. Transthoracic two-dimensional echocardiography images large regions of the heart, and in some views, all four cardiac chambers can be seen and wall motion abnormalities reflecting regional or global dysfunction can be assessed. Localized or loculated pericardial effusions as well as primary and metastatic tumors can be appreciated using two-dimensional echocardiography although other imaging modalities may also supplement and reinforce information initially obtained from the cardiac ultrasound.3 In situations where ultrasound imaging is suboptimal, echo contrast agents may be employed to augment the echocardiographic interface. Such agents are increasingly used and are generally regarded as safe.4
Spectral and color flow Doppler studies, which are performed routinely as part of a cardiac ultrasound evaluation, show the direction and velocity of blood flow in the cardiac chambers and across the valves. This information is graphically depicted and is often superimposed on the two-dimensional echocardiogram. Valvular hemodynamics, intracardiac shunts, turbulent blood movement, and abnormal direction of blood flow are best evaluated using Doppler studies. Doppler studies provide important information regarding diastolic function, an aspect of cardiac physiology that has become increasingly important. Strain (deformation) echocardiography provides information regarding active and passive repositioning of myocardial segments. While these newer techniques improve the predictive value of the ultrasound examination, their role in the routine management of cancer patients is evolving.5 Transesophageal echocardiography provides a higher resolution view of certain cardiac structures than does the transthoracic study. Although the technique is semi-invasive, the increased diagnostic sensitivity of the transesophageal studies often offsets this disadvantage. Transesophageal studies are especially useful in identifying vegetations and other valvular lesions or myocardial involvement of cancer, which may be extremely difficult to assess on transthoracic studies (Figure 1). The transesophageal study, in part because of the position of the probe, is an important adjunct for the evaluation of posterior accumulations of pericardial fluid, as may be seen post-operatively; they are also of considerable value in defining and monitoring intracardiac masses and thrombi (Figure 2).6 Intraoperative transesophageal echocardiography is helpful in documenting the extent of an inferior vena caval tumor as well as the result of resection of such tumors (Figure 2).5 Three-dimensional cardiac ultrasound now is available in many centers and provides enhanced information regarding spatial relationships of intracardiac structures including the cardiac valves. The technique allows the two-dimensional image to be rotated around a selected axis, providing images of improved special orientation.6
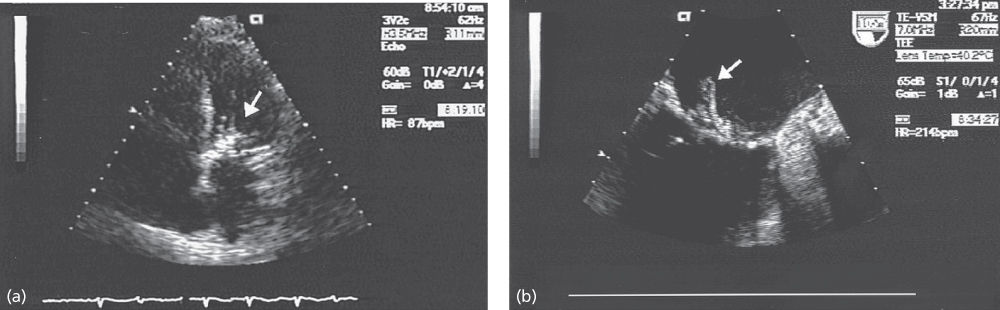
Figure 1 Echocardiogram frames of a patient with infective endocarditis involving the mitral valve. (a) Transthoracic 5-chamber image from a patient with suspected infective endocarditis involving the aortic valve. Note significant thickening of the aortic cusps. (b) Transesophageal image from the same patient recorded the same day, more clearly showing the bulky aortic valve vegetation.
Nuclear imaging techniques provide important information concerning both cardiac function and evaluation of ischemic heart disease. The multigated (MUGA) cardiac blood pool scan remains a common assessment tool for following left ventricular ejection fraction in patients being treated with agents known or suspected of being cardiotoxic. Nuclear imaging techniques may have a lower intra- and interobserver variability than do echocardiographic assessments. The technique requires electrocardiographic gating that can be problematic in patients with dysrhythmia; in addition, MUGA scans acquire data over a period of several minutes, and therefore depend on patients being able to remain immobile during acquisition. Once the imaging data is acquired, it provides an estimation of ejection fraction, information regarding wall-motion abnormalities, and parameters of cardiac relaxation (diastolic function). While MUGA scans have previously been used to assess cardiac function in large oncologic clinical trials, the improvement in cardiac ultrasound imaging and the concerns regarding radiation exposure are providing an impetus toward the wider use of ultrasound in following patients both in and out of clinical trials. Regardless of the method used, it must be emphasized that the results of any estimation of cardiac function are affected by many noncardiac factors, and that alterations in cardiac function for a specific patient must be interpreted with some caution.7 Furthermore, it should be noted that small changes in the left ventricular function (LVEF changes of 10% points or less) frequently reflect conditions not associated with the cancer or its treatment but rather by physiological variations of cardiac function or by unrelated conditions such as metabolic state, anemia, or other conditions.
Magnetic resonance imaging (MRI) can delineate intracardiac pericardial, and great vessel anatomy, and can delineate intracardiac and pericardial masses.8 Rapid-acquisition MRI, MR angiography, and contrast-enhanced MRI techniques, while not having usurped cardiac ultrasound, are assuming a broader role in the routine evaluation of cancer patients.9, 10 To some extent, it now can be considered the new gold standard for quantification of ventricular volumes, function, and mass.11 The cost of MRI remains considerable, and acquisition times are problematic for some patients; the technique is increasingly utilized to estimate ejection fractions for patients who cannot be otherwise evaluated. Cardiac computerized tomography is increasingly being used as an imaging modality and, like MRI, allows for excellent visualization of the pericardium and nearby extracardiac structures. Positron emission tomography (PET) has theoretical advantages over more traditional imaging techniques and is increasingly used to evaluate myocardial viability in cancer patients.12
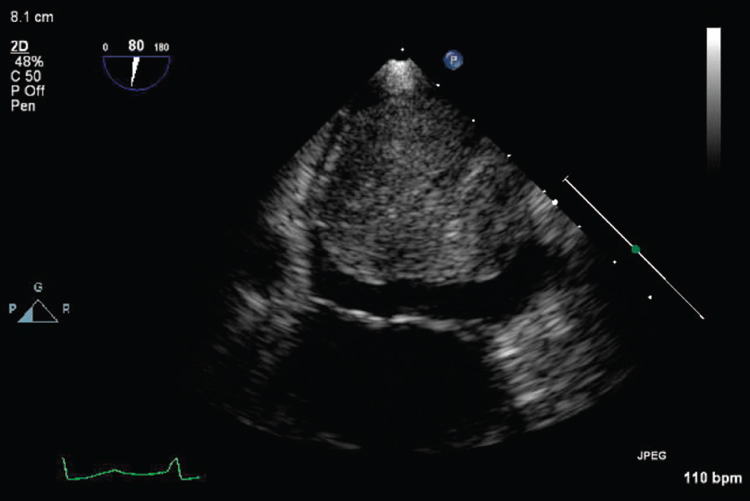
Figure 2 Transesophageal echocardiogram demonstrating a large left atrial mass in a patient who presented with angina. Echocardiographic features were consistent with tumor, and pathology was consistent with a pleomorphic myxoid sarcoma.
Metastatic involvement of cardiac structures
Metastatic involvement of cardiac structures is common and is seen in approximately 8–10% of patients with cancer; the incidence is somewhat less in elderly patients.13 Involvement may constitute an incidental finding at autopsy or may be the initial catastrophic presentation of cancer. There are wide variations among primary disease sites and tumor types. Newer imaging techniques have made it possible to recognize cardiac involvement much earlier than was previously possible, often at a time when intervention can be efficacious. Tumor spread to cardiac structures may be by direct invasion (i.e., lung or esophagus), by retrograde lymphatic spread (i.e., lung and breast), or by hematogenous seeding (i.e., melanoma, leukemia, or lymphoma). In view of the relatively high incidence of lung and breast cancers, these neoplasms are the most common primary sites of metastatic lesions to cardiac structures. Malignant melanoma, once metastatic, is particularly likely to involve cardiac structures.14 While spread to the pericardium is much more common than is spread to other cardiac structures, metastatic involvement has the potential to involve myocardial and endocardial sites. The heart, therefore, should be included in routine examinations that seek metastatic involvement in patients with Hodgkin and non-Hodgkin lymphomas, leukemias, gastrointestinal, gynecologic cancers (especially ovarian), multiple myeloma, and sarcoma (Figure 2).15 In addition, renal cell cancer may spread to the inferior vena cava and extend into the right atrium and right ventricle; these lesions often are amenable to surgical resection.
Pericardial involvement
Pericardial effusion
Pericardial effusion in cancer patients may be malignant or nonmalignant in nature and may be related to the tumor, its treatment, or to underlying cardiac or systemic disease. Malignant pericardial effusion is defined as an effusion associated with pathologic evidence for tumor invasion of the pericardium. A malignant pericardial effusion, however, may still be present even when malignant cells are not demonstrable by routine cytologic examination of the fluid. Pericardial effusion is also seen following radiation, anticancer treatment, lymphatic obstruction, altered oncotic balance, or infection. Fluid may demonstrate considerable variability with regard to both the quantity of fluid that accumulates and the pressure exerted on the pericardium and the cardiac chambers. The rate of accumulation and the distensibility of the pericardial sac determine the hemodynamic effect and the symptoms of these effusions.16 As little as 100 mL of fluid may cause symptoms in a patient with a scarred or infiltrated nondistensible parietal pericardium, whereas large effusions containing as much as 1 L may remain relatively indolent when the pericardial sac is elastic and the effusion accumulates gradually. The finding of malignant pericardial effusion generally implies a poorer prognosis.17
Generally, pericardial fluid is not static but is in equilibrium with other body fluids. Abnormal fluid build-up occurs when fluid enters the pericardial sac more rapidly than can be reabsorbed. This disequilibrium may occur when the efferent lymphatic vessels are obstructed, or when subcarinal lymph node metastases mechanically prevent effective drainage. Malignant effusions are usually serosanguinous or frankly bloody and often (but not always) contain cytologically identifiable cancer cells. When chylous effusions are malignant, the most likely cause is lymphoma; chylous effusions also have been reported following radiation for gynecologic malignancy.18
The onset of symptoms in patients with malignant pericardial effusion may be insidious. Indeed, many patients with large effusions are totally asymptomatic, the effusion only suspected when cardiac silhouette enlargement is noted on the chest radiograph. Decreased mean electrocardiographic QRS voltage also suggests a pericardial effusion, but other causes of decreased voltage are common in cancer patients, making this finding less useful; a recent drop in voltage should raise suspicion of the presence of pericardial effusion. Pericardial effusions are often first discovered as incidental findings on ultrasound, radionuclide, or other cardiac imaging studies, with the fluid appearing as a relatively inactive area separating the cardiac from the hepatic and pulmonary blood pools. Occasionally, a rocking motion of the heart suggesting hemodynamic compromise (cardiac tamponade) is noted. Computed tomographic (CT) images of the chest also may demonstrate pericardial effusions but are not especially helpful for estimating the fluid volume. CT of the chest is frequently used to evaluate pulmonary or mediastinal tumor involvement, and therefore may provide the first indication of an unsuspected pericardial effusion. Once suspected, the diagnosis is usually confirmed by echocardiography, and its progression or resolution may be followed with serial studies.19–21
Cardiac tamponade
The accumulation of pericardial fluid may lead to an increase in global or localized intrapericardial pressure and compromise cardiac output (tamponade).22 The symptoms include dyspnea and exertional intolerance; signs include hypotension, tachycardia, neck-vein distention, hepatomegaly, and cardiogenic shock. Heart sounds are often, but not always, distant and difficult to auscultate, and pericardial friction rubs may or may not be present. Vague chest discomfort or fullness is frequently noted. Most patients with significantly increased intrapericardial pressure also demonstrate an exaggeration of the decrease in pulse pressure during inspiration; when the systolic blood pressure decreases more than 10 mm Hg with normal inspiration, pulsus paradoxus is deemed to be present. A highly characteristic finding of cardiac tamponade is electrical alternans, whereby the electrocardiographic QRS voltage becomes larger and smaller on alternate complexes. This phenomenon is caused by physical movement of the heart toward and away from the electrode as the heart rocks back and forth within the fluid-containing pericardial sac. While electrical alternans is not always seen in cases of cardiac tamponade, when present it is a very helpful finding. Cardiac tamponade can almost always be diagnosed on the basis of physical findings and noninvasive studies. Echocardiography confirms the diagnosis and characterizes the extent and location of accumulated fluid, which may vary considerably. Systolic inward motion (collapse) of the right atrium is a sensitive but not specific finding; collapse becomes more specific when it extends for more than one-third of the cardiac cycle. Diastolic collapse of the right ventricular wall becomes more pronounced as hemodynamic compromise progresses and is a much more specific finding. A dilated, incompressible inferior vena cava is uniformly present in the setting of tamponade. Doppler flow studies show exaggerated respiratory variation in aortic outflow and mitral inflow velocities. Cardiac catheterization is usually not required to confirm the diagnosis but may show a graphic representation of pulsus paradoxus, and elevation and equalization of the diastolic pressures in the cardiac chambers ensues as tamponade progresses. With tamponade, the pulse becomes weak or totally absent during inspiration, and patients develop symptoms of low-output cardiogenic shock. Death, sometimes preceded by profound bradycardia, may ensue if tamponade is not resolved promptly.
Management of malignant pericardial effusion and pericardial tamponade
The management of malignant pericardial effusion depends on a number of factors, including the likelihood of the tumor responding to local (surgical, radiotherapeutic, or intracavitary) or systemic anticancer therapy; the extent of and the symptoms attributable to the effusion; and the overall anticipated survival of the patient.23, 24 Patients with tumors highly likely to respond to the systemic therapy may proceed with their treatment; sometimes, the malignant effusion resolves in responses to the systemic anticancer therapy alone. Pericardial effusion diagnosed in patients with tumors unresponsive to treatment may require local intervention. In patients who have a more favorable oncologic prognosis and who are sufficiently strong to undergo general anesthesia and surgery, creation of a pleuropericardial window is both effective and generally considered the procedure of choice. Although the transthoracic and the subxyphoid approaches are equally efficacious, in-hospital mortality was significantly greater for patients treated with the subxyphoid approach.25, 26 Using either approach, the communication formed usually remains patent, and the larger surface area available in the pleural space permits more effective reabsorption of the excess fluid. Pericardial needle drainage may be required before surgery as a stabilizing measure in patients who have very large effusions or evolving tamponade. Symptoms often resolve dramatically after removal of fluid, allowing patients to again engage in activities that had become impossible.
Needle drainage is now almost always undertaken with either echocardiographic or fluoroscopic confirmation of the position of the draining catheter, whereby newer instrumentation using smaller penetrating devices and improved imaging techniques have lowered the pericardiocentesis risk.27 Some patients experience transient left ventricular dysfunction after resolution of cardiac tamponade, and thus, a period of careful monitoring is important.28 The advantages of the pericardial window over percutaneous pericardiocentesis have not been fully evaluated, but some studies suggest little advantage to the more invasive pericardial window procedure. The advisability of routine drainage of large pericardial effusions in patients without tamponade has also been questioned; Merce et al. point out the low diagnostic yield and the lack of therapeutic benefit.29 The clinical management of such patients should be determined by their overall performance status, oncologic prognosis, and the expertise at the treatment center.
Sclerosis may be considered following needle drainage but is now employed much less frequently than heretofore, in part due to the significant patient discomfort associated with this procedure. A number of sclerosing agents have been studied, including hyperosmolar glucose, radioactive gold, bleomycin, sterile talc, doxycycline, and triethylenethiophosphoramide (thio-TEPA®).30, 31 Doxycycline, used at a dose of 250–500 mg, has received considerable attention as an effective sclerosing agent.32 In many instances, daily draining the fluid until the residual effusion is <50 mL without instilling a sclerosing agent is highly effective in preventing recurrent accumulations.33 Balloon pericardiotomy has been largely replaced by surgical pericardiotomy, an intervention that can often be accomplished with minimal invasion.34
Metastatic involvement of the myocardium
The spread of malignant tumors to the myocardium is being increasingly recognized in vivo through the broader use of imaging studies. While previously myocardial involvement was most commonly found at autopsy, this is no longer the case. Many patients in whom metastatic disease to the myocardium is identified before death also have evidence of concomitant pericardial involvement.
The most dramatic manifestation of myocardial metastatic disease is sudden dysrhythmia.35, 36 Sudden cardiac death can occur in this setting but is unusual. Cardiac perforation and erosion of the coronary vessels with hemorrhage or infarction may also occur but are exceedingly rare. More commonly, patients with myocardial involvement demonstrate signs of loss of functioning muscle mass and present with progressive shortness of breath or exercise intolerance; a decreased ejection fraction may be seen. The electrocardiographic representation of loss of electrical potential can be seen and may be indistinguishable from changes typically encountered with myocardial infarction due to coronary occlusion (Figure 3). ST segment elevations, T-wave inversions, or Q waves may be seen in such cases, even when the coronary arteries are normal angiographically.37
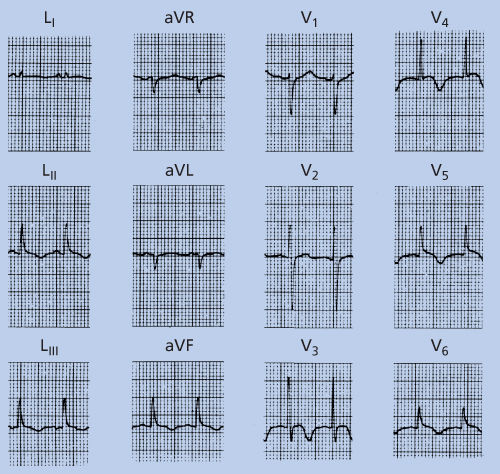
Figure 3 Electrocardiogram suggesting ischemia in a 26-year-old man with documented (by magnetic resonance imaging) myocardial metastatic disease and no history to suggest other causes of the electrocardiographic abnormality. Note T-wave inversions in the inferior leads (2, 3, and AVF) as well as in the precordial leads (V4–V6).
The diagnosis of metastatic involvement of the myocardium may be difficult to establish. A high suspicion may prompt special imaging, and MRI studies may be helpful in determining the presence and extent of metastatic myocardial disease. Although treatment of metastatic disease to the myocardium often is supportive, large lesions or those with impending valvular obstruction should be considered for surgical removal.38, 39
Cardiac effects of mediator release, high output states, and infiltrative disorders in cancer patients
Metabolically active mediators commonly are associated with some forms of neoplastic diseases and frequently are the immediate cause of a patient’s presenting signs and symptoms.40 Such mediator-associated diseases, or paraneoplastic syndromes, may have a direct or indirect effect on the cardiovascular system.
Carcinoid heart disease
Carcinoid tumors arise from enterochromaffin cell-derived neuroendocrine tissue, most commonly in the gut or lungs; oncologic considerations regarding these tumors are considered elsewhere in this text. Carcinoid heart disease results from prolonged release of biologically active mediators from the tumor that stimulate the formation of a distinctive fibromuscular plaque that destroys the integrity of the cardiac valves.41–46 Carcinoid heart disease is seen most commonly in patients who have ileocecal carcinoid tumors that have metastasized to the liver. Rarely, it occurs in patients with bronchial or ovarian carcinoid tumors, and, if outside the confines of the portal venous system, carcinoid heart disease may develop in the absence of hepatic metastases. The exact mechanism of plaque formation and cardiac injury remains elusive, but a number of possible mediators, including kinins, serotonin, 5-hydroxytryptophan, histamine, and prostaglandins, have been suggested; another as yet unidentified compound or combination of compounds may also contribute.47
Mediator release into the hepatic vein from metastatic liver disease predisposes patients to right-sided cardiac lesions. Because these mediators are eliminated by the lungs, the left heart is spared unless a right-to-left shunt is present. The lesions generally appear along the intima of the great veins, the right atrium, and the coronary sinus. The margins and distal (ventricular or downstream) aspect of the tricuspid leaflets are often thickened, and the chordae tendineae may also be involved. The pulmonic valve may be thickened and retracted. The damage appears to be aggravated by turbulent blood flow, which explains the characteristic location of the lesions. When the primary tumor or metastasis is in the lung, the mediators are released directly into the pulmonary venous bed and bypass the inactivating properties of lung tissue; left-sided valvular lesions are seen in such cases.48 Left-sided lesions are less frequent than right-sided ones but are more likely to result in hemodynamic compromise. A review of surgically excised valves noted considerable variation in the histological appearance of the material.49–51
The most important consequence of carcinoid plaques is thickening and fibrosis of the valves with resultant distortion of the valvular apparatus and ring. Tricuspid regurgitation and pulmonic stenosis are typically seen, but in the case of progressive destruction, a rigid tricuspid valve with hemodynamic abnormalities reflecting both stenotic and regurgitant characteristics may be encountered. Significant pulmonic regurgitation is rare. Stiffening of the right atrium may be noted and contributes to the neck-vein distention commonly seen in patients with the carcinoid syndrome. A high-output state, probably due to mediator release, has also been described.52
The clinical manifestations of carcinoid heart disease vary considerably. Some patients are able to tolerate the hemodynamic consequences of their valvular lesions well, whereas others develop symptoms early; this is especially so in the elderly or those with predisposing cardiac abnormalities.53 Early symptoms include fatigue, dyspnea on exertion, and palpitations owing to a high-output state, dysrhythmias, or to both. Later, symptoms of right-sided congestive heart failure, including edema, hepatomegaly, and ascites, predominate. Cardiac murmurs often predate symptoms, and the murmur of tricuspid regurgitation may be an early finding. Most frequently, the murmur is appreciated as a loud, holosystolic, blowing sound heard along the left lower sternal border. The murmur may be augmented during inspiration. The murmur of pulmonic stenosis cannot always be distinguished from that of the often coexisting tricuspid regurgitation; when heard, the pulmonic murmur is usually harsher and is appreciated most prominently in the second left intercostal space.
The chest radiograph may reveal prominence of the right ventricle. Unlike congenital pulmonic stenosis, which often includes poststenotic dilatation of the pulmonary trunk, this finding is usually not seen with carcinoid heart disease. Electrocardiographic findings include changes suggestive of right ventricular volume or pressure overload with or without right atrial abnormalities, right ventricular hypertrophy, right bundle-branch block, and/or right axis deviation; low voltage in the standard (limb) leads may also be seen.
Echocardiography is the most useful noninvasive tool for diagnosing carcinoid heart disease (Figure 4). It not only identifies the valvular abnormality, but, when coupled with Doppler ultrasonography studies, also provides hemodynamic data for estimating the degree of valvular involvement.54 Along with the thickening and loss of mobility of the tricuspid leaflets, increased flow velocity across the tricuspid valve during diastole is often evident. A regurgitant jet can be seen in the right atrium during systole. Furthermore, echocardiography can quantify the right atrial and ventricular enlargement that is characteristic of this condition. When of sufficient size, echocardiography is helpful in recognizing metastatic carcinoid tumors that involve the heart.55
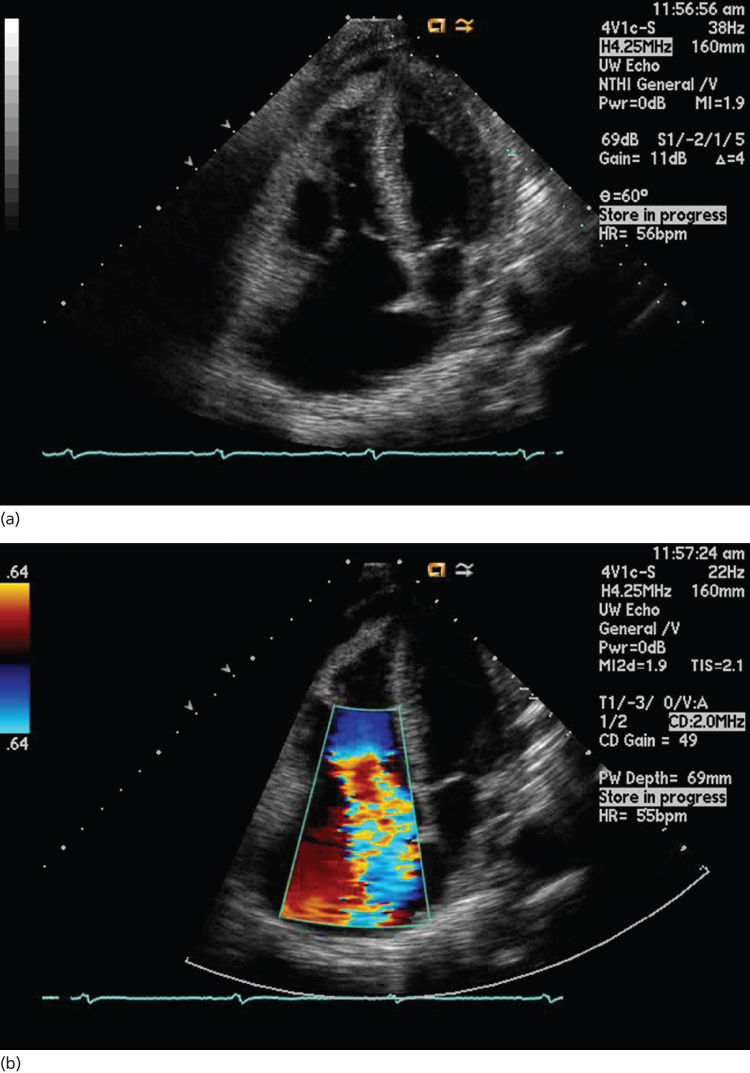
Figure 4 Carcinoid heart disease: (a) two-dimensional four-chamber study showing the thickened retracted and mal-coapting tricuspid valve leaflets. (b) Image from the same study with color-flow Doppler views showing severe tricuspid regurgitation. Also evident is right atrial and right ventricular chamber enlargement and a small pericardial effusion.
The management of patients with carcinoid heart disease must be individualized and is often challenging. Although the carcinoid plaque is largely irreversible, controlling or eliminating the offending mediators can delay plaque progression. In this respect, treatment of the primary tumor or metastatic disease is crucial. Once the diagnosis is established, carcinoid heart disease is initially managed pharmacologically with diuretics, afterload reduction, and salt restriction; the benefit of β-adrenergic blockade is unproven. Surgical intervention in the form of valvuloplasty or replacement is being considered more frequently than heretofore and with improved outcome, and some suggest early intervention.44
High-output states and high-output cardiac failure
Increased cardiac output occurs in many cancer patients and is most commonly due to anemia, hyperthyroidism, the syndrome of inappropriate antidiuretic hormone secretion, or the shunting of blood through tumors. High-output states are relatively common in patients with multiple myeloma.56 In addition, liver disease (nutritional cirrhosis or infectious hepatitis), fever, emotional excitement, and hypoxemia are also common causes of increased cardiac output and hyperdynamic states. High-output states are seen as well following treatment with a number of biologic response modifiers, including the interferons (possibly related to fever and the influenza-like reaction) and interleukins, in which case the phenomenon usually is of short duration.
High-output states are associated with a moderately increased heart rate (usually 85–110 beats per minute, but sometimes higher) and with increased stroke volume. Physical examination typically reveals neck veins of normal appearance as right-sided pressures are generally not increased. Peripheral pulses, however, are often bounding and have a rapid upstroke and fall; systolic blood pressure is elevated, and diastolic blood pressure often is reduced. Auscultation may reveal a systolic murmur and demonstrate a presystolic (S4) gallop. Pulmonary congestion is not uncommon in severe hyperdynamic states.
Echocardiography or radionuclide imaging is helpful in establishing the diagnosis. Two-dimensional ultrasound studies show increased wall motion in all views. In extreme cases, the images appear to suggest an almost total obliteration of the left ventricular cavity during systole; the ejection fraction is increased. Doppler examination reveals uniformly increased flow across all four valves. MUGA scans may also offer important data concerning the left ventricular ejection fraction and cardiac output. Right heart catheterization with the measurement of cardiac output confirms the diagnosis, but the procedure is rarely justified. It is important not to confuse this clinical picture with that of the more commonly encountered low-output congestive heart failure, with which it shares a number of characteristics. The treatment of high-output states should be directed toward the underlying cause; in the cancer patient, metastatic disease with or without shunting, hyperthyroidism, hypoxia, anemia, and infection are the most common considerations. High-output states often respond to blood transfusion, diuretics, oxygen administration, or antipyretics. In selected cases, β-adrenergic blockers may be useful. Unless patients are symptomatic, the high-output state does not require specific therapy, and efforts should be directed at managing the underlying cause.
Cardiac amyloidosis
Amyloidosis, or the deposition of amyloid proteins, may occur in a variety of organs including the heart, and may be caused by a number of pathologic processes.57 Amyloid proteins are made up of fibrils consisting of anti-parallel beta-pleated sheets that deposit in the interstitial spaces and are resistant to proteolysis. They are formed from a wide variety of precursor proteins.57, 58 Clinically, significant cancer-related amyloidosis is encountered in patients with multiple myeloma and, rarely, in patients with Hodgkin lymphoma. The amyloid protein associated with these diseases is known as AL amyloid and is derived from light-chain immunoglobulin (both Igλ and Igκ). AL amyloid accumulates in the atrial and ventricular myocardium and leads to either a restrictive or less commonly a dilated cardiomyopathy. Endocardial deposition resulting in valvular abnormalities has also been described.
Clinically, patients with cardiac amyloidosis experience fatigue and show signs of decreased cardiac output. Dyspnea and edema are common symptoms, and anorexia, weight loss, and presyncope are also encountered. In addition to heart failure, atrial or ventricular dysrhythmias or cardioembolic events are reported. Physical signs include elevated jugular venous pressure, edema, hepatic congestion, ascites, hypotension, macroglossia, and periorbital purpura. Conduction abnormalities are seen and, when associated with low effective heart rates or low-output states, may be symptomatic; stress-precipitated syncope may be a precursor of sudden cardiac death.59, 60 Both systolic and diastolic function may be impaired. Restrictive cardiomyopathy can be difficult to distinguish from constrictive pericarditis clinically; even findings at cardiac catheterization are not always conclusive in establishing the correct diagnosis. The chest radiograph shows a normal-sized or slightly enlarged cardiac silhouette. When cardiac failure appears, pulmonary congestion or pleural effusion may be seen. Electrocardiographic findings can include decreased voltage, a pseudo-infarction pattern, conduction system abnormalities, and atrial arrhythmias.61 The echocardiogram is often helpful in suggesting the diagnosis of amyloidosis (Figure 5). Ultrasonic images may demonstrate a thickened septum and posterior wall with normal internal dimensions of the left ventricle; diastolic relaxation is often impaired. In addition, a spotted or stippled echogenic appearance is often seen, and pericardial effusion is not uncommon. Atria are enlarged and may show markedly reduced mechanical function, even in the absence of atrial dysrhythmias. The paradox of left ventricular hypertrophy on cardiac ultrasound with decreased electrocardiographic voltage should suggest cardiac amyloidosis.62 Antimyosin scintigraphy, showing left ventricular thickening and diffuse myocardial antimyosin uptake, has been reported to be highly suggestive of amyloid heart disease. Cardiac MRI may be a useful adjunct in diagnosing amyloid infiltration, as a characteristic pattern of late gadolinium enhancement helps distinguish amyloid heart disease from other cardiomyopathies.63
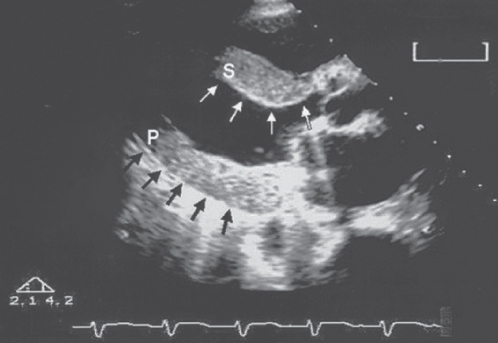
Figure 5 Long-axis echocardiographic view from a patient with cardiac amyloid infiltration. Note thickened septum (S) and posterior wall (P) with stippled appearance (arrows).
Cardiac catheterization demonstrates elevated intracardiac pressures in all chambers, with the minimum left ventricular pressure often increased to at least 10 mm Hg. The “dip and plateau” pattern seen on intraventricular pressure tracings in patients with constrictive pericarditis may be absent. Prominent papillary muscles are demonstrated with angiography. Endomyocardial biopsy with specimens stained with Congo red are helpful in confirming the diagnosis.64
The therapeutic interventions for cardiac amyloidosis are limited. Loop diuretics serve as the mainstay of medical treatment. Cardiac glycosides are dangerous in that they contribute to dysrhythmia; sudden death has been reported following their use.65 Beta blockers and non-dihydropyridine calcium channel blockers can exacerbate a low cardiac output state and angiotensin-converting enzyme inhibitors can precipitate profound hypotension. In instances where malignant dysrhythmia is triggered, implantable defibrillators have been offered, but current evidence does not support their use in amyloid cardiomyopathy.66 Clinical improvement may parallel control of the underlying process in patients with reactive forms of amyloidosis, and systemic treatment of myeloma may delay or alleviate the symptoms of myeloma-related cardiac amyloidosis.67 Intracardiac thrombosis is a significant risk in patients with amyloid infiltration. In one autopsy series, thrombosis was encountered in 33% of patients coming to autopsy. Anticoagulation in the presence of significant cardiac amyloidosis has been suggested. Therapy with a number of antineoplastic agents, including melphalan, cyclophosphamide, carmustine, and vincristine, have been attempted, as has hematopoietic stem cell transplantation.58 Restrictive cardiomyopathy due to light-chain deposition disease may, in rare cases, be reversible. Cardiac transplantation followed by autologous hematopoietic stem cell transplant has been successfully performed in selected patients.68
Cardiac dysrhythmia in the cancer patient
Cardiac rhythm disturbances are common in cancer patients. Dysrhythmias may be caused by the tumor, but more often, they are a consequence of anticancer treatment, the result of metabolic abnormalities, or from a reduced dysrhythmic threshold associated with underlying heart disease. The rhythm disturbances seen in cancer patients are morphologically and functionally identical to those seen in patients without malignancy. In some settings, it is essential to suppress a cardiac dysrhythmia vigorously, whereas in others, rhythm disturbances may be a transient manifestation of a temporary disturbance in homeostasis requiring little or no intervention. Many antineoplastic agents are associated with transient dysrhythmia, and because such rhythm disturbances are often asymptomatic, the phenomenon is not fully appreciated. Such chemotherapy-related rhythm disturbances generally are of short duration and little immediate clinical importance, yet they may be an indication of actual myocardial involvement that may become overtly manifest only at a later time. When associated with cancer treatment, most instances of dysrhythmia do not constitute an absolute indication to alter the chemotherapeutic regimen.
When faced with clinical decisions regarding which patients to treat, at what point in their management to initiate therapy, how long to continue treatment, and what form of therapy (pharmacologic or electrical) to use have been the focus of considerable debate. The wide assortment of antidysrhythmic drugs has not simplified these decisions. One useful approach is to distinguish dysrhythmias resulting from toxic substances or other metabolic abnormalities from those associated with structural abnormalities directly related to cardiac structures.36
Categories of dysrhythmia: primary (structural) versus secondary (metabolic)
The structural abnormalities within the heart that can result in cardiac abnormalities encompass a broad group of cardiac disorders. Ischemic heart disease, muscle hypertrophy, valvular disease, and infiltrative processes all fall within this group of disturbances. In cancer patients, tumor infiltration, cell loss following chemotherapy, pharmacologically induced ischemia, and fibrosis following radiation should also be included. Severe dysrhythmias, both supraventricular and ventricular, may appear with little warning and progress to hemodynamic instability and sudden cardiac death. Sudden death is more common in the presence of infiltrative processes such as amyloidosis.
Dysrhythmias in cancer patients also may be the result of nonstructural abnormalities. Most commonly, these include alterations in volume status, electrolyte disturbances, drug effects, and hormonal alterations, but other metabolic abnormalities that effect cardiac pacemaker and conduction tissue should also be considered.
Treatment of cardiac rhythm disturbances
Acute rhythm disturbances that result from metabolic abnormalities and that are not life threatening may be managed conservatively; in such cases, careful observation during treatment of the underlying abnormality may suffice. Active intervention is required when the dysrhythmia results in significant hemodynamic embarrassment, when the rhythm disturbance is likely to progress and become life threatening, or when a protracted rhythm disturbance is of the type that results in an increased likelihood of a thromboembolic event. Ventricular ectopy is seen commonly and ranges from isolated ventricular extrasystoles and benign accelerated idioventricular rhythm to malignant forms such as ventricular tachycardia or fibrillation. Coexisting conditions, such as fever or debilitation, which may augment tissue hypoxia, especially in anemic patients, predispose patients to ventricular ectopy. Unexpected death in cancer patients is usually attributed to dysrhythmia. Hemodynamic instability, regardless of the underlying cause, constitutes a medical emergency requiring the use of advanced cardiac life-support protocols.
Once the decision has been made to treat a patient experiencing dysrhythmia, the choice between pharmacologic intervention and the use of internal nonpharmacologic therapy such as implantable pacemakers or defibrillator or the use of ablation therapy generally follows the usual guidelines for these therapies. In difficult cases, electrophysiologic studies or pharmacologic threshold analysis may be useful. Implantable devices increasingly are being used in cancer patients; malignant disease per se should not be considered a barrier to their use, but should be balanced with the patient’s prognosis.
An ever-increasing number of drugs are being implicated in causing a well-recognized variant form of ventricular tachycardia known as torsades de pointes. This polymorphic ventricular tachycardia (Figure 6) is frequently preceded by a prolonged QT interval on the standard electrocardiogram. The QT interval is measured from the onset of the QRS complex to the end of the T wave and is then corrected for heart rate. This form of ventricular dysrhythmia is also associated with some antibiotics (erythromycin, clarithromycin, and pentamidine) psychotropic drugs (haloperidol), anti-emetics, some forms of high-dose chemotherapy, and bone marrow transplantation.69–71 Arsenic trioxide, which is most commonly used in the treatment of acute promyelocytic leukemia, also prolongs the QT interval, and patients should be observed carefully for QT prolongation during treatment with this agent.72, 73 Prompt recognition of this potentially malignant dysrhythmia and withdrawal of the offending agent may be the most appropriate therapy. Anecdotally, torsades de pointes may be more likely in African-Americans. QT prolongation has been reported to be more frequent in male patients as well as in those with hypokalemia. Serial monitoring of the QT interval is advised in high risk individuals.74 Table 2 lists the most important agents associated with QT prolongation.

Figure 6 Rhythm strip of a patient with episodes of torsades de pointes. A previous rhythm strip (not shown) demonstrated prolongation of the QT interval.
Table 2 Drugs associated with QT prolongation and known risk of torsades de pointes
Amiodarone | Halofantrine |
Anagrelide | Haloperidol |
Arsenic trioxide | Ibutilide |
Astemizole | Levofloxacin |
Azithromycin | Levomethadyl |
Bepridil | Mesoridazine |
Chloroquine | Methadone |
Chlorpromazine | Moxifloxacin |
Ciprofloxacin | Ondansetron |
Cisapride | Pentamidine |
Citalopram | Pimozide |
Clarithromycin | Probucol |
Cocaine | Procainamide |
Disopyramide | Propofol |
Dofetilide | Quinidine |
Domperidone | Sevoflurane |
Dronedarone | Sotalol |
Droperidol | Sparfloxacin |
Erythromycin | Sulpiride |
Escitalopram | Terfenadine |
Flecainide | Thioridazine |
Fluconazole | Vandetanib |
Grepafloxacin |
Adapted from the Arizona Center for Education and Research on Therapeutics.
Reference: https://crediblemeds.org/
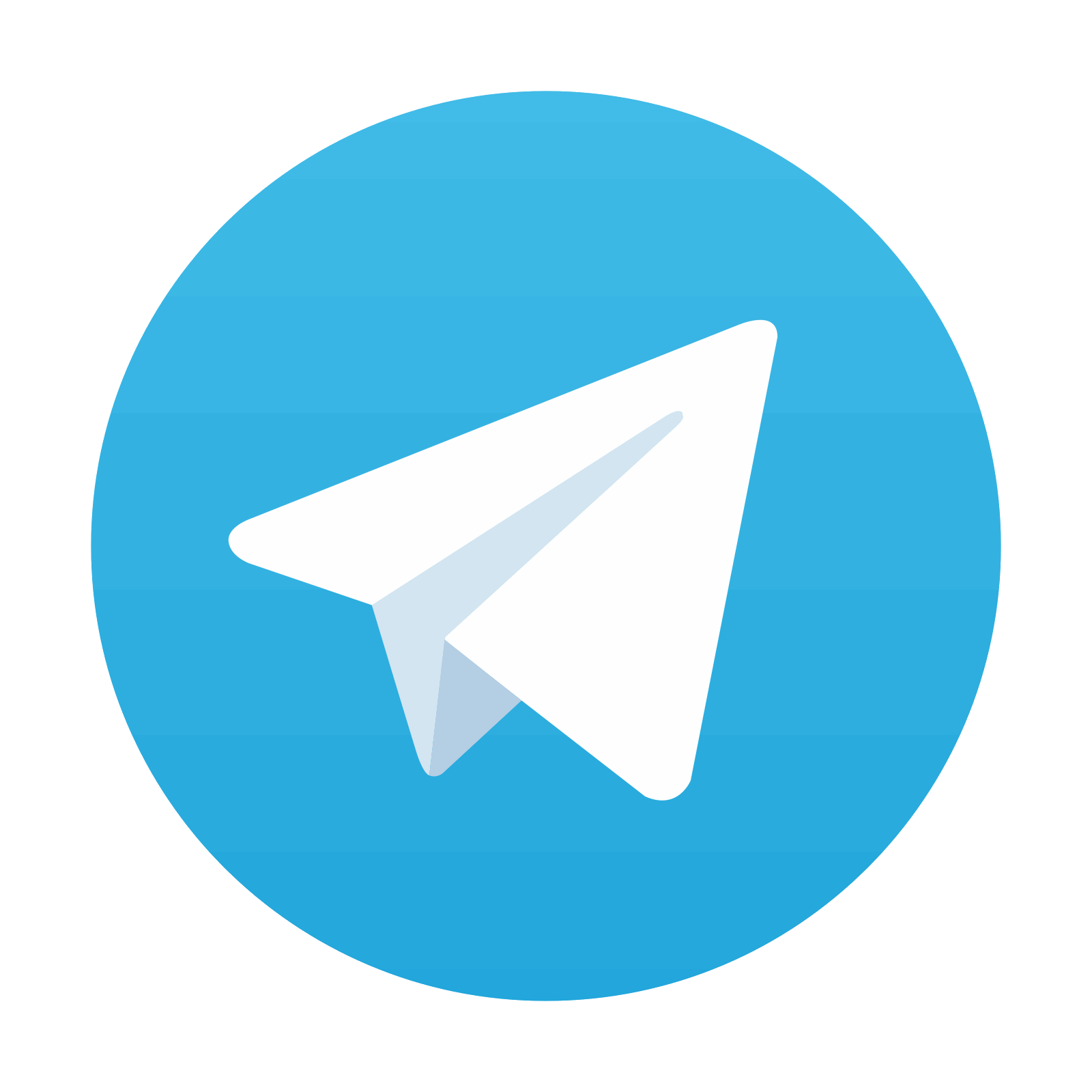
Stay updated, free articles. Join our Telegram channel

Full access? Get Clinical Tree
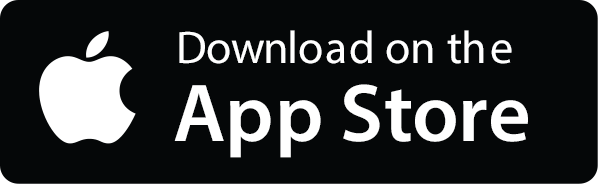
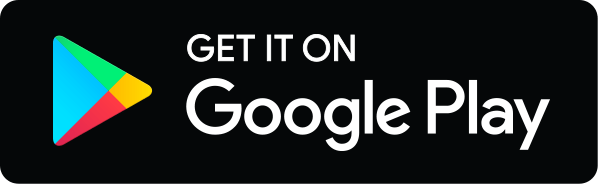