(1)
Medical Sciences Division Northern Ontario School of Medicine West Campus, Lakehead University, Thunder Bay, Ontario, Canada
Key Topics
Brast cancer (BrCa) screening
Molecular pathology of BrCa
Circulating BrCa biomarkers
Circulating BrCa cells and stem cells
Key Points
BrCa is a disease that has benefited from recent molecular genetic research, because of the well-characterized molecular pathology. For instance, despite the numerous histopathologic subtypes, molecular profiling recognizes only four relevant subgroups with prognostic and predictive potential. Gene expression profiling has uncovered clinically actionable sets of genes used in the Oncotype DX® and MammaPrint BrCa tests.
Noninvasive tests for BrCa hold potential for advancing acceptable disease detection and management. The detection and quantification of ccfDNA, epigenetic alterations (e.g., CDKN2A methylation), mutations (e.g., TP53), microsatellite alterations (e.g., D13S2118 alterations), mRNA (e.g., hTERT), ncRNA (e.g., miR-155), proteins, and metabolites (e.g., choline-containing compounds) in circulation should add to the arsenal of biomarkers needed to effectively manage the different BrCa subtypes.
Circulating BrCa cells (CBrCaCs) are established prognostic biomarkers of early and metastatic BrCa. Additionally, their enumeration and characterization are useful in monitoring for treatment efficacy and disease relapse. The ability to track CBrCaC clones with different genotypes from the primary tumor informs real-time treatment decision-making.
4.1 Introduction
Breast cancer (BrCa) is one disease that recent molecular and genetic research efforts have contributed immensely to the understanding of its pathology, and hence effective management. This feat has translated into improved survival outcomes. For example, the 5-year survival rate has improved from ~63 % in the 1960s to about 90 % in 2010. However, BrCa remains a disease of importance, being the second most common cancer in the world and the first and second most common cause of cancer-related deaths in the less and more developed parts of the world, respectively. Globally, 1.67 million cases were diagnosed and 522,000 people died from the disease in 2012. The 5-year global prevalence stands at 6.23 million. In the US, the expected incidence and mortality in 2016 are 249,260 and 40,890 cases respectively.
Early cancer detection offers the best opportunity for cure. For BrCa, the 5-year survival rate can be as high as 98 % for localized cancers, compared to a woeful 26 % rate for those diagnosed with advanced stage diseases. Late stage BrCa patients are treated with hormonal, chemotherapeutic, and biologically targeted agents, yet metastatic relapse occurs in a vast majority of cases, and this accounts for the poor prognosis. Also established in BrCa is the concept of field cancerization, which should enable the discovery of early detection biomarkers for BrCa.
Screening strategies, including mammography, have proven useful in early detection and improved survival of BrCa patients. Current screening efforts detect ~63 % of BrCas at an early stage. This is probably because mammography has limited utility in premenopausal women with dense breast tissue, and yet they contribute to about 12 % of all BrCas. Measurement of serum CA15-3 has also been helpful in the management of some women with metastatic BrCa. The need for novel accurate and validated biomarkers for early BrCa detection and management is hence acute, and there have been a plethora of biomarkers. But biomarkers in body fluids (circulation, breast fluid, urine, or saliva) should enable acceptable screening of all women at risk, especially premenopausal women and women in resource-poor communities of the world. Body fluid biomarkers will also fit into the ease of serial longitudinal sampling necessary for making important clinical decisions required for personalized BrCa oncology.
4.2 Screening Recommendations for BrCa
Screening for BrCa includes breast self-examination (BSE), clinical breast examination (CBE), and imaging primarily by mammography. A randomized case-control study concludes that BSE has limited value in early BrCa detection. However, because this is harmless, there is absolutely no reason why a woman should not occasionally examine her own breast or has her partner feel it for possible lumps. Breast self-examination should be performed monthly beginning at age 20. Clinical breast examination complements BSE and is useful in BrCa detection especially in younger women (≤50 years). However, in comparison to mammography, CBE is less sensitive at cancer detection. This may be due to the limited experience of some family physicians and gynecologists in breast examination techniques. Also the improved detection by mammography is mostly in older women with less dense breast tissue. Clinical breast examination is recommended to be performed annually for women 40 years and older in conjunction with mammography. Mammography is currently the best screening modality for early detection of BrCa. In postmenopausal women with less dense breast tissue, mammography can reduce cancer mortality by at least a third. The method is, however, inaccurate when used in premenopausal women with dense breast mass. It is still useful for the fraction of premenopausal women with less dense breast tissue, for whom it is recommended. Radiation exposure and cost consideration must be examined in the use of this technology in all younger women. Body fluid biomarkers will be very useful for this group of women.
4.3 Molecular Pathology of BrCa
BrCa is a very heterogeneous disease, with different histopathologic and molecular subtypes. Knowledge on the molecular differences of BrCa informs adoption of efficacious targeted therapies in disease management.
4.3.1 Histopathologic Classification of BrCa
Histopathologic classification relies on the cytoarchitectural origins of the cancer, organization of the cancer cells, as well as demonstrating the presence or absence of substances secreted by the cancer cells. The well-defined and used method is that by the WHO. The various types are briefly described below:
Carcinoma in situ comprises of neoplastic cellular proliferation that fills the lobular acini and ductules without breaching the underlining basement membrane. They are thus confined lobular and ductal lesions and can be completely cured, by surgery, if these are the only malignant cells in the breast. Histoarchitectural compartmentalization of the transformed cells identifies two classes of in situ BrCa. Ductal and lobular lesions are referred to as ductal carcinoma in situ (DCIS) and lobular carcinoma in situ (LCIS), respectively. Ductal carcinoma in situ is an impalpable lesion, but can be identified by mammographic screening as a cluster of microcalcification. Mammography often does not pick up LCIS. These are occult lesions diagnosed incidentally on biopsies performed for other concerns. Both lesions are risk factors for invasive BrCa, but DCIS can be a precursor of invasive disease.
Invasive or infiltrating lobular carcinoma constitutes ~10 % of all invasive BrCas. This is a very challenging carcinoma both from a complete diagnostic workout, as well as treatment perspectives because of the mode of stromal invasion. The cells tend to file into the stroma in linear fashion (Indian file pattern). The often diffuse nature and lack of distinct boundaries make it difficult to accurately estimate tumor size or offer lumpectomy as a surgical alternative. The extent of invasion is thus more accurately determined using immunohistochemical stains.
Invasive ductal carcinoma (IDC) accounts for the majority of BrCas, comprising between 60 and 80 % of all invasive BrCas. They are often associated with DCIS, partly explaining the belief that DCIS is a precursor lesion. They are further classified based on the degree of differentiation: being well differentiated (grade 1 tumors), of intermediate differentiation (grade 2 tumors), or poorly differentiated (grade 3 tumors). Approximately 10 % of IDCs are of special histomorphology and are thus diagnostically distinguished by special names. The vast majority are thus designated as “classic or not otherwise specified (classic/NOS).”
The special IDC types (with defined histomorphology) include mucinous or colloid carcinoma, which comprises up to 2 % of all invasive BrCa. They are so named because the cancer cells produce extracellular mucin. These tumors are often diagnosed in older women and have good prognosis. Medullary carcinoma comprises ~5 % of all invasive BrCas. They resemble benign fibroadenomas with well-demarcated borders. They are associated with younger age and have a better prognosis than classic/NOS IDC. Papillary carcinoma comprises up to 2 % of invasive BrCa. They grow in a papillary fashion with cystic and solid compositions. They may be intraductal or invasive and have better prognosis than classic/NOS IDC. Tubular carcinoma make up another ~2 % of invasive BrCa. The tumors are well differentiated with tubular or small acinar growth patterns that resemble normal breast architecture. They may be associated with intraductal carcinoma and are in general small lesions measuring just about 1 cm. Inflammatory carcinoma is a very aggressive subtype of IDC. It is an inflammatory lesion with skin indurations and subcutaneous lymphatic obstruction that pulls on the underlying connective tissue ligaments resulting in an orange peel texture referred to as “peau d’orange.” The remaining IDCs are very rare, comprising less than 1 % of all invasive BrCas. They include adenoid cystic carcinoma that contains cystic and glandular components and resemble adenoid carcinoma of the salivary glands and secretory (juvenile) carcinoma that occurs in children and young adults. These tumors secrete lots of α-lactalbumin. Apocrine carcinoma as the name suggests resemble cutaneous sweat glands. Metaplastic carcinoma is composed predominantly of non-epithelial neoplastic cells. They could be bone, cartilage, or undifferentiated spindle cells (pseudosarcoma). Cribriform carcinoma cells are arranged in cribriform pattern and have a good prognosis. Clear cell carcinoma is diagnosed by distinct staining and has poor prognosis. Paget’s disease of the breast is often associated with intraductal carcinoma with or without invasive components. Indeed, Paget’s disease of the breast is the spread of cancer cells from the underlining lobe along the lactiferous dusts and sinuses and into the epidermis.
4.3.2 Molecular Classification of BrCa
In the era of personalized oncology, molecular classification is important not only for prognostication but selection of patents for targeted therapy. There are a number of targeted therapies for BrCa. Thus, testing for ER/PR and HER2 status enables the deployment of the right treatment regimen. For example, tamoxifen and fulvestrant target ER-positive tumors, while trastuzumab and pertuzumab are useful in patients with HER2-positive tumors. There are other targeted agents that inhibit the PI3K (e.g., inhibition of mTOR with agents such as everolimus) and FGFR pathways.
Irrespective of the numerous and diverse histopathologic subtypes, molecular markers place all BrCas into just four subcategories. This is an important step because the vast majority (>80 %) of BrCa is histologically classified as IDC/NOS. Gene expression profiling and genomic analysis of chromosomal aberrations have enabled the molecular stratification of invasive BrCa into the four distinct subtypes (Table 4.1). They are HER2 enriched, luminal A, luminal B, and basal-like. Each subtype displays unique gene expression patterns as well as chromosomal abnormalities, which translate into treatment selection and prognostication.
Table 4.1
Features of the molecular subtypes of breast cancer
Tumors | Molecular features | Targeted therapy | Prognosis |
---|---|---|---|
Luminal A | ER positive, HER2 negative High-level amplifications at 8p11–12, 11q13–14, 12q13–14, 17q11–12, 17q21–24, 20q13 Gain at 1q, 16p Loss at 16q Express ESR1, PGR, GATA3 – consistent with ER status | Hormonal | Good |
Luminal B | ER positive, may be HER2 overexpressing High-level amplifications at 8p11–12, 8q, 11-q13–14 Gains at 1q, 8q, 17q, 20q Losses at 1p, 8p, 13q, 16q, 17p, 22q | Hormonal | Worse than luminal A, but better than HER2 and basal-like tumors |
HER2 | HER2 overexpressing, ER negative High-level amplification of 17q Amplifications of 1q, 7p, 8q, 16p, 20q Loss of 1p, 8p, 13q, 18q Expression of ERBB2, GRB7 (17q loci) | HER2-targeted agents such as trastuzumab, lapatinib | Worse than luminal subtypes |
Basal-like | Triple negative – ER, PR, and HER2 negative Locus amplification is uncommon Gains at 3q, 8p and 10p Losses at 3p, 4p, 4q, 5q, 12q, 13q, 14q and 15q Express CK5, CK17 (basal epithelial and myoepithelial genes) Loss of BRCA1 by methylation is common (hence share features with BRCA1+ve tumors) | No established targeted therapy, but sensitive to epirubicin–cyclophosphamide combination therapy | Worse than luminal subtypes |
The gene set that defines a group are those reminiscent of HER2-positive status, ER-positive status, breast cytoarchitecture (e.g., luminal and basal cells), or of the normal breast. For example HER2-enriched tumors express ERBB2, and GRB7, while luminal subtypes express ESR1, GATA3, and PGR, which are consistent with their HER2-positive and ER-positive status, respectively. Luminal tumors have a better prognosis than HER2-positive and basal-like tumors. But luminal B cases have worse outcome than luminal A. Similarly, basal-like tumors overlap considerably with triple-negative tumors and tumors harboring BRCA1 mutations, and they express basal cytokeratin (CK5/6/17). The triple-negative tumors are not all basal-like, and a refined classification is needed for this subtype. They are common in younger and African-American women and are associated with worse prognosis. On the therapeutic front, the small molecule tyrosine kinase inhibitor (lapatinib) and monoclonal antibody (trastuzumab) are efficacious in HER2-positive BrCas, while targeted hormonal therapies are offered for luminal tumors. Although there is currently no targeted therapy for basal-like tumors, they tend to be sensitive to epirubicin–cyclophosphamide combination therapy.
4.3.3 Clinically Relevant BrCa Transcriptomic Biomarkers
Global transcriptome profiling of BrCa is probably the best-illustrated example of the utility of “omics” data in cancer management. This area of research has received extensive discovery and valid biomarkers that are contributing to improved BrCa care. For instance, the molecular subtyping of invasive BrCa has important implications for objective management of this disease. In addition, validated gene sets that comprise the Oncotype DX® and MammaPrint BrCa tests have received FDA acceptance and are clinically available for companion diagnostic use. They both provide powerful prognostic and therapeutic predictions.
Oncotype DX® BrCa assay is a companion diagnostic test offered by Genomic Health. A carefully and rationally selected 21-gene set comprised of 16 BrCa genes and 5 control genes form this multigene predictive test. It interrogates the existing formalin-fixed and paraffin-embedded (FFPE) samples from patients using RT-PCR analysis, and in combination with a proprietary algorithm generates a recurrence score (RS) indicative of disease behavior and benefit from chemotherapy. The test, which is highly validated, is exempted by the FDA and endorsed by the American Society of Clinical Oncology® and the National Comprehensive Cancer Network® in making treatment decisions. The test is used for early stage (I and II), node-negative, hormone receptor-positive BrCa patients. Validated data indicate that patients at elevated risk of recurrence (those with high RS scores) benefit from adjuvant chemotherapy, while those with favorable prognosis (low RS scores) can just remain on hormone therapy and be spared the toxicities of adjuvant treatment.
Another personalized BrCa test in the clinic is MammaPrint, developed by Agendia. The 70-gene set that comprises this test were uncovered by scientists at the Netherlands Cancer Institute and has since then been well validated for stratifying early stage BrCa women into high and low risk for distant recurrence following surgery. MammaPrint is the first of these tests to be approved by the FDA in accordance with its IVDMIA guidelines. It is indicated for women with node-negative stage 1 or stage 2 invasive BrCa irrespective of ER status. Similar to Oncotype DX® BrCa test, MammaPrint identifies patients who will benefit from adjuvant therapy, because patients with predicted unfavorable benefit from such therapy are the low-risk category. Unlike Oncotype DX®, the MammaPrint® BrCa test requires high-quality RNA, either fresh frozen samples or those collected in RNA preservatives.
Other BrCa gene expression signatures with prognostic and predictive values include the HOXB13:IL17BR ratio (H/I index) and the 76-gene signature assays. The H/I index assay was discovered through global gene expression profiling of ER-positive early stage BrCa from women treated with adjuvant tamoxifen. The two genes were identified to be the best predictor of disease recurrence. It has received extensive independent validation studies involving multiple samples. High H/I ratio is associated with tumor aggressiveness, worse outcome, and tamoxifen failure. The performance of the assay is independent of tamoxifen treatment and is much better in early stage lymph node-negative tumors. The test, which is performed on FFPE samples, is offered by bioMérieux (bioTheranostics). The 76-gene signature was discovered by scientists at Rotterdam and has been validated as a potential strong prognostic indicator in premenopausal and postmenopausal women with ER-positive BrCa. This assay requires high-quality RNA for analysis (frozen or preserved in RNA protective reagents).
4.3.4 Molecular Model of BrCa Progression
Molecular data provide a not so distinct complex pathway of BrCa progression. This progressive model involves epigenetic alterations, mutations, and importantly chromosomal instabilities that are modified by individual genetic composition. The high level of BrCa heterogeneity precludes charting a simple and well-defined model as is in colorectal cancer. An even more complex issue is the evidence that the sorts and levels of genomic damage that drive BrCa also depend on the normal progenitor cell type of origin.
Hormonal stimulation, gene promoter hypermethylation, loss of proliferation control, increased cell cycle activity, lack of checkpoint control, loss of DNA repair mechanisms, loss of cell death control, telomerase expression, eroding telomeres (telomere crisis), high genomic instability, loss of tumor suppressor gene function through mutations and methylation, oncogene amplification, and growth factor secretion are among the factors that operate in concert to drive the normal breast epithelial cell to hyperplasia, through in situ carcinoma then to invasive carcinoma. Analysis of deletions, amplifications, and recurrent mutations reveal relevant pathways in BrCa progression, including the ERBB2/EGFR and PI3K signaling pathways.
In spite of the difficulties encountered in charting a path for BrCa development, it is recognized that ER status has distinct molecular pathology that is relevant to disease progression. Estrogen receptor-expressing tumors harbor deletions at 16q and gains of 1q, while ER-negative tumors have increased genomic instability and loss of BRAC1 and TP53, with amplification of HER2. With the demonstration that ER-positive tumors may progress from low-grade in situ to high-grade tumors, attempted progressive models are provided for ER-positive and ER-negative tumors (Fig. 4.1).
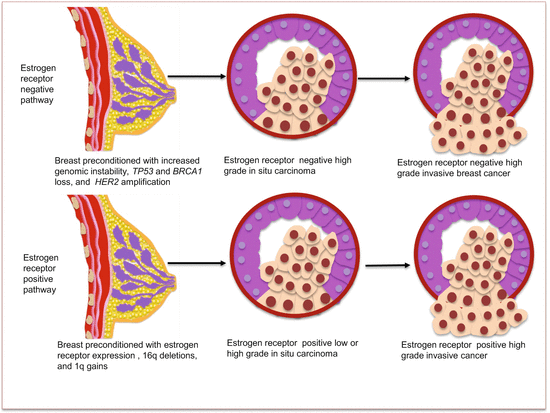
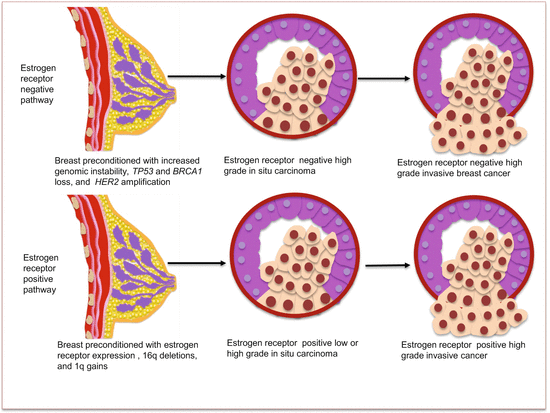
Fig. 4.1
BrCa molecular progression model based on estrogen receptor (ER) status
4.4 Circulating BrCa Biomarkers
There has been extensive pursuit in uncovering circulating BrCa biomarkers. This effort has led to the discovery of a plethora of molecular genetic alterations in women with BrCa. Validations of most of these biomarkers are awaited. However, circulating breast cancer cell characterization is in routine clinical practice.
4.4.1 Circulating Cell-Free Nucleic Acid Content as BrCa Biomarkers
Attempts have been made to uncover cell-free DNA as clinical biomarkers of BrCa. Serum analysis of DNA fragments of ALU repeats in women with primary BrCa and healthy female controls indicate a mean higher DNA integrity index (DII) in stages II, III, and IV cancer patients than controls with discriminatory AUROCC of 0.79. DII correlated with size of invasive cancer and was significantly higher in those with lymphovascular and lymph node metastasis. Detection of lymph node metastasis was accurate, with AUROCC of 0.81. In a multivariate analysis, lymphovascular invasion and DII significantly predicted lymph node metastasis, making this a promising biomarker for the detection of BrCa progression and lymph node involvement [1]. Sunami et al. analyzed the sera of BrCa patients for LINE1 DNA fragments to predict possible early detection of BrCa [2]. These fragments were increased in BrCa patients, and the copy number correlated with tumor size. Deligeze et al. proved that non-apoptotic DNA fragments contribute to the change in DNA levels during adjuvant chemotherapy of BrCa [3]. Larger DNA fragments released from non-apoptotic cells mainly contributed to the elevated DNA levels in circulation during adjuvant chemotherapy [4]. Another study compared ccfDNA with CTCs, CA15–3, and current standard of care (medical imaging) and found unequivocal evidence for the use of ccfDNA in BrCa monitoring [5]. Circulating cell-free DNA was demonstrated in 97 % of women. Concordance between tumor mutations and mutations in plasma were observed. Importantly, some mutations detected in plasma DNA were not found in archival tissue samples collected 10 years prior, indicating the presence of tumor evolution with time. For diagnostic performance, levels of ccfDNA performed at 96 % compared to the CELLSEARCH® system for CTCs that achieved 87 % and levels of CA15–3 that performed at 78 % in BrCa detection among these women. Increasing plasma levels of ccfDNA was a better prognostic indicator than conventional imaging assessment. The increasing ccfDNA levels were associated with poor overall survival (OS), and detected progressive breast disease 5 months before conventional imaging could do so, making this a much early detection assay.
4.4.2 Circulating BrCa Epigenetic Biomarkers
Alterations in the epigenome, especially promoter CpG island hypermethylation, are prevalent in BrCa samples. Although these tumor markers are yet to be clinically validated and translated, the promise to use these biomarkers in early detection and prognostication is not far from reality. Of even more clinically relevant is the sensitive detection of these cancer-specific epigenetic changes in minimally invasive samples including blood, nipple aspirate fluids, ductal lavage, and fine needle aspirates from patients. Several methylated genes detected in body fluids are potential biomarkers of early BrCa detection. Whereas these genes demonstrate variable sensitivities, they appear to be very specific (up to 100 %) for cancer. Promoter hypermethylation of several genes including RASSF1A, APC, CDKN2A, CDH1, CCND2, HIC-1, DAPK, GSTP1, SFN (stratifin), RAR-β, and TWIST occur at various frequencies in BrCa samples.
4.4.2.1 Circulating BrCa Diagnostic Epigenetic Biomarkers
As a proof of concept, Silva et al. were able to demonstrate that de novo methylated cancer genome could be detected in circulation of BrCa patients [6]. Exon 1 of CDKN2A/INK4A hypermethylation was detected in 23 % of tumors and specifically in 14 % of paired plasma samples. Rykova et al. demonstrated using methylation of RASSF1A and HIC-1 that cancer-derived circulating DNA is primarily cell surface bound (on RBCs and WBCs) [7]. In this study, detection of gene methylation was enhanced when DNA was eluted from cell surfaces (csb-DNA), and was even positive in samples that were negative for methylation in plasma. A follow-up study of methylation of RASSSF1A, RARβ2 and CCND2 indicated positive detection in 13 % of breast fibroadenomas, and in 60 % of women with BrCa. Using csb-DNA as template increased the detection of methylated genes to 87 % for adenomas and 95 % for BrCas [8]. But this assay shows no specificity for cancer, as it detects adenomas as well.
Serum was examined as a less invasive sample (compared with NAF/DL) for BrCa methylation biomarker discovery and utility. Three important genes involved in cancer biology (RASSF1A, APC, and DAPK) were selected for this study. Promoter hypermethylation in one or more genes was observed in 94 % of tumor DNA (RASSF1A at 65 %, DAPK at 50 %, and APC at 47 %) and 76 % of corresponding serum samples. These findings also included premalignant and early stage disease (DCIS, LCIS, and stage I) patients, attesting to the early detection potential. Specificity of this assay was 100 %, because all samples from control individuals (normal, inflammatory, and nonneoplastic breast disease patients) were negative [9]. Shukla and colleagues further investigated RASSF1A, RARβ2, and HIC-1 methylation in total ccfDNA, which indicated the absence of methylation in healthy controls but in as many as 95 % of BrCa patients as well as patients with benign breast disease (fibroadenomas at 60 %) [10]. The methylation status of RASSF1A and RARβ2 in invasive BrCa tissues and paired sera has revealed the presence of RASSF1A methylation in 85 % of breast tumors and 75 % of paired sera [11]. RARβ2 was methylated in just 10 % of tumor samples [10].
A study attempted to address an important need of accessible diagnostics for underserved population of women. It focused on BrCa from West African women, a geographic region where mammography and other sophisticated diagnostics are not readily available. Methylation of APC, GSTP1, RASSF1A, and RARβ2 in plasma from 93 women with primary BrCa and 76 controls were assessed. Cutoff values and gene panel selection were achieved using ROC curves in a training data set, and assay performance was tested on a validation set. A sensitivity of 62 % and specificity of 87 % was achieved. Importantly, 33 % of early stage cancers were detected by this serum assay [12].
In order to uncover an early detection biomarker for women at an above-average risk, a study from the BrCa family registry focused on RASSF1A methylation in plasma of BrCa women. Blood samples were collected prior to diagnosis, and cases were tightly matched with controls. Eighteen percent of plasma samples were positive for RASSF1A methylation, and two healthy high-risk women were also positive for methylation. None of the population-based healthy control plasma samples were positive. All available tumor samples were positive for RASSF1A methylation. This finding could serve as an early warning sign for this population at above-average risk for BrCa [13]. Another study targeting hypermethylation of RASSF1A and DAPK1 in sera from BrCa patients, healthy women, and those with benign breast disease found hypermethylation of at least one gene in 96 % of cancer, 43 % of benign disease patients, and 8 % of controls. RASSF1A and DAPK1 were methylated at frequencies of 69 % and 88 %, respectively, and both gene methylations were associated with ductal carcinomas [14].
The methylation status of other genes in circulation as diagnostic biomarkers of BrCa has been evaluated. The diagnostic value of APC hypermethylation was assessed in 84 women with BrCa. APC hypermethylation was detected in 45.2 % of tumors and 31 % of paired plasma samples. A statistically significant correlation existed between methylation in tissues and paired serum samples. The use of this serum assay as a single biomarker for BrCa detection performed at a sensitivity and specificity of 68.4 % and 97.8 %, respectively [15].
Sharma et al. attempted to find noninvasive diagnostic biomarkers for a population of Indian women with invasive BrCa. They initially examined CDKN2A/INK4A, CDKN2A/ARF, CCND2, and SLIT2 methylation in invasive BrCa and paired sera as a possible screening tool [16]. Methylation of at least one gene was observed in 86 % and 83 % of tumor and sera, respectively. Each gene was methylated at different frequencies, and there was concordance between methylations in tissue and sera. In another study by this group [17], promoter methylation of the following tumor suppressor genes, TMS1, BRAC1, ERα, and PRB, was evaluated in samples from a population of Indian women where invasive BrCa is engulfing younger women and often with poor prognosis (ER-/PR-negative tumors). Methylation in at least one gene was present in 72 % of tumors and 64 % of paired sera. Methylation of three genes could be detected in as many as 34 % of tumors and 24 % of associated sera. Methylation in tumor and sera for each gene was concordant, and methylation of ERα and PRB were significantly correlated. A follow-up study examined methylation of SFN, ERα, and PRB in tumor and circulating blood of 100 BrCa women. Methylation of SFN in tumors (61 %) correlated significantly with sera (56 %). Significant correlations were also found for methylation of ERα with PRB and SFN [18].
4.4.2.2 Circulating BrCa Prognostic Epigenetic Biomarkers
The methylation of RASSF1A in circulation is a potential predictive biomarker of response to adjuvant tamoxifen systemic therapy. Methylation frequencies in pre- and posttreatment sera were identical (19.6 % pretreatment and 22.3 % posttreatment). The presence of RASSF1A methylation after 1 year of surgery and adjuvant chemotherapy was a predictor of poor outcome with a relative risk (RR) of 5.1 for relapse and 6.9 for death. The loss of serum RASSF1A methylation during treatment was indicative of response [19]. Muller et al. examined 39 genes in an evaluation set using high-throughput MethyLight assay [20]. In applying appropriate selection strategies, five genes (ESR1, APC, HSD17B4, HIC, and RASSF1A) were identified to be of prognostic potential. Methylation of RASSF1A and/or APC was found to be associated with poor outcome in the training set and was confirmed in a validation cohort. Multivariate analysis of all pretreatment sera confirmed RASSF1A and APC methylation to be an independent predictor of poor outcome with an RR 5.7 for death. In a subsequent study, Muller et al. examined the prognostic value of methylation in several genes in pretreatment sera from patients with breast (n = 112) and cervical (n = 93) cancers [21]. In cervical cancer, hypermethylation of MYOD1, CDH1, and CDH13 were significantly associated with poor outcomes. This report further confirmed the importance of serum hypermethylation of RASSF1A and APC as independent prognostic biomarkers for BrCa.
DNA from tumor, normal breast tissue, normal blood cells, and plasma were used for genetic analysis targeting MSA using the following markers, D17S855, D17S654, D16S421, TH2, D10S197 and D9S161, as well as TP53 mutations and methylation of CDKN2A. At least one of these alterations occurred in tumors from 90 % of patients and in 66 % of plasma samples. The presence of cancer genome in circulation evidenced by these molecular alterations correlated significantly with poor prognosis [22]. In another study, 44 % of patients harbored tumor DNA alterations (either MSA, TP53 mutation, or CDKN2A methylation). These alterations were detected in plasma before mastectomy, and 19.5 % retained plasma gene alterations 4–6 weeks after mastectomy, and this was significantly associated with patients who presented with >3 lymph node involvement, vascular invasion, and higher histologic grade at diagnosis. These findings have potential for prognostication at initial diagnosis [23]. Hypermethylation of CDKN2A and CDH1 were examined in tumor and plasma samples and correlated with two serum markers, CEA and CA 15–3 in women with invasive BrCa. CDKN2A was methylated in 11 % of tumors and 8 % of plasma samples, while CDH1 methylation was in 25 % of tumors and 20 % of plasma samples. Either gene was methylated in 31 % of tumors, but in as many as 82 % of plasma samples from those with gene methylation. Methylation of CDKN2A in tumor was associated with advanced stage, size, and nodal metastasis; however, plasma CDKN2A methylation only correlated with nodal metastasis. Combined CDKN2A methylation and serum CEA levels were significantly associated with tumor stage, size, and extensive nodal involvement [24]. The promoter methylation of DNA repair genes (BRAC1, MGMT, and GSTP1) in tumor and circulating DNA of 100 BrCa patients were examined. These genes were methylated at various frequencies from 27 to 32 % in both tissue and sera and were associated with loss of protein expression (except for BRAC1). GSTP1 and BRAC1 hypermethylation were independent predictive factors for disease recurrence [25].
4.4.3 Circulating BrCa Genetic Biomarkers
4.4.3.1 Circulating Chromosomal Alterations as BrCa Biomarkers
BrCa is a disease of chromosomal instability including LOH, aneuploidy, as well as epigenetic alterations and mutations of specific genes. Together, these lesions interfere with normal functioning of signaling pathways important in cellular maintenance such as apoptosis, DNA repair, senescence, cell cycle control, cellular proliferation, and differentiation among others. Several chromosomal regions are amplified, deleted, or rearranged in BrCa. Affected chromosomes include 1, 3, 4, 6, 7, 8, 9, 10, 11, 12, 13, 15, 16, 17, 18, 19, 20, and 22. That these instabilities are causative and/or drivers of BrCa progression is the fact that benign breast pathologies such as fibroadenoma rarely harbor these genetic alterations, with premalignant cells containing intermediate proportions of chromosomal instabilities, but levels become markedly increased in aneuploid BrCas. Mutations and other DNA sequence anomalies are found in BRAC, TP53, EGFR, VEGF, and HER2 and multiple other genes in BrCa. These genetic alterations have been profiled in body fluids as BrCa biomarkers.
4.4.3.2 Circulating Mutated Genes as BrCa Biomarkers
Mutations in the guardian of the genome, TP53 have been detected in ~37 % of primary BrCa tissues. Of these positive samples, 46 % had detectable mutations in ccfDNA in plasma. The mutations correlated with clinical stage, tumor size, estrogen receptor status, and lymph node metastasis. A 29-month follow-up revealed in both univariate and multivariate analyses that TP53 mutations (in both plasma and tissue) significantly predicted recurrence-free and overall survival. In both lymph node-positive and lymph node-negative patients, mutation status still conferred a worse survival outcome [26]. Mutations in TP53 and CDKN2A as well as six microsatellite markers were assayed in tissues, plasma, and blood cells in women with BrCa before and after mastectomy. Forty-four percent of the genetic alterations in tissue samples were detectable in plasma prior to mastectomy. Plasma served as a useful noninvasive medium to monitor women who after surgery still harbored micrometastatic cancer cells [23]. An ultrasensitive method referred to as MIDI-Activated Pyrophosphorolysis (MAP) was designed to detect microinsertions, deletions, and indels (MIDI) that make up 15 % of all genomic mutations. Using this method, plasma samples could be assayed to determine responders and nonresponders to therapy. Posttreatment remission was associated with undetectable TP53 mutations as opposed to nonresponders who had persistent circulating mutant TP53 [27]. PIK3CA mutations were examined in ccfDNA using BEAMing technology. The detection rate was about 29 % in two independent cohorts, and this assay achieved a 100 % concordance between plasma and tissue samples. Cancer recurrence altered PIK3CA status, thus requiring the reassessment of patients using body fluid samples such as blood [28].
4.4.3.3 Circulating MSA as BrCa Biomarkers
Microsatellite alterations (MSAs) in BrCa tissue samples correlate with circulating levels and are detectable in both early and advanced stage disease. Seven polymorphic markers were used for LOH analysis in primary and metastatic BrCa samples, as well as matched blood and bone marrow plasma. Marker D3S1255 showed concordant frequencies of LOH in tissue, blood, and bone marrow plasma and also was the marker with the highest frequency of LOH in serum and bone marrow plasma. Tumor tissue samples were more frequently altered at D13S2118 and D17S855. A significant relationship between lymph node-positive status and LOH in serum samples was observed for marker D3S1255, and this marker in tissues correlated with undifferentiated nuclear grade [29]. MSI and LOH (used multiple markers including markers DM1 and D17S1325) in plasma or sera from BrCa patients indicated LOH was present in 15–48 % of plasma samples [30]. The LOH was importantly detectable in patients with early stage disease, such as those with small tumors (T1), low grade I, and in situ carcinomas, indicating their potential as noninvasive early detection biomarkers. Two polymorphic markers, DM1 and D16S400, were used to assess LOH and MSI in plasma, tissue, and lymphocyte DNA from primary and metastatic BrCa patients [31]. Plasma LOH (31.3 %) and MSI (11.6 %) were frequent events in BrCa patients, and these changes were concordant with tissue MSI. MSA was detectable in women with primary and metastatic BrCa. Plasma DNA was found preferable to bone marrow or other invasive sampling methods to assess tumor burden in sequential sampling for BrCa management.
4.4.4 Circulating BrCa Coding RNA Biomarkers
Transcripts of telomerase, hTR and hTERT, the two components of telomerase, were assayed in sera from patients with BrCa, benign breast disease, and in healthy volunteers. Transcripts of hTR were present in 94 % of tissue samples but in only 28 % of sera, while hTERT was positive in tissue samples at the same frequency of 94 % and in sera of 25 % of patients. However, they were undetectable in sera from patients with benign breast disease and healthy controls [32]. Novakovic et al. also addressed the possible presence of hTR and hTERT transcripts in plasma from women with primary BrCa and patients with advanced melanoma and advanced thyroid cancer [33]. Among the BrCa cohort, hTR transcripts were present in all tested plasma, but hTERT was present in 52.2 % of the samples. In malignant melanoma patient samples, hTR and hTERT were detected at frequencies of 100 % and 71 %, respectively.
Transcripts from other genes have been assayed in circulation of BrCa patients as potential prognostic biomarkers. Yie et al. tested BIRC5 expression in BrCa cells in circulation [34]. Of 67 patients tested, 34 (50.7 %) expressed the gene compared to none of the 135 controls. BIRC5 expression was associated with blood vessel invasion; histologic grade; tumor size; nodal status; ER, PR, and HER2 status; and clinical stage. In a 26-month follow-up period, 81.8 % of patients with positive BIRC5 expression relapsed compared to 33.3 % of patients without initial BIRC5 expression. Polycomb member, BMI-1, controls cellular proliferation, and its deregulation is associated with cancer. Because deregulated expression is demonstrated in many tumor tissues, analysis in plasma was conducted. The expression of BMI-1, examined in plasma from 111 BrCa patients and 20 controls, revealed elevated expression in samples from cancer patients. This high expression correlated with poor prognostic factors such as TP53 expression and PR-negative status. In patients with advanced stage disease, plasma BMI-1 transcript levels also correlated significantly with poor disease-free and OS [35]. This group later examined the predictive value of plasma CCND1 and TYMS (thymidylate synthase) mRNA in BrCa women. Among patients clinically classified as having good prognosis, CCND1 expression in plasma conferred poor outcome. The presence of both markers in plasma was associated with dismal response to therapy upon relapse. Additionally, CCND1 expression predicted patients nonresponsive to tamoxifen [36]. Serum metastasis mRNA as a predictive biomarker was investigated. As a screening tool, this assay achieved a sensitivity of 85 % and specificity of 100 % for BrCa detection. Prognostically, higher serum levels were associated with lymph node involvement and poor survival (six times worse outcome compared to those with low levels). Serum metastatin-positive patients had distant metastasis, while patients who were negative had only local recurrences [37].
4.4.5 Circulating BrCa Noncoding RNA Biomarkers
Breast cancer is characterized by deregulated expression of hundreds of miRNAs that participate in disease progression. Among these are upregulated miR-10b, miR-21, and miR-27a, as well as downregulated let-7, miR-125a, miR-125b, and miR-206. Indeed, miRNA-deregulated expression characterizes various neoplastic cells of the breast. For example, miR-15b, miR-21, miR-30d, miR-141, miR-183, miR-200b, and miR-200c are upregulated, while miR-572, miR-638, miR-671-5p, and miR-1275 are downregulated in atypical ductal hyperplasia. Some of these miRNAs including miR-21, miR-141, miR-183, miR-200b, miR-200c, miR-638, and miR-671-5p show similar deregulated expression patterns in DCIS/IDC. Moreover, some miRNAs characterize molecular BrCa subtypes. For instance, miR-214 is elevated in normal-like, luminal A, and triple-negative BrCa subtypes. Noteworthy, aberrant expression of miRNAs is involved in BrCa stem cell proliferation, self-renewal, differentiation, and metastasis. Among these BrCa stem cell miRNAs are upregulated miR-27 (induced by VEGF), miR-142, miR-214, and miR-221/222 clusters and decreased expression of let-7 family, miR-183 and miR-200 clusters. Several of these as well as novel miRNAs show differential levels in the peripheral circulation of BrCa patients, with diagnostic and prognostic relevance (Table 4.2).
Table 4.2
Circulating BrCa miRNAs
Diagnostic | Prognostic | |
---|---|---|
Increased | Decreased | Increased |
miR-10b, miR-16, miR-21, miR-25, miR-29a, miR-34a, miR-125b, miR-141, miR-145, miR-148b, miR-155, miR-191, miR-200a, 200b, miR-200c, miR-203, miR-210, miR-215, miR-222, miR-299-5p, miR-324-3p, miR-373, miR-375, miR-376c, miR-382, 409-3p, miR-411, miR-451, miR-801 | miR-30a, miR-92a, miR-145, miR-768-3p | let-7a, miR-10b, miR-34a, miR-141, miR-155, miR-195, miR-200a, miR-200b, miR-200c, miR-203, miR-210, miR-375, miR-801 |
4.4.5.1 Circulating BrCa Diagnostic miRNA Biomarkers
Zhu and colleagues were first to publish altered serum miRNA levels in BrCa patients [38]. Levels of miR-16, miR-145, and miR-155 conferred risk for BrCa, and miR-155 correlated with PR status. Circulating miR-148b, miR-376c, miR409-3p, and miR-801 are uncovered as early detection BrCa biomarkers. Indeed, except for miR-801, the rest could differentiate BrCa from benign tissues [39]. Low plasma levels of miR-145 and increased miR-451 discriminate BrCa from controls [40]. Serum miR-145 is rather elevated in some cancer patients [41]. Other potential early detection circulating BrCa miRNAs are miR-21, miR-92a, miR-10b, miR-125b, miR-155, miR-191, miR-382, and miR-30a [41–43]. Circulating miR-195 was diagnostic at 87.7 % sensitivity and 91 % specificity. MiR-195 was also detected in blood from early stage cancer (tumors < 2 cm) patients, and levels correlated with tumor size. The levels of miR-195 returned to normal after surgery [44]. A panel of miR-16, miR-25, miR-222, and miR-324-3p was highly accurate (sensitivity of 92.1 % and specificity of 93.4 %) in detecting BrCa. Serum miR-29a and miR-21 are elevated in BrCa patients as well, and serum miR-155 is a potential diagnostic biomarker of BrCa.
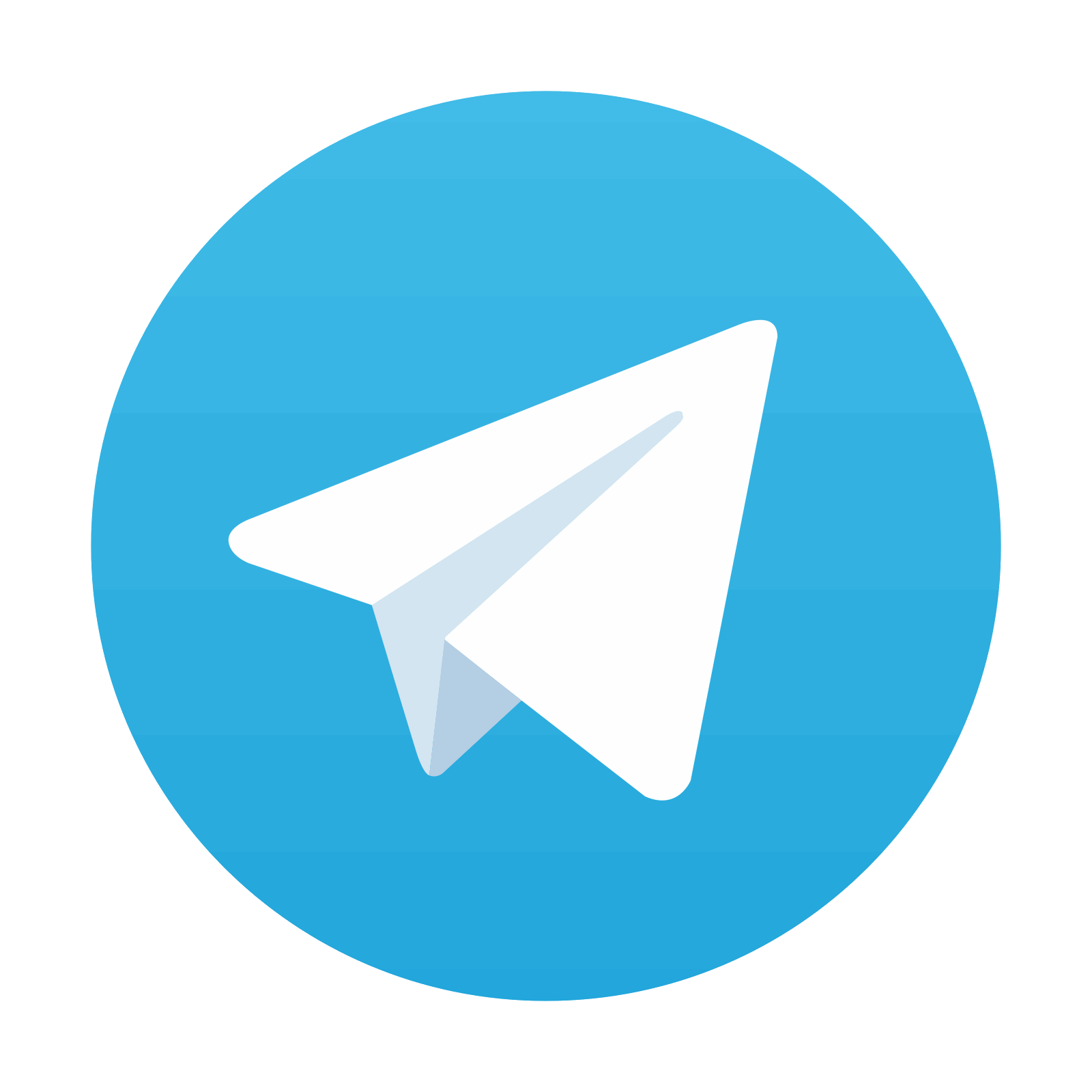
Stay updated, free articles. Join our Telegram channel

Full access? Get Clinical Tree
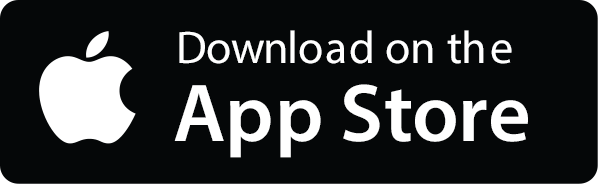
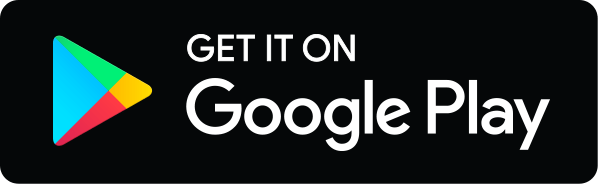
