Biology of the B Lymphocyte
Introduction
In previous chapters we described how B cells synthesize an enormous array of antibodies with different functions, with the function of the antibody—IgM, IgG, IgA or IgE—depending on the nature of the heavy chain. We also discussed the mechanisms B cells use to develop a vast repertoire that can respond to the universe of different antigenic specificities. These mechanisms explain two key features of the adaptive immune response (Chapter 1, Figure 1.1): diversity, the ability to respond to an enormous variety of different antigenic determinants (epitopes) even if the individual has not previously encountered them; and specificity, in which only the lymphocyte expressing the “correct” receptor among the universe of lymphocytes interacts with a particular epitope (clonal selection theory). With this enormous repertoire of potentially reactive B cells, it can almost be guaranteed that every person contains one or more clones of B cells able to interact with measles virus, one or more clones of B cells able to interact with a mycobacterium, and so on.
In this chapter we focus on the critical steps in B-cell development, from the earliest stages that take place in the absence of antigen through to the later stages that occur after the interaction with antigen and result in antibody synthesis. By these processes B cells acquire two other key features of the adaptive immune response: discrimination between self and nonself—the ability to respond to antigens that are “foreign” or nonself, and not to respond to antigens that are self; and memory—the ability to recall previous contact with a particular antigen so that a subsequent interaction leads to a quicker, more effective secondary immune response.
Development of B Lymphocytes
Overview
B lymphocytes acquired their name from early experiments in chickens. The synthesis of antibody was shown to require the presence of an organ called the bursa of Fabricius (an outpouching of the cloacal epithelium). Surgical removal of the bursa early in life prevented antibody synthesis. Thus the cells that developed into mature, antibody-forming cells were called bursa-derived or B cells. In contrast to chickens and other birds, mammals do not have a bursa; rather, the early stages of mammalian B-cell differentiation take place predominantly in the bone marrow throughout the life of the individual. Our understanding of B-cell differentiation has been facilitated by studies in animals in which the early embryonic stages can be easily manipulated. For this reason, B-cell differentiation is particularly well characterized in mice and chickens, but many of these differentiation steps are common to humans as well.
Figure 8.1 illustrates the key stages in the B-cell differentiation pathway, many of which are defined by specific immunoglobulin (Ig) gene rearrangements and by the expression of different surface markers (CD markers). As we describe below, many of the stages also represent developmental checkpoints—the signal received at these checkpoints determines whether the cell develops along one or another alternative pathway. The lack of a signal at this point may result in the death of the cell. Similar developmental checkpoints are also found in the differentiation of the T-cell lineage (see Chapter 10).
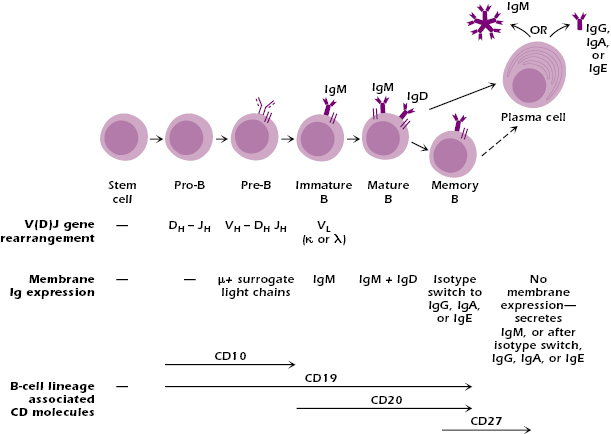
Sites of Early B-Cell Differentiation
B lymphocytes arise from hematopoietic stem cells. In mammals, the hematopoietic stem cells and the precursors of the B-cell lineage are found early in development of the fetus, at sites including the fetal liver. Later in fetal development, after birth and for the rest of life, the bone marrow is the main site of the early stages of B-cell differentiation. Thus in humans and other mammals, the bone marrow is the primary lymphoid organ for B-cell differentiation—the site at which the antigen-specific receptor is first expressed (see Chapter 3). B-cell differentiation occurs more or less throughout life, so the B-cell repertoire is continuously replenished.
During the early steps in the bone marrow, the stroma, the nonlymphoid cells that make up the framework or matrix of the marrow, provide critical adhesive interactions and produce cytokines such as interleukin (IL)-7 that promote the survival and enhance the proliferation of cells early in the B-cell lineage.
Pro-B and Pre-B Cells: First Ig Rearrangements
Pro-B cell: D to J Rearrangement.
Figure 8.1 depicts the earliest distinguishable cell in the B lineage, the pro-B cell, which shows the first rearrangement of Ig genes: at the heavy-chain locus, a DH gene segment rearranges to a JH gene segment. This rearrangement is mediated by the V(D)J recombinase (also see Chapter 7). Pro-B cells express CD19, and CD19 is expressed on the surface of all subsequent stages of B-cell development except the plasma cell. CD19 is expressed almost exclusively on B cells, so expression of CD19 is a useful marker of all cells in the B-cell lineage up to the plasma cell. Pro-B cells also express CD10.
Pre-B Cell: V-DJ Rearrangement.
In the next stage of B-cell differentiation, the pre-B cell, the Ig heavy-chain gene locus undergoes a second rearrangement. The V(D)J recombinase joins a heavy-chain VH gene segment to the rearranged DHJH segments, forming a VDJ unit. This rearranged VDJ is thus positioned close to Cμ, and the pre-B cell synthesizes a μ chain. No light-chain gene rearrangement has yet taken place. Like pro-B cells, pre-B cells express CD10 in addition to CD19.
The Ig heavy-chain gene rearrangements that occur during these early phases of B-cell differentiation follow an ordered sequence (Chapter 7): If the rearrangement at the heavy-chain locus on the first of the two parental chromosomes is productive, that is, capable of being properly transcribed and translated into a μ chain, further rearrangement of heavy-chain gene segments is shut down. If the rearrangement is not productive on the first chromosome, rearrangement takes place on the second chromosome. If this rearrangement is productive, a μ chain is made.
The Pre-B Cell Receptor.
A key characteristic of the pre-B cell is that it expresses the pre-B cell receptor (pre-BCR) at the surface (Figure 8.2A). The expression of the pre-BCR is a key early developmental checkpoint in the B cell lineage; if the cell does not make a pre-BCR—because no heavy-chain rearrangement is productive—the cell dies by apoptosis, also known as programmed cell death.
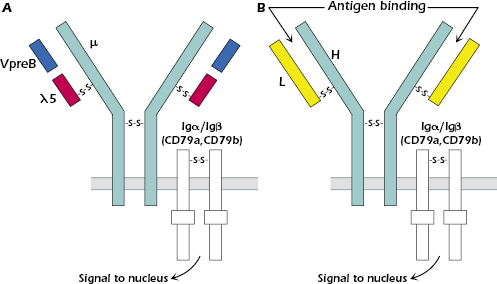
The pre-BCR consists of the μ chain and two sets of tightly associated proteins: One set are the surrogate light chains VpreB (CD179a) and λ5 (CD179b) (Figure 8.2A) that bind to the μ chain on the outer side of the cell surface. VpreB is structurally similar to an IgV region and λ5 is structurally similar to the constant region of a λ light chain. λ5 and VpreB are the products of non-rearranging genes that are expressed only at this stage in B-cell development. λ5 and VpreB are associated but not covalently linked to each other; λ5, though, is covalently linked through disulfide bonds to the μ chain, similar to the binding of a light chain to a heavy chain.
The second set of proteins associated with the μ chain are Igα (CD79a) and Igβ (CD79b), which are juxtaposed in the membrane with the Ig heavy-chain on all cells in the B-cell lineage, from the pre-B cell to the memory B cell (see Figure 8.1). Igαand Igβ form a disulfide bond-linked heterodimer (Igα/Igβ). Igα/Igβ do not bind antigen but act as signal transduction molecules for both the pre-BCR (Figure 8.2A) and the B-cell receptor (BCR) (Figure 8.2B), the complex of molecules that is expressed in cells of the B-cell lineage beyond the pre-B cell stage of development. Signal transduction molecules transmit signals into the cell that activate intracellular pathways which ultimately reach the cell nucleus, leading to a change in the pattern of genes expressed. Similar signal transduction molecules are associated with the antigen-specific receptor on the T lymphocyte (Chapters 10 and 11). The signal transduction function of Igα/Igβ is mediated by their cytoplasmic regions, which contain sequences of amino acids known as immunoreceptor tyrosine-based activation motifs (ITAMs). The function of ITAMs is explained later in this chapter.
In B cells that express a BCR, Igα/Igβ transmit signals following antigen binding. It is currently unresolved, however, what molecules, if any, act as ligands for the pre-BCR to trigger signaling. Our current understanding is that in the pre-B cell, the signal from Igα/Igβ indicates that the cell has successfully rearranged its Ig heavy-chain genes and has made a functional μ chain. As a result of signaling through the pre-BCR, the pre-B cell further differentiates: It proliferates, shuts down surrogate light-chain synthesis, starts light-chain gene rearrangement mediated by the V(D)J recombinase, and stops further heavy-chain gene rearrangement.
Like heavy-chain gene rearrangements, light-chain gene rearrangement in the later phases of pre-B-cell development is sequential: the κ-chain genes rearrange first, but if neither of the chromosomes coding for κ chains rearranges successfully, λ gene rearrangement takes place. If no productive light-chain gene rearrangement occurs, the cell dies. The biological consequence of this use of genes from only one chromosome to make a heavy chain and genes from one chromosome to make a light chain—allelic exclusion—ensures that an individual B cell expresses on its cell surface an Ig molecule with only one single antigenic specificity (also see Chapter 7).
Bruton’s tyrosine kinase (Btk) is an enzyme involved in a step in intracellular signaling from the pre-BCR (and BCR) that eventually leads to the nucleus. Btk plays a crucial role in the transition of pre-B cells to the next stage in B-cell differentiation: Boys with mutations in the BTK gene develop the immunodeficiency condition, X-linked agammaglobulinemia, in which B-cell differentiation is arrested at the pre-B-cell stage because of a lack of signaling (discussed in Chapter 18).
Immature B Cells
At the next stage of B-cell differentiation, the immature B cell, light chains pair with μ chains to form monomeric IgM, which is expressed at the cell surface in association with Igα/Igβ to form the BCR (Figure 8.1). Immature B cells express CD20, which is also expressed by cells in the next stages of B-cell differentiation; thus, CD20 is a marker for the later stages of B-cell development.
Negative Selection and the Development of Central Tolerance.
The immature B cell can respond to antigen; that is, the IgM expressed on the immature B cell can bind and respond to an individual antigenic epitope. Because the mechanisms used to generate this IgM—VDJ recombination and the pairing of heavy and light chains—are essentially random, some immature B cells that develop will express an IgM specific for a foreign (nonself) antigen and some will express an IgM specific for a self-antigen. If the immature B cell expresses a receptor for a nonself-antigen, it leaves the bone marrow and develops further to become a member of the huge repertoire of mature B cells specific for nonself-antigens.
However, allowing a B cell that has too high a reactivity to a self-molecule to leave the bone marrow runs the risk of developing autoimmune problems in the tissues. Thus, if an immature B cell expresses a receptor specific for a self-antigen and interacts with that self-antigen in the bone marrow, it is eliminated or deleted, by apoptosis (Figure 8.3). The self-antigens that are recognized by immature B cells are likely molecules expressed by stromal cells in the bone marrow.
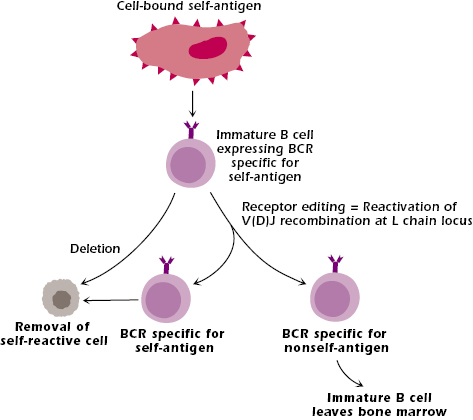
This elimination or deletion of self-reactive B lymphocytes during development in the bone marrow is known as negative selection. It is a fundamental feature of the clonal selection theory and the development of central tolerance: the development of tolerance to self in the primary lymphoid organ, the organ or tissue in which lymphocytes acquire their antigen-specific receptor. As a consequence of negative selection, the lymphocytes that survive and leave the primary lymphoid organ to move into the periphery are tolerant to self but reactive to foreign antigens. Negative selection is also a critical feature of T-cell development in the thymus. Negative selection and central tolerance are discussed further in Chapters 10 and 13.
However, not all self-reactive immature B cells are deleted as soon as they react with self-antigen (Figure 8.3). Some self-reactive cells have the opportunity to change their receptor, via receptor editing. In this pathway, the interaction of self-antigen with the BCR expressed on the immature B cell reactivates the cell’s V(D)J recombinase, and Ig light-chain genes undergo a secondary rearrangement that uses unrearranged V and J light-chain segments. For example, in the rearrangement of the B cell’s κ locus shown in Figure 7.3 in Chapter 7, V1 and J5 are unrearranged gene segments that may be recombined during receptor editing. Receptor editing does not occur on the heavy chain because the initial rearrangement deletes DH segments and the VH and JH segments that remain cannot be combined without a DH.
If receptor editing generates an Ig specific for a nonself-antigen, the immature B cell survives and is able to leave the bone marrow; it becomes part of the repertoire of B cells responsive to nonself-antigens. If, however, receptor editing generates a specificity for the same or a different self-molecule, the immature B cell is deleted via apoptosis. In this way, B cells with the potential for high reactivity to self-molecules are prevented from exiting the bone marrow and entering the bloodstream and tissues. In some mouse models, self-reactive immature B cells that interact with self-antigen are not always deleted. They survive but are not able to respond to antigen; these B cells are said to be anergic; this is described further in Chapter 13.
Transitional B cells
Immature B cells that survive negative selection in the bone marrow have gone through a second critical developmental checkpoint. They remain in the bone marrow for approximately 24 hours, and then move out through blood to the spleen. These cells are known as transitional B cells. At this stage they start to express IgD together with IgM at the cell surface.
Transitional B cells are the first stage in the B-cell differentiation pathway to express a receptor for, and so respond to, the cytokine BAFF (B lymphocyte factor belonging to the tumor necrosis factor family, also known as B lymphocyte stimulator [BLyS]). BAFF, which is synthesized by multiple cell types including monocytes, dendritic cells and bone marrow stromal cells, is a survival signal for transitional B cells, as well many later stages in the B cell lineage. High BAFF levels have been reported in patients with the autoimmune conditions rheumatoid arthritis and systemic lupus erythematosus, and one current therapy for those diseases is targeted at reducing BAFF levels (see Chapter 13).
Mature B Cells
The next stage in the B-cell differentiation pathway is the IgM+ IgD+ mature B cell, which expresses higher levels of IgD than does the transitional B cell. These mature B cells that have not yet been exposed to antigen are referred to as naïve, and their interaction with antigen in the secondary lymphoid tissues generally results in activation, leading to the formation of memory cells or plasma cells and the production of antibody.
The IgM and IgD expressed on a single B cell have identical antigenic specificity as a result of having the same V region in the heavy chains and using the same light chain (see Figure 7.4). The function of IgD on the mature B cell is not well understood and is downregulated following antigen exposure. In addition to IgM and IgD, the mature B cell expresses several other molecules on its surface, which play key roles in interactions with other cells, particularly T cells, or that have other essential roles in transmitting signals into the B cell nucleus (see below).
Most naïve mature B cells circulate through the blood to secondary lymphoid organs, primarily to lymph nodes throughout the body and to mucosa-associated lymphoid tissue (MALT). These B cells are referred to as follicular B cells because they are found in primary and secondary follicles (see Chapter 3). The circulation time of about 12 hours quickly brings a B cell with the “correct” antigenic specificity in contact with antigen if it is present. Some mature B cells move to the marginal zone of the spleen, where they develop into splenic marginal zone B cells, which do not recirculate through the blood.
The recombination mechanisms operating during differentiation and throughout the life of the individual generate a vast repertoire of B-cell antigen specificities, more or less guaranteeing a response to any antigen that a person may encounter. The repertoire is so extensive that most mature B cells do not interact with antigen during their lifetime but remain as resting unstimulated IgM+ IgD+ B cells.
Plasma Cells
Plasma cells (Figure 8.1) synthesize and secrete Ig molecules and are the terminally differentiated stage of B-cell development. They do not express a membrane form of Ig. An individual plasma cell secretes antibody of a single antigenic specificity—the same antigenic specificity as the immunoglobulin on the surface of the B cell that developed into that plasma cells and that was initially triggered by antigen—and of a single isotype: IgM, IgG, IgA, or IgE. Plasma cells (and memory cells) express high levels of CD27, which is not expressed on other cells in the B cell lineage, but they do not express the markers CD10, CD19, or CD20 that characterize earlier stages of the B-cell lineage.
In responses to the major set of antigens known as thymus-dependent (TD)—because they require helper T cells (see below and Chapter 11)—plasma cells are generated at two major sites: in the germinal center of lymph nodes and spleen, and in MALT. Responses to TD antigens generally involve synthesis of antibodies of more than one isotype (that is, IgM plus IgG, IgA, or IgE) and antibodies specific for more than one epitope of a particular antigen. Thus, the population of plasma cells responding to an antigenic challenge is diverse and produces a mixture of immunoglobulins (a polyclonal response).
Both short-lived (days to weeks) and long-lived (up to years) plasma cell populations develop in the germinal centers of lymph nodes outside MALT synthesize and secrete IgG, IgA, or IgE. Long-lived plasma cells migrate primarily to the bone marrow, where they synthesize high levels of IgG and monomeric IgA that provide protection against a subsequent exposure to infectious agents such as viruses and bacteria. Long-lived IgE-synthesizing plasma cells are also found in the bone marrow. Plasma cells that develop in MALT synthesize and secrete the dimeric IgA at mucosal sites such as the gastrointestinal and respiratory tracts (see Figure 5.13), as well as salivary and tear glands and lactating mammary glands.
Some antigens do not require T-cell help for the B cell to make antibody. These are referred to as thymus-independent (TI) antigens. The polysaccharide components of bacterial capsules are one clinically important set of TI antigens. Responses to TI antigens are generally rapid (within a few days after exposure to antigen) and almost exclusively involve synthesis of IgM antibodies. The IgM synthesized in response to TI antigens is made by short-lived plasma cells in the marginal zone of the spleen. This IgM can agglutinate the antigen and activate the complement system, providing crucial early protection against many bacterial infections, even in people who lack T cells (discussed further in Chapter 18). Sites of IgM production are also discussed later in this chapter.
In Chapter 6 we described how plasma cells can be transformed and “immortalized” in cell culture. They can be used to generate monoclonal antibodies—antibodies specific for a single antigen epitope—that have a wide range of clinical and diagnostic functions.
Memory B Cells
Memory B cells develop in germinal centers of secondary organs after TD-antigen activation of the mature B cell. They express isotypes other than IgM (IgG, IgA, or IgE) on their surface and do not express IgD. Memory cells express CD27, as do plasma cells. Memory B cells are nonproliferating, generally long-lived cells, that can be activated for a subsequent (secondary) and more rapid response to antigen. When reactivated, memory cells can be converted into plasma cells. Memory B cells leave the lymphoid organ in which they were generated. Rather than circulating through lymphoid organs as naive B cells do, memory B cells move into tissues.
Sites of Antibody Synthesis
The major function of B cells—specifically plasma cells—is to synthesize Ig molecules. In the sections that follow we describe in more detail the main sites in the body where Ig synthesis occurs, and the different types of responses to antigen that occur at those sites.
Interaction of Antigen, B Cells, and Helper T Cells in the Lymph Node
We described above that antibody responses to TD antigens require helper T cells. These helper T cells express the surface marker CD4 and so are referred to as CD4+ T cells (see Chapters 10 and 11). The CD4+ T and B cell that cooperate in the response to TD antigens are both specific for the same antigen; we say these are cognate
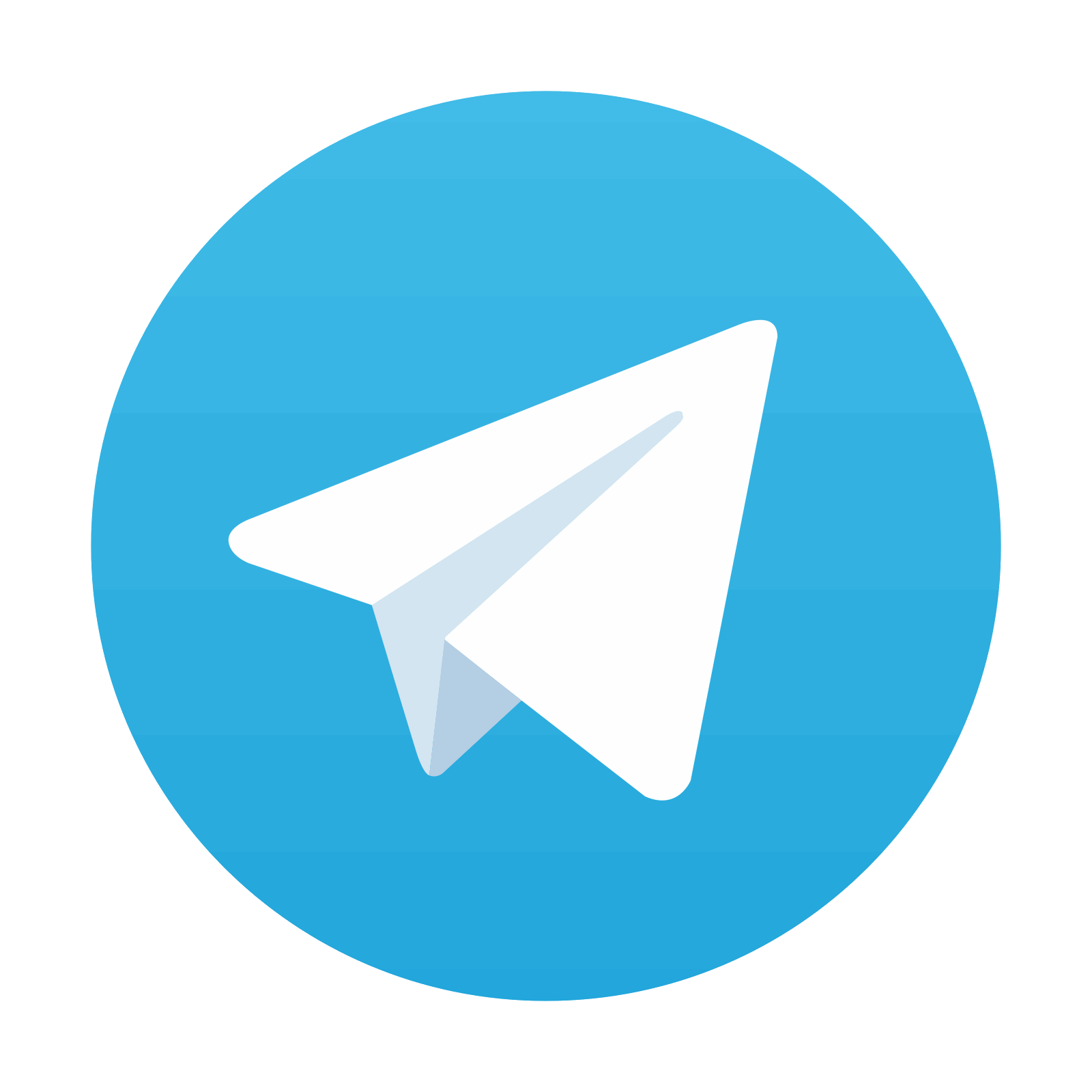
Stay updated, free articles. Join our Telegram channel

Full access? Get Clinical Tree
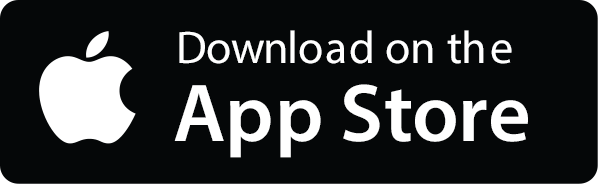
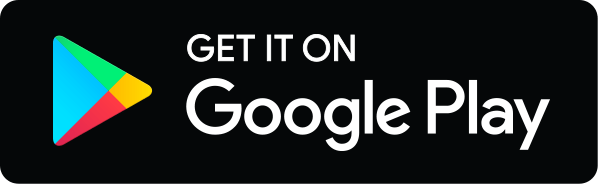