Activation and Function of T Cells
Introduction
In previous chapters we touched on the signals that are needed to activate the two major subsets of T cells CD4+ and CD8+ T cells. In this chapter we describe in more detail the cells and signals involved in T-cell activation, and how once T cells are activated they exert their effector functions. For CD4+ T cells, we will focus on the cytokines produced by different subsets and their role as helper T cells in antibody synthesis by B cells. For CD8+ T cells we will describe their role as cytotoxic or killer T cells. We will also describe how memory cells are formed and the termination of the T cell response. We end with descriptions of the roles of other subsets of T cells in the immune response.
A Two-Signal Model for the Activation of T Cells
Figure 11.1 shows that two sets of signals are needed to activate naïve CD4+ and CD8+ T cells, that is, T cells that have not previously encountered antigen. The first signal is antigen-specific: peptide bound to major histocompatibility complex (MHC) on the surface of a host cell interacting with the T-cell receptor (TCR). The interaction of the T-cell co-receptor (CD4 or CD8) with the MHC molecule is considered part of this first signal. The second signal is not antigen-specific and is provided by co-stimulator pairs, molecules expressed on the surface of specialized antigen-presenting cells (APCs) interacting with molecules expressed on the T-cell surface. Both the first and second signals send messages into the T-cell nucleus (i.e., trigger signal transduction) that change the pattern of the genes expressed by that T cell. Both signals are needed to activate naïve T cells; ultimately, they lead to survival, proliferation, and differentiation of the T cell. Note that the first signal by itself is necessary but not sufficient. Indeed, in the absence of a second signal, the first signal alone turns the T cell off rather than on (see Chapter 13).
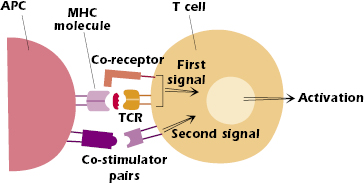
However, we also now recognize that the activation and signaling processes within T cells are complex, and that the family of co-stimulator molecules is extensive. We now understand that some members of the co-stimulator family play important negative and regulatory roles in T cell activation. We discuss these molecules later in the chapter.
In the sections below we focus first on how CD4+ T cells are activated and their function; later in the chapter we discuss the activation and function of CD8+ T cells.
Dendritic Cells Are the Key APC for Naïve T Cells
Only a few specialized, or “professional,” APCs express MHC class II molecules, process protein antigens, and present selected catabolized linear fragments of the protein (peptides) to CD4+ T cells (also see Chapter 9). Dendritic cells are one of these MHC class II expressing cells, and they are the principal APCs for initiating the primary responses of both CD4+ and CD8+ T cells. Dendritic cells, initially named for their dendritic star-shaped appearance under the microscope, are derived from the same hematopoietic precursor as monocytes and macrophages in bone marrow.
Two major subsets of dendritic cells have been distinguished based on their function (and expression of surface molecules). Plasmacytoid dendritic cells synthesize the interferons (IFNs) α and β in the early phases of an immune response and are major contributors to the innate phase of the response to pathogens such as viruses. Myeloid dendritic cells have a major role in the induction of T-cell responses and subsequently we use the term dendritic cells to refer to this set of cells. Dendritic cells are found in the circulation and in many tissues. Antigens can enter the body by several different routes—especially the airways, gastrointestinal tract, and skin—and dendritic cells are found in tissues close to these entry sites. Dendritic cells are also found in lymphoid organs. (In Chapter 10 we discussed the role of the dendritic cells resident in the thymus in negative selection of developing T cells, a key feature of the establishment of central tolerance in the T-cell population.) In addition, dendritic cells are also found in secondary lymphoid organs.
One of the key features of dendritic cells is that they can migrate from tissues to lymph node after encountering antigen. For example, the dendritic cell in the skin—known as the Langerhans cell—interacts with antigen and moves through lymph to the nearest draining node. Thus, dendritic cells can also be divided into “migratory” and “lymphoid organ resident.” Migratory and lymphoid organ resident dendritic cells show some differences in surface molecule expression, but it is not currently clear that these are subsets with distinct functions. In this chapter we focus on the properties of the migratory dendritic cells.
The dendritic cell in tissues that has not interacted with antigen is referred to as an immature dendritic cell (Figure 11.2). It expresses low levels of MHC class II molecules. It also expresses many different pattern recognition receptors (described in Chapter 2) that interact with components of several types of infectious microorganisms, particularly bacteria and viruses. Among these pattern recognition receptors are the Toll-like receptors (TLRs), a family of predominantly cell surface molecules that interact with bacterial DNA, lipoprotein, and lipopolysaccharide, as well as viral RNA and DNA. The immature dendritic cell also expresses phagocytic receptors, which enhance uptake of pathogen into the cell. Once the cell has taken up the pathogen it is also very efficient at catabolizing its protein components into peptides in the MHC class II pathway (Chapter 9).
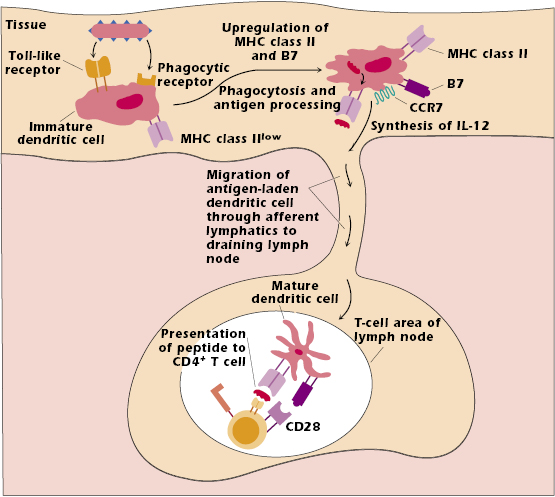
The uptake and processing of a pathogen trigger development into a mature dendritic cell. This cell upregulates and expresses high levels of both MHC class II and co-stimulator molecules, particularly of the B7 family. Upregulation of MHC class II and co-stimulator molecules enhances the ability of the cell to present antigen to T cells. Interaction with bacterial pathogens also results in the synthesis and secretion of many cytokines, one of the most important being IL-12 (see below).
Interaction with pathogen also upregulates dendritic cell expression of the chemokine receptor CCR7, which binds to a ligand expressed by high endothelial venules at the entrance to lymph nodes. As a result, the dendritic cell with peptide bound to MHC class II molecules leaves the tissue in which it encountered pathogen and migrates through lymph vessels to the closest or “draining” lymph node. This mature dendritic cell then presents peptide to a T cell with the “correct” TCR in the T-cell region of the node (Figure 11.2).
The migration of an antigen-bearing dendritic cell to the draining node, combined with the ability of naïve T cells to recirculate through lymph nodes, increases the likelihood that the rare T cell expressing the appropriate TCR will be activated by the antigen-bearing dendritic cell. The interaction between the antigen-bearing dendritic cell and the T cell generally occurs within 8–10 hours of exposure to antigen.
Note that in the absence of signals induced by pathogens, immature dendritic cells express low levels of co-stimulator and MHC class II molecules. Thus, antigens that do not induce high levels of co-stimulator function do not activate naïve T cells. This is why we believe that the encounter of a dendritic cell with self-molecules in normal tissue does not lead to activation of either the dendritic cell or of T cells: co-stimulator function is not induced (see Chapter 9). Similarly, T-cell and antibody responses to many “harmless” foreign antigens (e.g., after injection with peptides or even small proteins in a vaccine) may evoke little or no response. For this reason proteins in many vaccinations are given with an adjuvant to ensure that a response is activated; components of the adjuvant enhance the activation of APCs, in particular by inducing the expression of co-stimulator molecules.
Activation of CD4+ T Cells
The lower part of Figure 11.2 shows the mature antigen-bearing dendritic cell interacting with a naïve CD4+ T cell in the T-cell area of the node. Figure 11.3 and the paragraphs below summarize the key pairs of interactions at the surface of the cells that result in the activation of the CD4+ T cell.
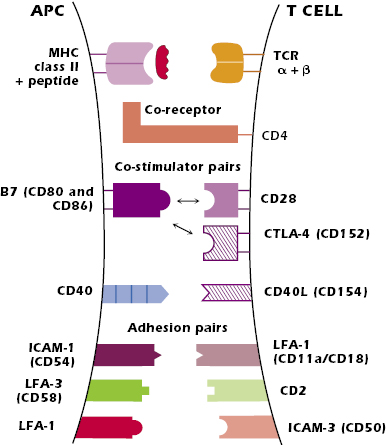
Paired Interactions at the Surface of the APC and CD4+ T Cell
Peptide/MHC and TCR.
The interaction between peptide + MHC class II molecule expressed on the APC and the variable regions, Vα + Vβ, of the T-cell’s TCR is the critical antigen-specific first signal for the activation of the CD4+ T cell. The TCR can detect a very small number of foreign peptides—as low as four peptide molecules out of the thousands of peptides expressed on a particular APC—so the T cell is very sensitive to foreign antigen. However, this interaction is of low affinity and has a rapid off-rate—the interacting components rapidly dissociate. The paired interactions described below both enhance the affinity of the interaction between the TCR and the peptide–MHC and stabilize the interaction between the two cells. Altogether these interactions provide the stability to sustain contact for several hours, a time period needed for activation of naïve CD4+ T cells.
MHC Class II with CD4 Co-Receptor.
The interaction of the nonpolymorphic region of an MHC class II molecule (the region outside the peptide-binding groove) with the co-receptor CD4 greatly enhances the ability of the T cell to respond to antigen, lowering the T-cell antigen stimulation threshold. It has been estimated that the CD4–MHC class II interaction makes a cell 100-fold more responsive to antigen than in the absence of the interaction. In addition, CD4 plays an important role in T-cell signal transduction, which is described below.
Co-Stimulator Pairs.
Co-stimulator pairs at the surface of the dendritic cell (and other APCs) and the CD4+ T cell deliver second signals that enhance the first signal, the MHC–peptide–TCR interaction.
B7–CD28. The best characterized co-stimulator interactions are between a family of molecules known as B7, expressed on professional APCs (dendritic cells, macrophages, and activated B cells) and CD28, expressed constitutively on T cells. The B7–CD28 interaction is a critical positive signal in CD4+ T-cell activation. Within the B7 family, most is known about B7.1 and B7.2 (CD80 and CD86, respectively), both of which bind to CD28. It is currently not clear if B7.1 and B7.2 have distinct functions, and so subsequently we refer to both simply as “B7.”
CD40–CD40L. A further important co-stimulator interaction occurs between CD40 expressed by APCs and CD40 ligand (CD40L or CD154) expressed on activated T cells. The interaction of peptide–MHC with the TCR upregulates T-cell expression of CD40L, which in turn induces increased expression of CD40 on the APC. This CD40–CD40L interaction further increases B7 expression on the APC, enhancing the B7–CD28 interaction between the APC and the T cell. B cells also express CD40 and the CD40–CD40L interaction plays a key role in antibody synthesis (discussed later in this chapter).
Activation of the T cell also induces expression of CTLA-4 (CD152), which is structurally similar to CD28. Both B7.1 and B7.2 bind to CTLA-4 (as well as CD28). The B7–CTLA-4 interaction plays a role later in T-cell activation to turn off the response (described later in this chapter).
Adhesion Molecules.
Pairs of adhesive interactions strengthen and stabilize the interaction of the APC and T cell over the several hours that the cells need to be in contact to ensure T-cell activation. These adhesive interactions slow down the movement apart of the APC and T cell after the cells first interact. This allows more time for the TCR to “scan” the APC for the appropriate MHC class II–peptide.
The key pairs of molecules are the intercellular adhesion molecule (ICAM)-1 (CD54) expressed on the APC and LFA-1 (CD11aCD18) expressed on the T cell; the integrin leukocyte function-associated antigen (LFA)-3 (CD58) expressed on the human APC and CD2 expressed on the T cell; and LFA-1 on the APC with ICAM-3 (CD50) expressed on the T cell. All these adhesion molecules are expressed on multiple cell types.
Intracellular Events in CD4+ T-Cell Activation
The intracellular pathways that are activated after peptide + MHC class II binds to the TCR of the CD4+ T cell are depicted in Figure 11.4. Understanding the intracellular pathways of T-cell activation is important in its own right but also provides insights into how T-cell activation may be blocked and how the function of activated cells may be prevented. These insights are being applied in many clinical situations, including the treatment of autoimmune diseases (see Chapter 13) and the prevention of transplantation rejection (Chapter 19), conditions in which T-cell activation is an undesirable but central feature.
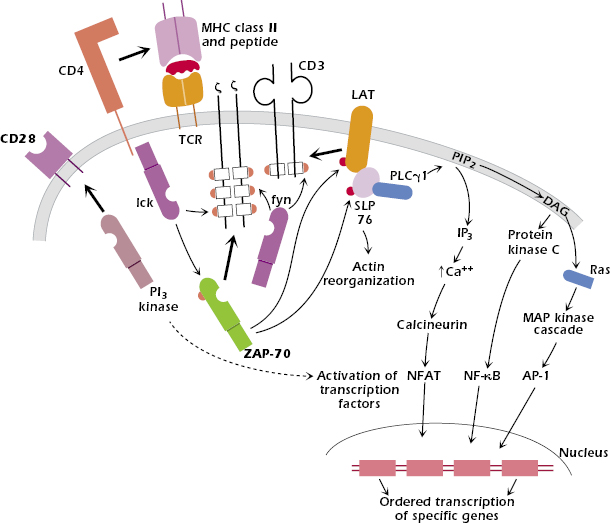
In brief, the recognition of antigen at the cell surface triggers multiple intracellular cascades that transmit signals in an ordered manner from the surface of the cell through the cytoplasm and into the nucleus. As a consequence of activation, the T cell also reorganizes the structure of both its internal cytoskeleton and its cell membrane. Some events occur within seconds, others within minutes, and yet others take hours following the initial interaction. As a result of these events, the T cell changes its pattern of gene expression from a resting or quiescent state to an activated state; the cell proliferates and expands the initial clone size and differentiates into an effector cell able to carry out its specific function.
Below we outline the sequence of events in the activation of the CD4+ T cell. Although we focus on the activation of CD4+ T cells, the pathways activated inside the CD8+ T cell are more or less identical after triggering by peptide–MHC class I complexes. The intracellular events are also very similar to those described in the activation of B cells by antigen (Chapter 8).
Formation of the Immunologic Synapse.
The interaction of an APC and peptide with the CD4+ T cell forms an area of contact between the cells known as the immunologic synapse, by analogy with the area of contact between neurons and other cells. The immunologic synapse appears to be required for sustained intracellular signaling, lasting until the APC and T cell split apart after approximately 8 hours in contact. The synapse is also formed when CD4+ T cells interact with B cells, described later in the chapter. The synapse incorporates the MHC–peptide and TCR, CD4, and pairs of co-stimulator and adhesion molecules. In addition, on the T-cell side, the synapse includes signaling molecules recruited from inside the T cell (described below) and cytoskeleton proteins.
The process of formation and development of the synapse is dynamic, and its composition and structure change with time after the initial contact. For example, the paired adhesion molecules ICAM-1 and LFA-1 are found in different regions of the synapse at different times after initial contact between the cells. In addition, other molecules are included or excluded from the synapse at different times.
The T cell also reorganizes the structure of both its internal cytoskeleton and its cell membrane as a consequence of activation. In the T-cell membrane, the distribution of the lipids is not homogeneous; rather, they form what are referred to as microdomains or lipid rafts, enriched in cholesterol and glycosphingolipids. When the T cell is activated, these lipid rafts, which had been dispersed throughout the membrane, are mobilized to the synapse and draw with them the intracellular signaling components. This redistribution also pushes those molecules not involved in the APC–T-cell interaction out of the contact area.
Initial Signal.
The binding of peptide–MHC to the extracellular variable regions (Vα + Vβ) of the TCR transmits a signal via the tightly associated CD3 and ζ molecules into the interior of the T cell (Figure 11.4). The nature of the signal across the membrane is not currently clear: It may involve the aggregation of multiple TCR molecules in the cell membrane or a conformational change in the transmembrane region of the TCR chains.
Activation of Kinases, Phosphorylation of ITAMs, and Assembly and Activation of Signaling Complexes at the Cell Membrane.
One of the earliest detectable events inside the T cell after binding to the TCR is the activation within seconds of tyrosine kinases, Fyn, which associates with the cytoplasmic regions of CD3 and ζ, and Lck, associated with the cytoplasmic regions of CD4. Fyn and Lck belong to the Src (pronounced “sark”) family of tyrosine kinases. Fyn and Lck are activated by the membrane protein CD45 (not shown in Figure 11.4), a tyrosine phosphatase that removes inhibitory phosphate groups.
When Fyn and Lck are activated, they bind to regions of the CD3 and ζ chains that contain the previously described immunoreceptor tyrosine-based activation motifs (ITAMs) and phosphorylate them (Chapter 10). Because Lck is associated with CD4, this binding also pulls CD4 into close association with the TCR complex. The phosphorylated ITAMs in CD3 and ζ then act as docking sites for another tyrosine kinase, ZAP-70 (belonging to a second tyrosine kinase family known as Syk), that is recruited to the complex. This step appears critical for T-cell activation, because T cells from the rare individuals who lack ZAP-70 do not respond to antigen; these individuals are profoundly immunodeficient (see Chapter 18). Because CD3 and ζ contain multiple ITAMs, more than one molecule of ZAP-70 is recruited into this complex of signaling proteins.
Lck activates ZAP-70 when it has joined the multiprotein signaling protein complex. Activated ZAP-70 phosphorylates linker for activation of T cells (LAT), which binds to the signaling complex already formed. LAT is an adaptor: a protein that does not have enzymatic activity but contains multiple binding domains for other proteins. LAT in turn recruits several more molecules, forming an even larger signaling complex. One of these is another adaptor molecule, SLP76, which activates the reorganization of actin molecules within the cell. SLP76 binds the enzyme phospholipase Cγ1 (PLCγ1). In summary, a multiprotein complex of signal transduction molecules is assembled in sequence and activated on the cytoplasmic side of the T-cell membrane.
Activation of Intracellular Signaling Pathways.
Three major intracellular signaling pathways are activated in the cytoplasm after PLCγ1 is recruited to the signaling complex. Ultimately these pathways lead to the activation of transcription factors that enter the nucleus and alter gene expression.
The first pathway involves increasing intracellular calcium levels. This occurs as a result of the PLCγ1-catalyzed breakdown of the membrane phospholipid, phosphatidylinositol 4,5 bisphosphate (PIP2), which is broken down into two products, one of which is inositol triphosphate (IP3). IP3 triggers the release of intracellular pools of calcium. The increased calcium level in turn activates the cytoplasmic molecule calcineurin, ultimately activating the transcription factor, NFAT. This pathway is clinically significant because the immunosuppressive agents cyclosporin A and tacrolimus (originally known as FK506) bind to and inhibit the function of calcineurin, particularly in T cells, thereby inhibiting the subsequent steps in T-cell activation. That is why these agents are extremely effective in preventing graft rejection when tissues are transplanted between genetically different individuals (see Chapter 19).
A second pathway leads to the activation in the cytoplasm of the transcription factor, NF-κB. This results from the formation of diacylglycerol (DAG), the second product of the PLCγ 1-catalyzed breakdown of PIP2. DAG activates the enzyme protein kinase C (PKC), which in turn activates a cascade of kinases, ultimately activating NF-κB.
The third pathway is the mitogen-activated protein (MAP) kinase signaling pathway, ultimately leading to the activation of the transcription factor AP-1. This pathway is activated when DAG binds to a protein that in turn binds to and activates Ras, an enzyme that hydrolyzes guanosine triphosphate (i.e., it is a GTPase).
The activated transcription factors NF-κB, NFAT, and AP-1 enter the nucleus of the activated T cell and bind selectively to regulatory sequences of several different genes. Two important genes that are transcribed and translated in the activated T cell (within 24 hours of the onset of activation) are the genes for the cytokine IL-2 and for one of the chains of the IL-2 receptor: IL-2Rα (CD25). IL-2 is also known as T-cell growth factor and stimulates T-cell proliferation.
B7–CD28 Interaction.
After B7 and CD28 interact, one of the key early events inside the T cell is the recruitment of phosphatidylinositol-3 (PI3) kinase to the cytoplasmic domain of CD28 (Figure 11.4). In addition, the B7–CD28 interaction mobilizes lipid rafts in the T-cell membrane, which brings tyrosine kinases and other molecules to the area of contact between the TCR and the APC. Ultimately these events lead to the activation and translocation into the nucleus of the transcription factors NF-AT and NF-κB. As described in the section above, these activated transcription factors upregulate expression of IL-2 and IL-2Rα.
The B7–CD28 co-stimulator interaction plays a critical role in IL-2 synthesis by increasing the rate of transcription of the IL-2 gene (via increased production of transcription factors) and increasing the half-life of IL-2 mRNA. The enhancement of IL-2 protein synthesis is calculated to be as much as 100-fold over and above the IL-2 synthesis induced by signaling through the TCR alone. This co-stimulator signal results in both survival—by suppressing apoptosis—and proliferation of the T cell.
Proliferation.
Figure 11.5 shows the events in the activated CD4+ T cell after it starts to transcribe and translate the IL-2Rα and IL-2 genes. Synthesis of the IL-2Rα chain converts the low affinity two-chain IL-2 receptor (β + γ) on the resting T-cell surface into a three-chain (α, β, and γ) high-affinity IL-2 receptor on the activated T cell (see also Chapter 12).
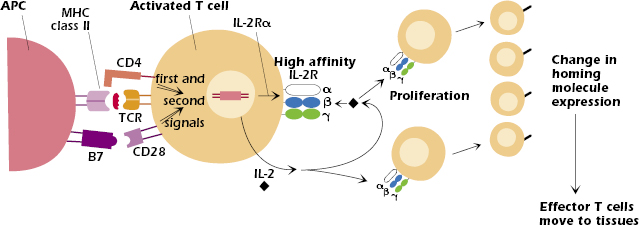
IL-2 is a growth factor for T cells and acts on any cell that expresses the high-affinity IL-2 receptor; thus, as shown in Figure 11.5, IL-2 acts on both the T cell that synthesized and secreted it as well as daughter cells produced by proliferation. As a result, the original clone of T cells undergoes enormous and rapid expansion, increasing the clone size several thousand-fold within a week. This expansion of T (and B cells, which are also activated in the response to antigen) is frequently noted as a swelling of the lymph node in which the response occurs.
Differentiation to Effector Cells and Migration Out of the Lymph Node
Toward the end of this phase of rapid expansion, the activated T cells differentiate into effector T cells, which now have the capability to carry out the effector function of their particular subset of T cells: synthesis of cytokines by CD4+ T cells or killing of targets by CD8+ T cells.
The activation of T cells in the lymph node and differentiation into an effector cell results in a change in expression of homing molecules, the set of adhesion molecules and chemokine receptors expressed by T cells and other leukocytes. Effector cells (and memory cells, described later in the chapter) downregulate the homing molecules that allowed the naïve T cell to enter the node and upregulate molecules that allow them to migrate out of the node and move to different sites in the body, particularly to tissues infected by pathogens (see also Chapter 9 and the discussion of lymphocyte homing in Chapter 8). For example, many effector cells upregulate the integrin VLA-4 (CD49CD29) and the chemokine receptor CCR10, which mediate homing to many tissues (except the skin) and sites of inflammation. Homing to skin is mediated by upregulation of CCR10 and the adhesion molecule, cutaneous lymphocyte antigen (CLA). Like that of activated B cells, migration of effector T cells to mucosal nodes is mediated by the integrin α4β7 and the chemokine receptor CCR9.
Termination of the Response
The interaction of antigen with naïve CD4+ (or CD8+) T cells results in the activation and enormous expansion of the reactive T-cell clones, generating a very large effector cell population. However, to reduce the risk of unregulated proliferation, it is also critical that the activation eventually stops. How does this occur? Several pairs of molecules expressed on the T-cell–APC surfaces convey negative signals to the activated T cell, which have the overall effect of turning off the response. These negative signals include inactivation of molecules activated during the response, inhibition of the cell cycle, and the induction of T-cell exhaustion; T cells that have been repeatedly stimulated to proliferate are no longer able to exert effector function.
One such important negative signal is via CTLA-4 (CD152). Expression of CTLA-4, structurally similar to CD28, is induced on CD4+ and CD8+ T cells within 24 to 48 hours after initial activation by antigen.
CTLA-4 has multiple effects: Once induced, CTLA-4 competes with CD28 for binding to B7 on the APC surface (Figure 11.6). Because it has a higher affinity for B7 than CD28, CTLA-4 outcompetes CD28 and the negative or turn-off signals to the T cell dominate. In addition, the cytoplasmic domain of CTLA-4 contains an immunoreceptor tyrosine-based inhibitory motif (ITIM). The B7–CTLA-4 interaction recruits and activates phosphatases at the CTLA-4 ITIM. The activated phosphatases then remove phosphate groups and thus inactivate molecules inside the T cell that were activated by the B7–CD28 interaction. (In Chapter 8 we described a similar negative function for the B-cell molecule, CD32, which contains an ITIM.) CTLA-4 also acts in the immunologic synapse by displacing critical components of the signaling complex. In this way the expression of CTLA-4 turns off the production of IL-2 and thus ends T-cell proliferation, limiting the extent of the immune response.
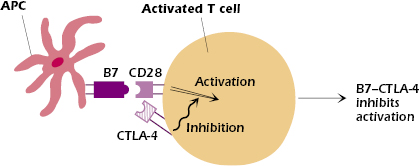
Programmed cell death protein (PD)-1 (CD279), a member of the CD28/CTLA-4 family, is expressed on activated CD4+ and CD8+ T cells and is associated with T-cell exhaustion. It conveys a negative signal to the activated T cell, primarily by inhibiting ZAP-70 function. PD-1 binds to programmed death ligand (PD-L)1 (CD274) and PD-L2 (CD273). PD-L1 is expressed on most tumor cells and many normal cells, and PD-L2 is expressed predominantly by dendritic cells as well as many tumor cells.
The understanding that CTLA-4 and PD-1 transmit negative signals into the T cell is being exploited in several clinical conditions. One example is the testing of treatments using CTLA-4 in autoimmune conditions such as rheumatoid arthritis to turn off T cells that are “inappropriately” activated. In addition, antibodies that interfere with both the B7–CTLA-4 and PD-1–PDL-1/2 interactions are being used to treat chronic conditions, including cancer in which T-cell responses may be “exhausted” and not functioning effectively. Preliminary results of this treatment in melanoma patients have shown prolonged survival, suggesting that inhibition of turn-off signals may be of clinical relevance.
Other Ways to Activate CD4+ T Cells
In the preceding sections, we focused on how a peptide–MHC complex expressed on an APC activates a specific clone of T cells. Since naïve T cells expressing a TCR specific for any one particular peptide (plus MHC) are rare—approximately 1 in 105–106 T cells—only a small fraction of the total T-cell pool is activated by any one peptide–MHC complex. Infectious organisms and other complex antigens do generate more than one peptide–MHC complex, so they stimulate more than one T-cell clone in the course of a normal immune response. Some agents, however, can stimulate many clones of naïve CD4+ T cells to proliferate, which can, as a result, have major consequences for the host. These agents are described in the sections that follow.
Superantigens.
In humans, most superantigens are bacterial toxins from disease-causing organisms. Two examples are the enterotoxin released by staphylococcal organisms (the cause of food poisoning) and the toxin responsible for toxic shock syndrome. As we showed in Figure 9.13 in Chapter 9, a superantigen binds to the Vβ region of a TCR and cross-links it to an MHC molecule expressed by an APC. The superantigen binds to the Vβ chain outside the TCR’s antigen-binding site. Because any one superantigen can bind to many different TCR Vβ molecules, a superantigen can activate huge number of T cells with different antigenic specificities—up to 10% of the total T-cell population—in an individual. The activation of so many T cells and massive release of cytokines into the circulation following superantigen activation can have serious clinical consequences, such as fever and cardiovascular shock and in some circumstances, can even be fatal (see further in Chapter 12).
Plant Proteins, Antibodies to T-Cell Surface Molecules, and Other T-Cell Activators.
Several naturally occurring materials have the ability to trigger the proliferation and differentiation of many, if not all, clones of T lymphocytes. These substances are referred to as polyclonal activators or mitogens because of their ability to induce mitosis of the cell population. The plant glycoproteins concanavalin A (Con A) and phytohemagglutinin (PHA) are particularly potent T-cell mitogens. These substances are lectins, molecules that bind to carbohydrate moieties on proteins. Both Con A and PHA are thought to act through the TCR but not through the antigen-binding site. Because the T-cell response of healthy human blood to Con A and PHA falls in a well-defined range, a low response to Con A or PHA frequently indicates that a person is immunosuppressed. Another plant lectin, pokeweed mitogen, activates both T and B cells.
Some antibodies specific for CD3 have the ability to activate T cells. Since CD3 is expressed on all T cells in association with the TCR, these anti-CD3 antibodies thereby induce all T cells to proliferate. In addition, a combination of phorbol 12-myristate 13-acetate (PMA) and ionomycin is used in vitro to activate all T cells to proliferate and synthesize cytokines. These molecules bypass the TCR: PMA activates PKC and ionomycin activates intracellular levels of calcium.
CD4+ T-Cell Function
Our goal in the sections that follow is to provide a fundamental understanding of the most important functions of CD4+ T cells. Many aspects of the role of CD4+
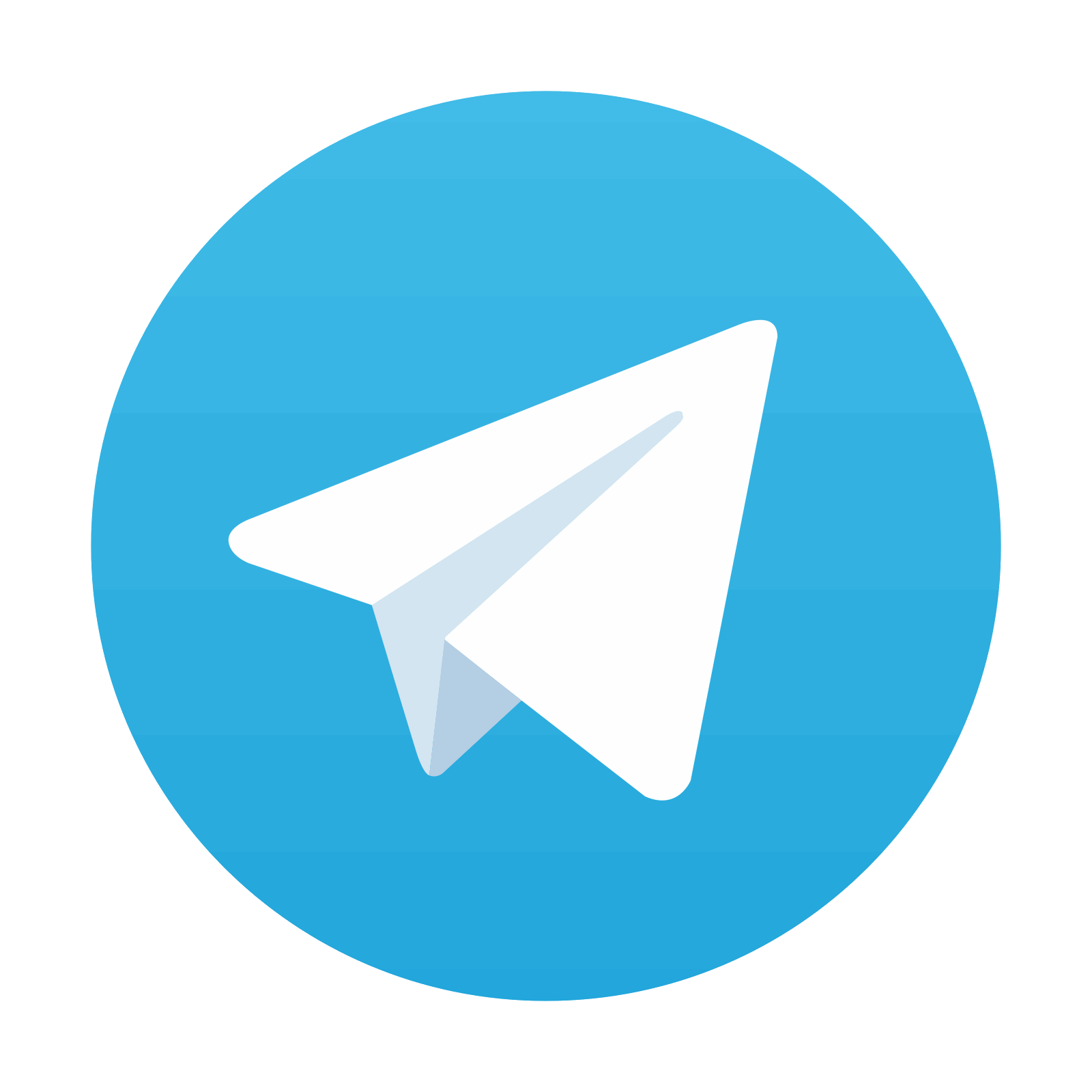
Stay updated, free articles. Join our Telegram channel

Full access? Get Clinical Tree
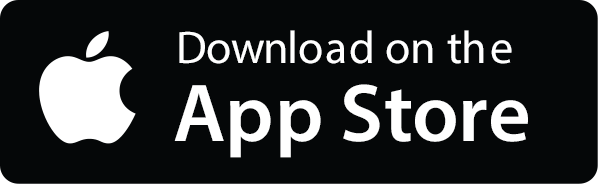
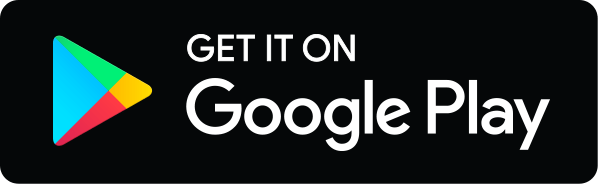