This article gives a general overview of venous thromboembolism (VTE). Pathophysiology, presentation, diagnosis, and initial management of VTE are briefly reviewed. More difficult management problems are reviewed in greater depth, including duration of anticoagulation, treatment of superficial venous thrombosis, and controversies surrounding bridging therapy, with a brief review of currently available new oral anticoagulants.
Thrombosis and its associated complications are a common cause of death throughout the world. Each year, more than 200,000 new cases of venous thromboembolism (VTE) are diagnosed and 30% of these patients die in the first 30 days after diagnosis. In addition, many patients suffer long-term physical consequences from the thrombotic event, resulting in an adverse quality of life and millions of dollars spent for health care.
The pathogenesis of thrombosis results from abnormalities in many components of the vascular and coagulation systems. In 1856, Virchow first described the pathogenesis of thrombosis as the result of defects in the vessel wall, platelets, and coagulation proteins. The interplay of these 3 factors became known as the Virchow triad. Thrombi that form within the venous system are rich in fibrin, resulting from disruption in the coagulation process in areas of blood stasis.
In normal circumstances, the endothelial lining of blood vessels is not a thrombotic surface. Surveillance and maintenance by cellular components of blood interact with the endothelial cells to inhibit thrombus formation. Blood cells, such as platelets, release several antithrombotic factors, such as thrombomodulin and plasmin, which regulate the tissue factor pathway inhibitor system as well as the antithrombin systems. As this is occurring, the platelets limit their own coagulation response with aggregation directly inhibited by prostacyclins and nitric oxide.
When the endothelial surface becomes damaged, the platelets become activated and they no longer guard the endothelium but instead perpetuate the coagulation response. Exposure of vascular collagen in the damaged endothelium also leads to activation of the normal mechanisms of hemostasis, including the coagulation cascade, through exposure of tissue factor leading to hemostasis in the wrong place.
The coagulation cascade is the main mediator of this process. Both the tissue factor–mediated pathway (extrinsic) and the contact-mediated pathway (intrinsic pathway) are based on the systematic activation of inactive enzyme precursors known as serine proteases, which then reflexively lead to activation of the next coagulation factor protein within the coagulation cascade in a chain reaction mechanism ( Fig. 1 ). The final steps lead to cross-linking of fibrin with stabilization of the emerging platelet plug culminating in the formation of a thrombus. The extrinsic and intrinsic pathways are essential to this process, with the main mediator being tissue factor. When tissue factor is released as a result of vascular endothelial injury, factor VII is activated and complexes with tissue factor, setting off the cascade. The steps of coagulation are now set into place with this complex, which next initiates activation of factor X and factor IX. Activation of factor X is essential for conversion of prothrombin (factor II) to thrombin through the prothrombinase complex on activated platelets.
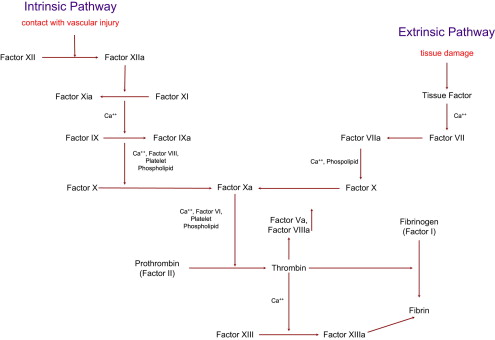
The activated platelets are key players in the thrombotic response and act as the glue that mediates the coagulation cascade. They release many procoagulant proteins, including tissue factor, resulting in uncontrolled hemostasis at the site of vascular injury. The release of these coagulation factors initiates a self-renewing process as the activated platelets recruit additional platelets to the area of injury, establishing the site of thrombus formation. These platelets further activate and become tethered together by binding to exposed glycoprotein Ib-V-IX in damaged collagen through interaction with Von Willebrand factor. More platelets are recruited to the growing thrombus through another receptor present on the platelet surface, GPIIb/IIa, which becomes activated upon platelet activation and undergoes a conformational change leading to increased affinity for fibrinogen, which leads to fibrin deposition. The culmination of this process is internal activation of platelets leading to release of even more proteins including, adenosine diphosphate (ADP), serotonin, and thromboxane A 2 , all of which are essential for recruitment of more platelets to the growing thrombus.
Coagulation is initiated at the molecular level but rapidly progresses to the cellular level, then microvascular level, and ultimately the macrovascular level. Large thrombi can be organ damaging and life threatening. This article reviews the presentation and diagnosis of VTE. Initial treatment of acute VTE is straightforward, noncontroversial, and is covered briefly here. Areas that are less well defined and for which data are limited and conflicting are reviewed in greater detail. These areas include duration of anticoagulation, bridging anticoagulation, treatment of superficial venous thrombosis, and VTE prophylaxis for travel. Two other areas covered in this article include new oral anticoagulants and testing for thrombophilias.
Venous Thromboembolism (VTE): presentation
Patients who develop all 3 components of the Virchow triad of vascular stasis with endothelial damage and hypercoagulability, as described earlier, can form thrombus in a deep vein of sufficient size to result in clinical symptoms. Development of deep venous thrombosis (DVT) alone is roughly twice as common as pulmonary embolism (PE), although the incidence of PE may be underestimated. Those patients who present with classic symptoms of DVT or PE in the context of situations in which the index of clinical suspicion is increased can be readily diagnosed with appropriate testing. Table 1 shows the percentages of patients experiencing clinical signs and symptoms of DVT and PE.
Signs and Symptoms | Percentage of Patients Experiencing Symptoms |
---|---|
Clinical Signs and Symptoms of DVT | |
Swelling | 88 |
Pain | 56 |
Tenderness | 55 |
Warmth | 42 |
Erythema | 34 |
Homan sign | 13 |
Palpable cord | 6 |
Clinical Signs and Symptoms of PE | |
Dyspnea | 77 |
Chest pain | 55 |
Hemoptysis | 13 |
Syncope | 10 |
Sudden death | Rare |
Tachypnea | 70 |
Tachycardia | 43 |
Hypoxia | 18 |
Hypotension | 10 |
Venous Thromboembolism (VTE): diagnosis
Many of these signs and symptoms, especially those associated with PE, can be the result of other pathologic processes. Making the diagnosis of VTE requires both clinical assessment and appropriate diagnostic testing, because clinical diagnosis alone is frequently wrong. Radiographic imaging is required. Missing a diagnosis of DVT or PE can lead to unnecessary morbidity and mortality. However, of the estimated 1 million patients who undergo diagnostic vascular ultrasound each year, only 12% to 25% have positive results. Strategies to decrease the cost burden of negative tests have been studied. Clinical prediction models to determine pretest probability, many of which incorporate use of D-dimer testing and diagnostic algorithms, have been developed to decrease radiographic testing. These models have not yet been validated in large randomized control trials. Detailed reviews of the strategies for diagnosing DVT and PE are covered elsewhere. This article briefly reviews the approach to diagnosis of DVT and PE.
When an extremity DVT is suspected based on clinical presentation and examination, compression duplex ultrasound should be the first diagnostic test. This noninvasive test has high sensitivity (89%–100%) and specificity (86%–100%), especially for proximal DVT (97% and 94% respectively). Venography is rarely used because it requires iodine-based contrast dye, is invasive, and can be technically difficult to perform because of the challenge of obtaining adequate venous access. Magnetic resonance venography (MRV) is excellent for visualization of the pelvic veins and can image proximal veins in the groin and thigh but has high cost and is less commonly available. MRV should not be considered as a first-line test for diagnosis of DVT in an extremity.
Making the diagnosis of PE can be more complex because the list of differential diagnoses associated with presenting signs and symptoms is extensive and imaging to evaluate for PE is more invasive and costly. Helical computed tomography pulmonary angiography (CTA) for diagnosis of PE is the most commonly used test. It has replaced VQ scanning and conventional pulmonary angiography, both of which are significantly more time consuming and cumbersome to perform and provide less information about alternative diagnoses. A definitive diagnosis of another disorder can be made in 50% to 60% of those cases in which PE is not present on CTA. Computed tomography (CT) requires iodine contrast dye and alternative methods must be used in patients with iodine allergy or significant renal failure. MRV is an excellent alternative for those with iodine allergy. As discussed elsewhere, the positive and negative predictive values of CTA vary with the pretest probability of PE.
VTE can occur in other vascular beds including the cerebral sinuses and abdominal vessels. VTE in these locations are often diagnosed with imaging studies for the evaluation of acute onset of headache or abdominal pain. Routine intravenous (IV) contrast CT scanning can usually detect thrombus; however, dedicated CT may be required to adequately define the extent of thrombosis. Patients who have thrombosis in these locations should undergo thorough evaluation for precipitating factors and prothrombotic conditions, including antiphospholipid antibodies and myeloproliferative neoplasms.
Venous Thromboembolism (VTE): diagnosis
Many of these signs and symptoms, especially those associated with PE, can be the result of other pathologic processes. Making the diagnosis of VTE requires both clinical assessment and appropriate diagnostic testing, because clinical diagnosis alone is frequently wrong. Radiographic imaging is required. Missing a diagnosis of DVT or PE can lead to unnecessary morbidity and mortality. However, of the estimated 1 million patients who undergo diagnostic vascular ultrasound each year, only 12% to 25% have positive results. Strategies to decrease the cost burden of negative tests have been studied. Clinical prediction models to determine pretest probability, many of which incorporate use of D-dimer testing and diagnostic algorithms, have been developed to decrease radiographic testing. These models have not yet been validated in large randomized control trials. Detailed reviews of the strategies for diagnosing DVT and PE are covered elsewhere. This article briefly reviews the approach to diagnosis of DVT and PE.
When an extremity DVT is suspected based on clinical presentation and examination, compression duplex ultrasound should be the first diagnostic test. This noninvasive test has high sensitivity (89%–100%) and specificity (86%–100%), especially for proximal DVT (97% and 94% respectively). Venography is rarely used because it requires iodine-based contrast dye, is invasive, and can be technically difficult to perform because of the challenge of obtaining adequate venous access. Magnetic resonance venography (MRV) is excellent for visualization of the pelvic veins and can image proximal veins in the groin and thigh but has high cost and is less commonly available. MRV should not be considered as a first-line test for diagnosis of DVT in an extremity.
Making the diagnosis of PE can be more complex because the list of differential diagnoses associated with presenting signs and symptoms is extensive and imaging to evaluate for PE is more invasive and costly. Helical computed tomography pulmonary angiography (CTA) for diagnosis of PE is the most commonly used test. It has replaced VQ scanning and conventional pulmonary angiography, both of which are significantly more time consuming and cumbersome to perform and provide less information about alternative diagnoses. A definitive diagnosis of another disorder can be made in 50% to 60% of those cases in which PE is not present on CTA. Computed tomography (CT) requires iodine contrast dye and alternative methods must be used in patients with iodine allergy or significant renal failure. MRV is an excellent alternative for those with iodine allergy. As discussed elsewhere, the positive and negative predictive values of CTA vary with the pretest probability of PE.
VTE can occur in other vascular beds including the cerebral sinuses and abdominal vessels. VTE in these locations are often diagnosed with imaging studies for the evaluation of acute onset of headache or abdominal pain. Routine intravenous (IV) contrast CT scanning can usually detect thrombus; however, dedicated CT may be required to adequately define the extent of thrombosis. Patients who have thrombosis in these locations should undergo thorough evaluation for precipitating factors and prothrombotic conditions, including antiphospholipid antibodies and myeloproliferative neoplasms.
Venous Thromboembolism (VTE): initial treatment
Anticoagulation therapy should be initiated once a diagnosis of VTE has been made. The initial goal of therapy is to prevent thrombus propagation and embolization, and to allow for natural fibrinolysis to occur. In a small number of clinical situations, such as in patients with significant hemodynamic compromise or limb-threatening acute venous occlusion, thrombectomy and catheter-directed thrombolysis should be considered. Many groups have made detailed and comprehensive guidelines for use of initial anticoagulant therapy for VTE, including the American College of Chest Physicians (ACCP), American College of Physicians, and American Academy of Family Physicians. The ninth edition of the ACCP Antithrombotic Therapy and Prevention of Thrombosis Guidelines were released on February 8, 2012. These guidelines are derived by consensus agreement after review of available data. The quality and strength of the data are reflected in the grade of the recommendation. The ACCP guidelines are an excellent review of the current data and give evidence-based recommendations for management of antithrombotic therapy; however, individual patient circumstances may dictate modifications of these recommendations for appropriate and successful treatment.
All patients diagnosed with acute VTE should be treated with rapid-onset anticoagulant therapy. In the United States, the only rapid-onset or immediate-onset agents are parenteral. In the European Union in December 2011, rivaroxaban, a new oral direct Xa inhibitor, was the first new oral anticoagulant approved for up-front monotherapy of DVT. The parenteral agents include IV unfractionated heparin (UFH) titrated to partial thromboplastin time (PTT), subcutaneous (SC) UFH, which is monitored with PTT, SC weight-based dose UFH without monitoring, SC low-molecular-weight heparin (LMWH), or SC fondaparinux (a synthetic pentasaccharide Xa inhibitor that works through antithrombin). Although high-quality data from well-controlled studies support the use of any of these agents, LMWH and fondaparinux are preferred agents compared with any type of administration of UFH, as discussed later.
Appropriately selected patients can be treated as outpatients, with no need for hospital admission. The largest number of well-run studies of initial outpatient therapy for DVT have been conducted using LMWHs. These studies show that a variety of types of LMWH are as effective and safe as IV UFH administered in a hospital setting. The use of outpatient therapy significantly affects cost-effectiveness analyses in favor of LMWH and outpatient treatment. For many reasons, initial use of LMWH has become standard of care for treatment of VTE, and is endorsed as such by the ACCP ninth edition guidelines. The hematology consultant needs to consider several factors when recommending the preferred anticoagulant and inpatient versus outpatient management. These factors include the severity of symptoms, the patient’s hemodynamic stability, the risk for major bleeding, the ability to comply with directions, and the availability of outpatient medical support. The availability of the brand of LMWH in local outpatient pharmacies affects the decision about which LMWH is prescribed.
Warfarin can be started on the same day as diagnosis of VTE and initiation of parenteral heparin therapy in those patients who will be treated with warfarin. Parenteral therapy must be continued for at least 5 days, even if the International Normalization Ratio (INR) is in the therapeutic range of 2.0 to 3.0, because it can take this long for levels of prothrombin to decline to reach the full anticoagulant effect of warfarin. Warfarin can also cause a transient hypercoagulable state due to a rapid decline in protein C and protein S levels, rarely leading to warfarin-induced skin necrosis.
Duration of anticoagulation therapy
The initiation of anticoagulation therapy for acute VTE addresses the primary goal of preventing clot propagation and embolization. The secondary goal of preventing recurrent VTE then needs to be considered. The duration of anticoagulation therapy to prevent recurrent VTE is controversial. Conflicting guidelines from different medical societies have been developed with variable fixed time periods recommended. As new data from well-designed studies are presented, evidence-based guidelines are emerging, however, decision making for an individual patient is a complex, risk-benefit analysis. The consultant needs to consider the benefit of continued anticoagulation to mitigate the patient’s risk of recurrent VTE versus the patient’s risk of bleeding on anticoagulation therapy. One of the biggest determinants in the assessment of an individual patient’s possibility for recurrent VTE is whether the VTE event was provoked by a transient or reversible risk factor or whether it was unprovoked or idiopathic. Unprovoked and idiopathic are used interchangeably during this discussion. These terms refer to VTE events in patients in whom a provoking or causative risk factor is not identified. Generally accepted transient risk factors for VTE include bed rest or hospitalization for 3 or more consecutive days within 12 weeks; surgery or general anesthesia within 12 weeks; trauma within 12 weeks; casting or immobilization, especially of the lower extremity; prolonged travel, greater than 4 to 6 hours; combined estrogen and progesterone oral contraceptive use; and pregnancy and the postpartum state. For patients who are in either category of provoked or unprovoked VTE, the assessment of risk and decision for duration of anticoagulation can be straightforward. However, many patients are not in these provoked or unprovoked categories and require individualized assessment.
Consensus guidelines support 3 to 6 months of anticoagulation therapy for a provoked VTE. Studies show that 6 weeks is an insufficient duration of therapy because of a high risk of recurrent VTE. The VTE recurrence rate at 2 years is nearly doubled for 6 weeks compared with 6 months of anticoagulation therapy (18.1% vs 9.5%). A meta-analysis of well-conducted randomized clinical trials shows that the risk of VTE recurrence at 12 months is 40% lower in patients treated for 12 to 24 weeks compared with those treated for 3 to 6 weeks, with no significant difference in major bleeding risk. In this analysis, patients who have only transient risk factors for VTE also benefit from longer anticoagulation treatment; however, the benefit of longer anticoagulation is greater in patients with idiopathic VTE. The risk of recurrent VTE in patients with transient risk factors is estimated at 4% to 5.8% in the first year, and declines after that time period. The annual risk of major bleeding while on warfarin therapy is 1% to 3%. The risk/benefit ratio in a patient with provoked VTE and no other risk factors favors discontinuation of anticoagulation after 3 to 6 months.
For patients with persistent risks associated with recurrent VTE and for those with idiopathic VTE, the optimal duration of anticoagulation therapy after the first 3 to 6 months of treatment is harder to define. In one randomized trial, patients with idiopathic VTE were anticoagulated for 3 versus 24 months. The VTE recurrence rate was higher in the shorter duration treatment group (27.4% vs 1.3% per patient year). This study was stopped at 10 months after interim analysis revealed this significant difference in VTE recurrence rates. Major nonfatal bleeding rate was 3.8% (3 of 79 patients over 10 months of anticoagulation). Similar studies and meta-analyses all suggest that longer duration of anticoagulation therapy decreases recurrence rates; however, the benefit seems to be gained only while the patient is on anticoagulation therapy. In one study of prolonged duration of anticoagulation, final analysis at 3 years revealed no difference in recurrence rate for those patients with idiopathic PE treated for 3 to 6 months versus 12 months (11.2% vs 9.1%). This group found similar results in a study of 3 months versus 12 months of anticoagulation for idiopathic DVT. At 1 year, the recurrent DVT rate was 8.3% for the 3-month arm and 0.7% for the 12-month arm. At 3 years, the DVT recurrence rates were equal at 15.8% and 15.7%.
The decreased risk of recurrent VTE with anticoagulation therapy must be weighed against the risk of bleeding. Many factors affect bleeding risk. Advanced age, renal failure, diabetes, cerebrovascular disease, malignancy, and concomitant use of antiplatelet therapy increase bleeding risk. Bleeding risk is the highest at initiation of anticoagulation, 0.8% per day with UFH in one study. In one registry, risk of major bleeding was 2.4% during the first 3 months of warfarin therapy. As noted previously, the estimated annual risk of bleeding with oral vitamin K antagonist (VKA) therapy is 3%. Fatal bleeding events have been reported to occur in 20% of major bleeds, with annual case fatality of 0.6%. In a study of patients with recurrent VTE, indefinite duration of anticoagulation prevented 0.43 episodes of recurrent thromboembolism per month per 100 patients, but at a rate of 0.20 major hemorrhages per month. A recent large meta-analysis of major bleeding in patients on anticoagulation longer than 3 months found a rate of 2.7 major bleeds per 100 patient years, with a case fatality rate of 9.1%.
Patients with very high risk for recurrent VTE and an unprovoked event clearly benefit from an indefinite duration of anticoagulation because the risk and consequences of recurrence are significantly greater than the risk of bleeding. High-risk patient populations include those patients with multiple recurrent idiopathic events who have failed off anticoagulation therapy; patients with antiphospholipid syndrome; patients with active cancer and VTE; patients with high-risk inherited thrombophilia and unprovoked VTE, including those with protein S, protein C, and antithrombin deficiencies, homozygous factor V Leiden; or compound factor V Leiden/prothrombin gene G20210A heterozygotes. Strong data for VTE recurrence rates in the inherited thrombophilias are lacking; however, risk is extrapolated from data for risk for first VTE event. Individual patient assessment of bleeding risk may affect the decision for indefinite anticoagulation despite the high risk of recurrent VTE in any of these patient groups, especially in those with malignancy. In one study, the 1-year incidence of recurrent thrombosis was 21% for patients with cancer compared with 6.8% for patients without cancer; however, the 12-month cumulative incidence of major bleeding was 12.4% in patients with cancer and 4.9% in other patients.
Many patients with VTE do not clearly fit into the categories of provoked or unprovoked with high risk of recurrence. They often have a unique combination of risks that give them an intermediate risk of recurrence that can be difficult to quantify or to place neatly into the category of provoked VTE and no need for further anticoagulation or the unprovoked category with high risk that benefits from anticoagulation despite bleeding risk. Many balk at the idea of lifelong anticoagulation therapy. Risk for major bleeding events is a substantial concern but also the economic and psychological burden of testing and impact on lifestyle are significant concerns. Rather than trying to rigidly categorize patients into groups favoring or not favoring indefinite anticoagulation therapy, individual risk assessments should be performed. A detailed patient history, as well as family history, events surrounding the development of VTE, severity of symptoms at presentation, and clot burden should be reviewed. Patient preference for ongoing anticoagulation is also a significant factor that is incorporated in the decision-making process. Some patients are willing to take the risk of approximately 10% to 12% per year of recurrent VTE and forgo anticoagulation, whereas for others the risk and concern for developing fatal PE is too high.
Strategies to help determine individual risk of recurrence have been developed and evaluated. Testing D-dimer levels and use of flexible duration of anticoagulation instead of a fixed time period have been studied. Clinical prediction tools to better quantify individual risk of recurrence and of bleeding risk have been designed and are being validated. Trials of lower intensity anticoagulation have also been performed. The development of new oral anticoagulants that do not require monitoring might significantly affect the risk-benefit analysis for some patients.
D-dimer levels have been used to risk stratify patients with idiopathic VTE into groups with high risk and low risk of recurrence. In most studies, patients with idiopathic VTE complete a specified course of anticoagulant therapy and then have D-dimer levels measured, often 1 month after discontinuation of anticoagulation. Several studies and meta-analyses have been performed confirming that patients with increased D-dimer levels have a significantly higher risk of recurrent VTE than those with normal levels. In one randomized study, patients with positive levels who remained on anticoagulation had a recurrence rate of 2%. Those who had normal levels and came off anticoagulation had a recurrence rate of 4.4%. Those with positive levels allocated to no anticoagulation had an annual recurrence rate of 10.9%.
A recent meta-analysis of 1818 patients with unprovoked VTE showed that the timing of D-dimer testing after stopping anticoagulation, patient age, and threshold D-dimer level for positive results (250 μg/L vs 500 μg/L) did not affect the ability of the D-dimer level to distinguish risk for recurrent VTE. In a prospective cohort study, the PROLONG II study, serial D-dimer levels were evaluated in patients with an unprovoked VTE who had a normal D-dimer level 1 month after stopping anticoagulation. Patients who developed positive D-dimer levels during the ensuing year had increased risk of recurrence compared with those whose D-dimer levels remained normal. A newly positive test at 3 months that remained positive conferred a hazard ratio of 7.9 for recurrence (27 per 100 patient years vs 2.9 per 100 patient years). Patients who developed a positive result after 3 months had an intermediate risk of recurrence at 11.1 per 100 patient years.
Another approach to determining risk for recurrence is to repeat imaging studies to assess for recanalization. Residual thrombosis at the time of discontinuation of anticoagulant therapy is a risk for recurrent VTE, with a hazard ratio of 2.4. A randomized trial was performed by the same investigators to assess fixed duration of anticoagulation versus flexible duration of anticoagulation based on serial ultrasound assessments of DVT. Patients assigned to fixed-duration treatment received 3 months for provoked DVT and 6 months for unprovoked DVT. Those assigned to flexible duration had anticoagulation stopped at 3 months if there was complete recanalization of veins, or continued for up to 9 months for provoked DVT and 21 months for unprovoked. The numbers of patients in each group were insufficient to detect effectiveness and risk of major bleeding. Overall, the rates of recurrent DVT were significantly lower at 11.9% in those receiving flexible-duration treatment versus 17.2% receiving fixed-duration treatment.
Clinical prediction rules have been designed that incorporate these and other factors that have been shown to increase the risk of recurrence in patients with an unprovoked VTE. These factors include (1) male gender, (2) increased body mass index (>30 kg/m 2 ), and (3) initial presentation with symptomatic PE versus proximal DVT versus isolated calf vein thrombosis. Two scoring systems have recently been developed to predict risk of recurrence after idiopathic VTE. The Vienna Prediction Model uses a nomogram to obtain a number value to predict individual risk. Several potential risk factors, including biochemical markers of thrombosis, were assessed. The factors that most strongly predicted recurrence included (1) male sex, (2) PE, and (3) increased D-dimer level after stopping anticoagulation. Another clinical scoring system that goes by the mnemonic men and HERDOO2 (male sex and signs of postthrombotic syndrome, including hyperpigmentation of the lower extremities, edema or redness of either leg, a D-dimer level >250 μg/L, obesity [body mass index >30 kg/m 2 ], and older age [>65 years]) has been developed based on prospective data. It is currently being validated in a larger patient population.
The use of a lower INR target, or lower intensity warfarin anticoagulation therapy, has been assessed in 2 large, double-blind, randomized studies. The proposed benefits of lower intensity are similar reduction in recurrent VTE risk but with lower risk of bleeding, and need for less frequent INR testing. In the PREVENT (Prevention of Recurrent Venous Thromboembolism) trial, a target INR of 1.5 to 2.0 was compared with placebo in patients with idiopathic VTE after a median of 6.5 months of full-intensity warfarin treatment. There was a 64% reduction in risk of recurrent VTE events in the 255 patients assigned to low-intensity warfarin (7.2 per 100 patient years) compared with 253 patients assigned to placebo (2.6 per 100 patient years, hazard ratio 0.36, 95% confidence interval 0.19–0.67). The rates of major bleeding were not statistically different at 0.4 per 100 patient years in the placebo group and 0.9 per 100 patient years in the low-intensity warfarin arm. In the ELATE (Extended Low-intensity Anticoagulation for Unprovoked Venous Thromboembolism) trial, patients completed 3 or more months of standard anticoagulant therapy and were then randomized to low-intensity warfarin with a target range of 1.5 to 2.0 or conventional intensity with the standard 2.0 to 3.0 range. The low-intensity arm had a recurrent VTE rate of 1.9 events per 100 patient years; the conventional arm had 0.7 recurrent VTE events per 100 patient years. There was no difference in rates of major bleeding between the 2 arms. The low-intensity group had 1.1 major bleeds per 100 patient years and the conventional-intensity group had 0.9 per 100 patient years. A criticism of the ELATE trial is the unexpectedly low bleeding risk compared with most previously published results in the 2.0 to 3.0 arm. INR tests were performed every 24 days in the conventional warfarin arm and every 26 days in the low-intensity arm. Time in therapeutic range values were reported and were 63% for the low-intensity arm and 69% for the conventional-intensity arm. Although these values are high, they are now routinely reported by many dedicated anticoagulation management services and may be attainable in a community setting. Compared with no long-term anticoagulant therapy, the low-intensity groups from both studies experienced a 76% risk reduction for recurrent VTE; the conventional-intensity arm had a greater than 90% risk reduction.
The WARFASA (Warfarin vs Aspirin) study was presented at the American Society of Hematology conference in 2011. Four-hundred and two carefully selected patients with idiopathic VTE were randomized to receive aspirin 100 mg daily or placebo after 6 months of warfarin treatment. Patients on treatment with aspirin had a recurrent VTE rate of 5.9% per patient year compared with 11.0% per patient year in the placebo-treated group (hazard ratio 0.57). Bleeding rates were the same in each group and were extremely low. Risk reduction with aspirin was 40%. In some patients with idiopathic VTE, such as those with high risk of bleeding with conventional anticoagulation, those with a first idiopathic event and otherwise low-risk assessment, and those who do not want to comply with anticoagulation treatment, aspirin could offer some level of protection against recurrence; however, further analysis, longer follow-up, and head-to-head comparison with warfarin is required before this approach can be fully endorsed.
Patients who have had an unprovoked VTE have an increased risk of recurrent VTE if anticoagulation therapy is stopped. Current consensus is to treat for a minimum of 6 months with full-intensity anticoagulation therapy, usually with warfarin. Extending treatment past 6 months with full-intensity warfarin therapy results in the most significant decrease in risk for recurrent events, but with the costs of increased bleeding risk, monitoring, and impact on quality of life. For patients in whom the risk-benefit analysis of continued duration of anticoagulation is not straightforward, an individualized risk-benefit assessment using a variety of the strategies described earlier is performed by most clinicians in the decision-making process. Patient preference is also considered. Although many of these approaches, such as vascular reimaging and use of D-dimer algorithms, have not been rigorously validated, they are widely practiced. Because of the lack of definitive data, none of these practices has been endorsed by any major society’s guidelines. Clinicians in practice need to make decisions despite the sometimes conflicting results of trials and the differences of expert opinions. The use of D-dimer testing, repeat vascular imaging, and use of clinical prediction rules or scoring systems can help the clinician decide on an appropriate treatment course for the individual patient. This decision should be reevaluated as new data emerge that better define the true risk of recurrent VTE for an individual patient.
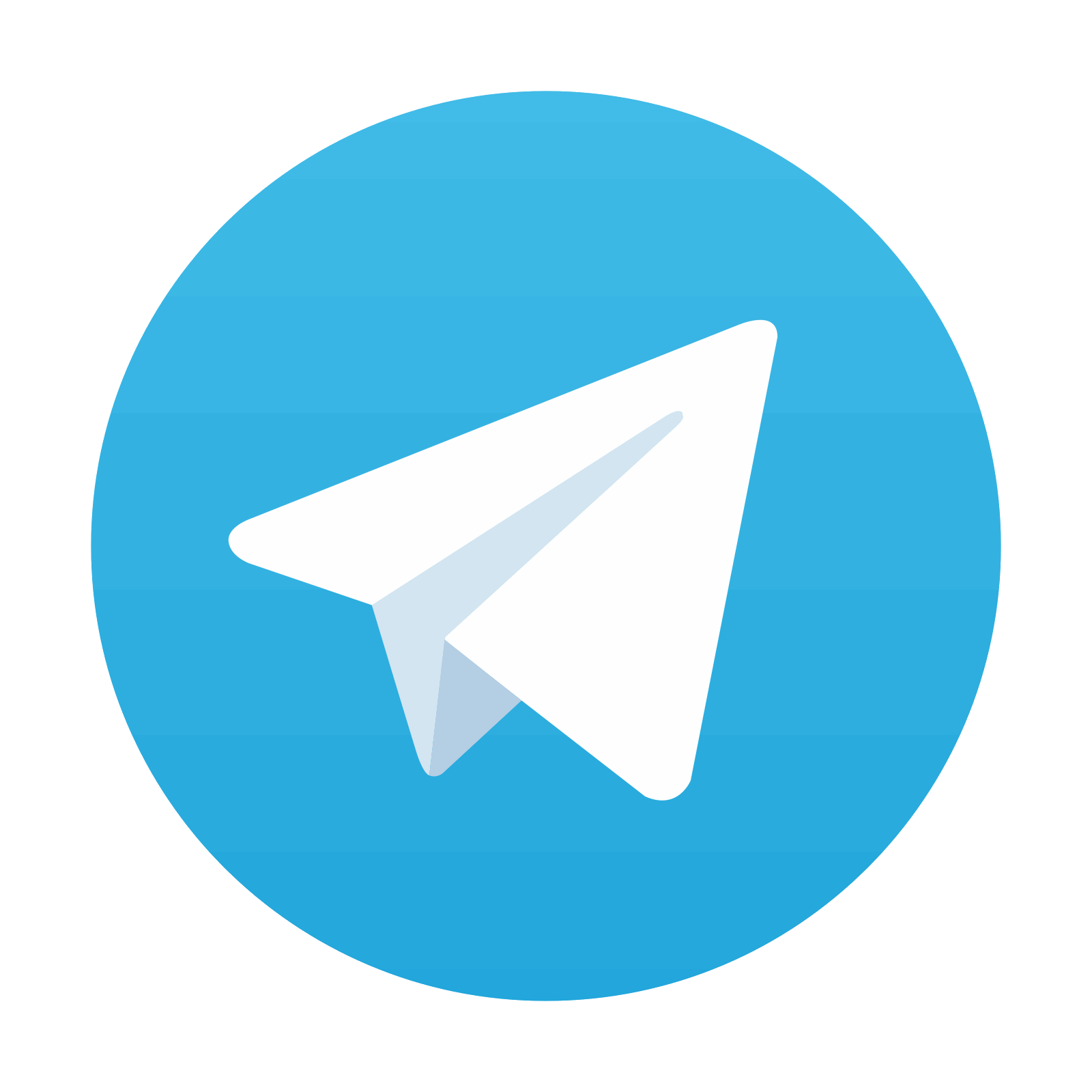
Stay updated, free articles. Join our Telegram channel

Full access? Get Clinical Tree
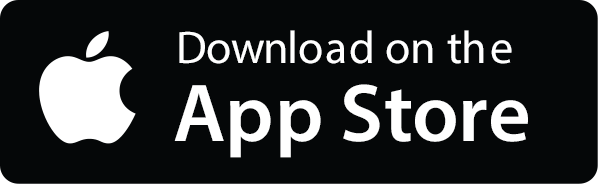
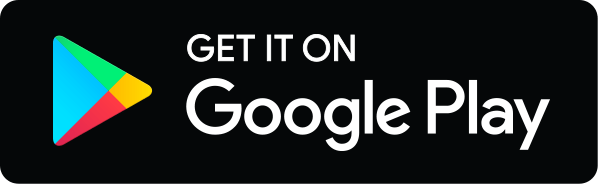