Existing data and nontest information
All available (eco)toxicological data from standardized or nonstandardized tests
Read across, chemical categories, quantitative structure activity relationships (QSARs) and other in silico predictions, and absorption-distribution-metabolism-excretion (ADME) model predictions
In vitro assays providing data about selected endocrine mechanism(s)/ pathways(s) (mammalian and nonmammalian methods)
Estrogen receptor transactivation (OECD TG 455)
Androgen or thyroid transactivation (if/when TGs are available)
Steroidogenesis in vitro (OECD TG 456)
MCF-7 cell proliferation assays (ER ant/agonist)
Other assays as appropriate
In vivo assays providing data about selected endocrine mechanism(s) / pathway(s)
Hershberger assay (OECD TG 441)
Amphibian metamorphosis assay (OECD TG 231)
Fish Reproductive Screening Assay (OECD TG 229)
Fish Screening Assay (OECD TG 230)
Androgenized female stickleback screen (GD 140)
In vivo assays providing data on adverse effects on endocrine relevant endpoints 2
Repeated-dose 90-day study (OECD TG 408)
One-generation assay (OECD TG 415)
Male pubertal assay (see GD 150 Chapter C4.3)
Fish Reproduction Partial Life Cycle Test (when/if TG is available)
Larval Amphibian Growth and Development Assay (when TG is available)
Intact adult male endocrine screening assay (see GD 150 Chapter Annex 2.5)
Prenatal developmental toxicity study (OECD TG 414)
Chronic toxicity and carcinogenicity studies (OECD TG 451-3)
Reproductive screening test (OECD TG 421 if enhanced)
Combined 28-day/reproductive screening assay (OECD TG 422 if enhanced)
Developmental neurotoxicity (OECD TG 426)
Mollusc Partial Life Cycle Assays (when TG is available)
Chironomid Toxicity Test (TG 218–219)
Daphnia Reproduction Test (with male induction) (OECD TG 211)
Earthworm Reproduction Test (OECD TG 222, 2004)
Enchytraeid Reproduction Test (OECD TG 220, 2004)
Sediment Water Lumbriculus Toxicity Test Using Spiked Sediment (OECD TG 225, 2007)
Predatory mite reproduction test in soil (OECD TG 226, 2008)
Collembolan Reproduction Test in Soil (TG OECD 232, 2009)
In vivo assays providing more comprehensive data on adverse effects on endocrine relevant endpoints over more extensive parts of the life cycle of the organism
Two-generation assay (OECD TG 416 most recent update)
Medaka Multigeneration Test (MMGT) (when TG is available)
Avian two-generation reproductive toxicity assay (when TG is available)
Mysid Life Cycle Toxicity Test (when TG is available)
Copepod Reproduction and Development Test (when TG is available)
Sediment Water Chironomid Life Cycle Toxicity Test (OECD TG 233)
Mollusc Full Life Cycle Assays (when TG is available)
Daphnia Multigeneration Assay (if TG is available)
Scrutiny of the CF shows that, as of mid-2011, three EDC-sensitive fish-based procedures have been published as OECD test guidelines (TGs), two at Level 3 (TG 229, the Fish Reproductive Screening Assay; and TG 230, the 21-Day Fish Screening Assay) and one at Level 4 (TG 234, Fish Sexual Development Test) [20,27,28]. The first two are primarily intended as relatively quick screens for endocrine activity rather than as apical tests, and are described in Section 7.3 of this chapter. A variant of TG 230 with high sensitivity to anti-androgens, the Androgenized Female Stickleback Screen (AFSS), also at Level 3, has recently been published as OECD Guidance Document (GD) 148 [29]. At Level 4, the Fish Sexual Development Test (FSDT; TG 234) has just been published, and can be considered as providing apical partial life cycle data of potential use in a risk assessment. It is described separately in Section 7.4. Finally, Level 5 of the CF does not at present contain any validated TGs for fish. A recent OECD Detailed Review Paper [30] recommended that a TG or TGs on fish life cycle testing should be developed to help populate Level 5, and these are described in Section 7.5. The parts of the fish life cycle covered by these various categories of screens/tests are shown diagrammatically in Table 7.2.
Table 7.2 Parts of the fish life cycle covered by various screens and tests for endocrine-disrupting chemicals
Although this suite of existing and planned fish-based procedures might seem comprehensive, it should be borne in mind that the screens and partial life cycle tests are not sensitive to all EDC modalities. Thus, for example, the fish screens are relatively insensitive to thyroid-active or corticosteroid-active substances, so tests with other taxa (e.g., amphibians and birds) are also under development (see Chapters 8 and 10). Furthermore, the fish screens are also expected to be insensitive to some substances that interfere with the endocrine systems of invertebrates, so a range of apical tests with arthropods (insects and crustaceans) and molluscs are planned or in development (see Chapters 4, 5 and 6).
7.3 FISH-BASED SCREENS DEVELOPED BY OECD FOR ENDOCRINE-DISRUPTING CHEMICALS
The two main OECD fish screens, TG 229 and 230 [20,27], are essentially designed to detect a defined range of potential EDCs and not to provide apical data of significant use in a definitive risk assessment (but see TG 229 further down Section 7.3). Both screens are sensitive to strong and weak estrogens, anti-estrogens, androgens, and aromatase inhibitors, but their sensitivity to anti-androgens is questionable, and they are not known to be sensitive to other EDC modalities. Due to the complexities of some types of endocrine disruption, they do not necessarily provide definitive data on modes of action, so generally they should be used in conjunction with in vitro methods designed to reveal relevant receptor interactions or enzyme inhibition. One of their advantages over current in vitro methods is, of course, their ability to detect potential EDCs that have been metabolically activated. Another advantage over in vitro methods is the fact that these and other in vivo assays involve fully functioning endocrine systems with intact hypothalamus-pituitary-gonad axes.
The simpler and more rapid of the two is TG 230 [27], the 21-Day Fish Screening Assay. It uses adult fish from one of three species (fathead minnow, Pimephales promelas; zebrafish, Danio rerio; medaka, Oryzias latipes) and exposes them to three test concentrations (plus a positive control) for three weeks. In each species, a key end point in both sexes is measurement of the yolk precursor protein VTG, usually in blood plasma. This may be induced in males by estrogens or aromatizable androgens, or depressed in females by anti-estrogens or aromatase inhibitors. If a depression in female VTG is seen, it is important to check that this is not due to any systemic toxicity. The other key end point (in fatheads and medaka only) is the induction of male secondary sexual characteristics in females by androgens. In fatheads, the characteristic in question is the appearance of nuptial tubercles on the snout, which in maturing males is under the control of 11-keto-testosterone (11-KT). Exogenous androgens are able rapidly to induce these tubercles in females. In medaka, an analogous response to exogenous androgens is the induction of papillary processes on the anal fin in females, but there are no quantifiable secondary sexual characteristics in zebrafish.
During validation of TG 230, the effects of anti-androgens on male secondary sexual characteristics were investigated, and some reductions of these were observed. However, this end point was found to have low statistical power and is therefore not recommended for use without caution. An alternative and more robust in vivo test for anti-androgens is the AFSS, which uses the three-spined stickleback, Gasterosteus aculeatus. This screen has been fully validated [31] and has been published as OECD GD 148 [29]. The kidneys of stickleback males produce a proteinaceous glue called spiggin that is used for nest building. Spiggin induction is under the control of 11-KT, and it can be induced by exogenous androgens in females. The AFSS exploits this as a biomarker by exposing sexually mature females to both a single concentration of an androgen (5 μg/L dihydro-testosterone; DHT) and to one of three concentrations of the suspected anti-androgen for three weeks. Both strong and weak anti-androgens sensitively cause the induced renal spiggin to decline in concentration, although care should be taken to ensure that there is no systemic toxicity that could mimic this effect. Furthermore, the test is also sensitive to androgens, which specifically cause an increase in renal spiggin in the negative control group (no DHT; test substance at highest concentration) or in the treatment groups by comparison with the effects of DHT alone (positive control group).
A more time-consuming variant of TG 230 is the Fish Reproductive Screening Assay (TG 229 [20]), which employs the same species and end points as TG 230 but also includes measurement of fecundity and (optionally) gonadal histopathology. Fecundity is observed for one to two weeks before the three-week exposure begins, so the complete test can take five weeks (rather long for a test designed for screening chemicals, although it is being used for this purpose by EPA EDSP). Fecundity in the test species also generally has a high coefficient of variation ranging from 20 to 60 percent, and at the upper end of this range, the power of the test to detect a 50 percent decrease in egg production is unacceptably low.
Despite these drawbacks, the fecundity end point in TG 229 is an apical response that could potentially be used in a risk assessment, although some warnings about this use are nevertheless required. First, a reduction in fecundity is not necessarily diagnostic of endocrine disruption, because many reproductive toxicants and other nonspecific parameters (e.g., low dissolved oxygen in aquaria) can affect this end point. Second, as only three concentrations of test substance are employed, the precision of an estimate of the no-observed-effect-concentration (NOEC) or Effect concentration affecting x% of the population (ECx) is low. Third, the exposure is relatively short (three weeks), and some substances may affect fecundity only over longer time scales. Consequently, an apparent absence of effect on fecundity in TG 229 cannot be taken as definitive, while if an effect is measured, it generally would be necessary to follow this up with a more comprehensive test using more exposure concentrations (e.g., a fish life cycle test).
In summary, validated and internationally standardized in vivo
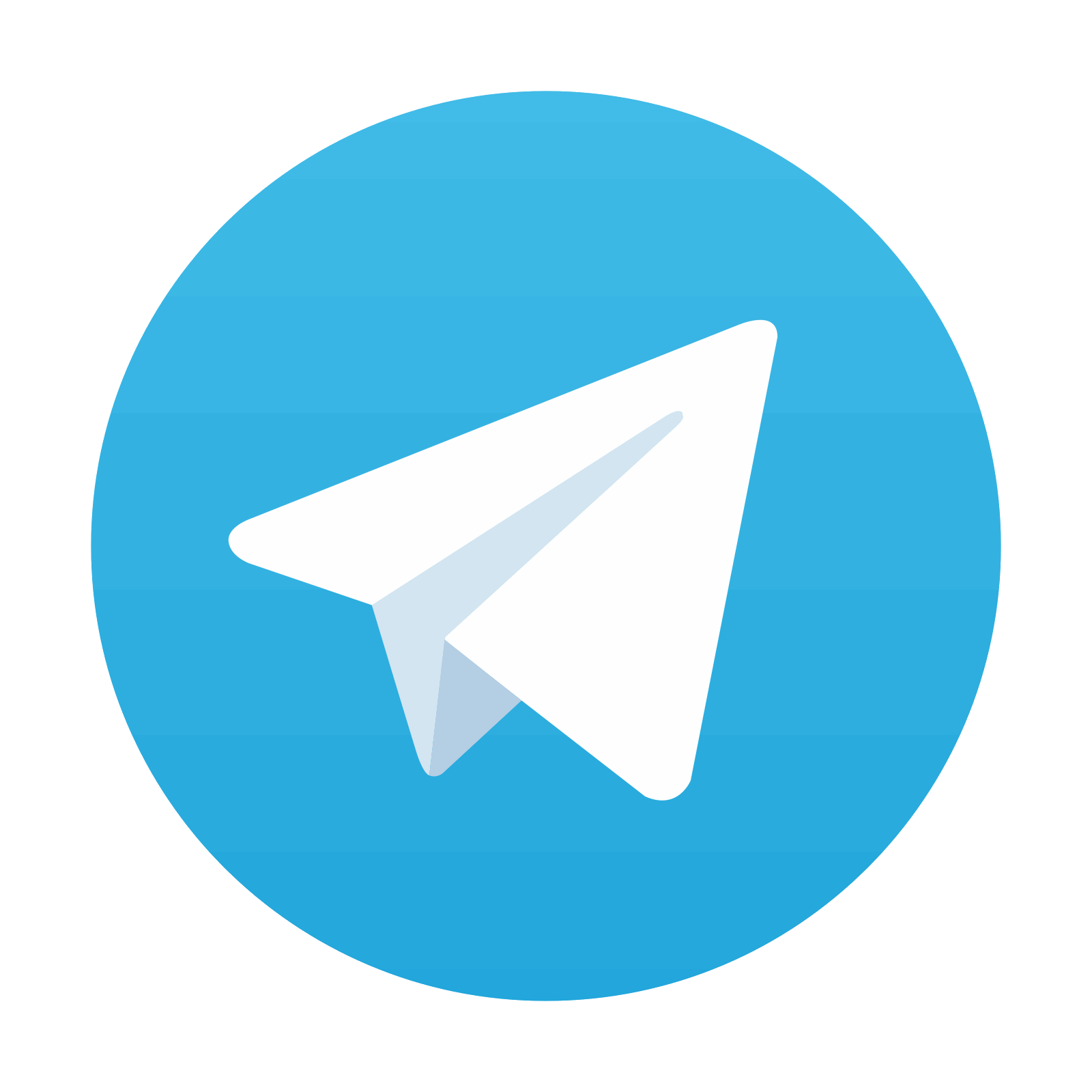
Stay updated, free articles. Join our Telegram channel

Full access? Get Clinical Tree
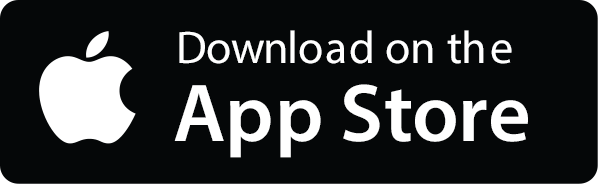
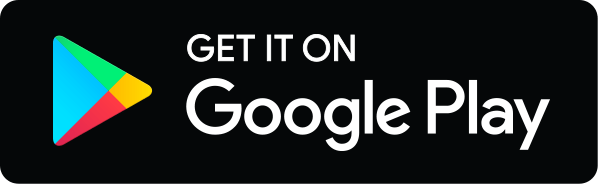