FIGURE 9.2 Temperature-dependent sex determination in three reptilian species: American alligator, Alligator mississippiensis; Red-eared slider turtle, Trachemys scripta; Alligator snapping turtle, Macroclemys temminckii. Sex ratio was significantly altered by egg-incubation temperature in all three species. Source: Modified from [19,20].
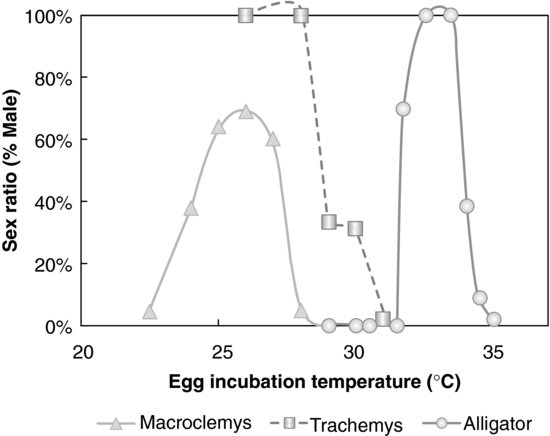
Over the last decade, significant work has been performed to establish the genetic pathways associated with TSD, much like the work examining GSD (for review, see [23]). Our laboratory, like others, has searched for common thermo-sensitive factors, such as the temperature-sensitive heat-shock proteins [24] that could contribute to TSD. However, to date, the key molecule that would initiate and establish the pathways leading to ovarian or testicular differentiation, whose expression should be directly sensitive to temperature, is still unknown in reptiles. What has been established is that the same genes that are associated with GSD in mammals are common to the process of GSD and TSD in nonmammalian species [23,25]. For example, although nonmammalian amniotes lack the sex-determining region Y (SRY) gene on sex chromosomes, the Y chromosome gene critical for sex determination in eutherian mammals, the other genes essential for testicular development, for example, are expressed in the development of the archosaurian and reptilian testis [26–29]. Sex appears to be a digital system; that is, one is either male or female. However, it is now widely accepted that, although the general anatomy of the reproductive system is male or female, much of the sex is expressed as a continuum, with variation in multiple parameters, including plasma hormone concentrations, gene expression profiles, and behavior. Animals exhibiting TSD therefore may be more “fragile” than GSD animals in that the sex determinations in the former are affected by environmental factors, in this case incubation temperature during a narrow window of developmental time. Thus, TSD animals could be useful models to analyze potential alterations in physiological and genetic responses to environmental factors, including chemical/pollutant inputs.
The genetic trigger(s) for TSD is still under study, but it is accepted that estrogens play a critical role in both TSD and GSD in sauropsids. That is, both of these sex determination systems can be overridden by the administration of a natural or synthetic estrogenic compound under male-producing conditions (MPT, ZZ, or XY). In contrast, the administration of an aromatase inhibitor (blocking the enzyme aromatase that synthesizes estrogens) under female-producing conditions (FPT, ZW, or XX) induces sex reversal in some species [30,31] (see Figures 9.3 and 9.4; Tables 9.1 and 9.2). However, the mechanism for sex reversal in a TSD species has not been fully clarified. It is well established that an exogenous estrogenic signal can induce sex reversal—male to “female”—in sauropsids with TSD; thus, these species could be affected by estrogenic or anti-estrogenic EDCs. In addition, because sex can be determined in a number of these species by temperature and it appears that the majority of the underlying genetic factors are common among vertebrates for gonadal determination and differentiation, species with TSD are excellent models to investigate the effects of EDCs on these processes. Finally, as temperature influences sex determination, changes in climate could also influence these processes. What has become increasingly obvious in the field of ecotoxicology is that examining contaminants alone, even if mixtures of contaminants are used, still does not predict outcomes in real-world situations. Real-world populations are exposed to multiple stressors, including changes in climate, microclimate, and diet; varying abiotic factors, such as oxygen partial pressure and carbon dioxide partial pressure, pH, and temperature; as well as contaminant loads [5]. Studies of wildlife populations are one of the few approaches that allow the examination of genetically diverse populations—similar to human populations—for associations between these variables and health outcomes. Importantly, studies of some wildlife populations (e.g., TSD species) also allow us to conduct experiments to test those associations for causation.
FIGURE 9.3 Temperature-dependent sex determination (TSD) and sex reversal at male-producing temperature (MPT) in the American alligator. When eggs are incubated at MPT during thermo-sensitive period (TSP), the embryo develops a testis and become male 100 percent of the time. In contrast, egg incubation at female-producing temperatures during the TSP produces females 100 percent of the time. However, male induction at a MPT can be overridden by the administration of estradiol-17β (E2) during the TSP. If egg incubation temperature is at the intermediate temperature of 31.5°C, neonates exhibit a 1:1 sex ratio.
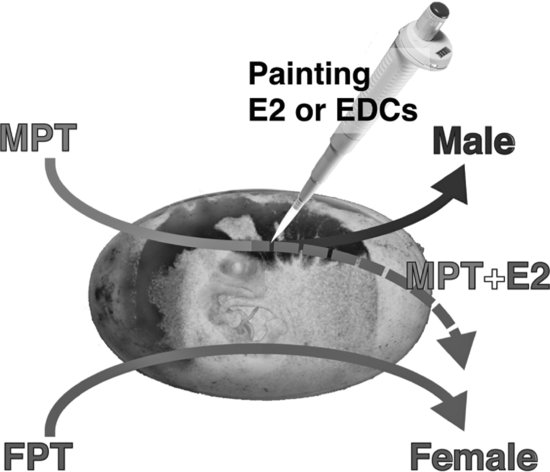
FIGURE 9.4 Sex reversal induced by estradiol-17β (E2) and endocrine-disrupting contaminants (EDCs) in (A) the American alligator at an intermediate temperature and (B) Red-eared slider turtle at a male-producing temperature. Source: Modified from [18,50].
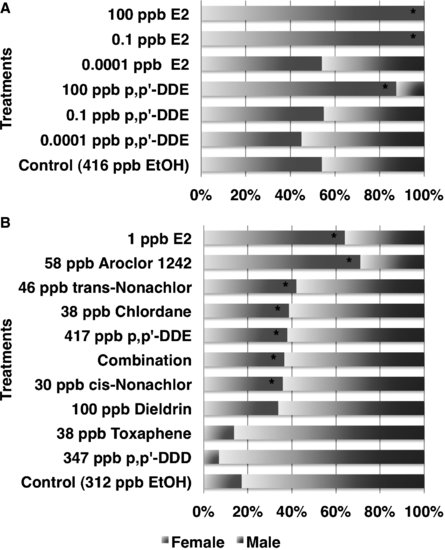
Table 9.1 Sex reversal induced by hormonal manipulation in sauropsids
Table 9.2 Reptilian species examined for sex reversal following treatment with DDT metabolites
The American alligator is the best-studied species among crocodilians in regard to TSD and ecotoxicology. The species exhibits TSD, and we have reported on reproductive and endocrine alterations observed in wild contaminant-exposed populations as well as experimentally exposed animals in the laboratory (for reviews, see [4,32]). Thus, for the remainder of this chapter we focus on the embryonic development and TSD of American alligators and outline our approaches to the investigation of EDCs in this model archosaurian species.
9.2 APPROACHES TO EXAMINE EFFECTS OF EDCS
9.2.1 Screening Chemicals for Receptor Activity
The endocrine system consists of multiple components: endocrine regulation by the hypothalamus of the central nervous system, hormone production and storage by various endocrine glands, hormone clearance by the liver and/or kidneys, and cellular-level sensitivity determined by receptors on cells at the target tissue. All of components work together to maintain homeostasis. Thus, perturbations or irreversible alterations could cause a variety of serious and chronic problems in victim organisms. Although in vivo experiments are very important to understand the effects of EDCs on the endocrine system at the organismal level, in vitro investigations can be conducted to screen chemicals for their potential to interact with a given receptor as well as to understand their mechanism of action without the need to kill animals.
The luciferase transactivation assay, a reporter gene assay, has been used to understand the potential impact of an EDC on the molecular mechanisms associated with transcription. Specifically, this assay has been used to investigate the activity of transcription factors such as the nuclear hormone receptors (NHRs) (e.g., the steroid or thyroid hormone receptors). However, it is important to recognize that EDCs not only affect NHRs; if they mimic endogenous steroids, they also could interact with steroid hormone receptors found in the cell membrane. The E-screen (MCF-7 cell-based assay) and YES (yeast estrogen screen) assays are well established and are reasonable screening systems for EDC interaction with human estrogen receptor-alpha (ERα; ESR1) [33,34]. To briefly review, vertebrates have at least two forms of the nuclear estrogen receptor, estrogen receptor-alpha (ERα; ESR1) and estrogen receptor-beta (ERβ; ESR2). Both receptors play a critical role in development, sex determination, sexual differentiation, and maturation as well as reproductive cyclicity. Generally, ESR1 is expressed in reproductive accessory organs in mammals, whereas ESR2 is expressed in the gonad and brain. Previous studies have suggested that ESR2 is reactive with a wide array of exogenous estrogenic compounds [35]. However, there is no established system to analyze ESR2 actions among vertebrate species. Thus, both ESR1 and ESR2 need to be investigated to estimate the estrogenicity of EDCs.
Endocrine disruption via thyroid or adrenal hormone action have also been reported [3,19,36]. Further, given that species variation exists in the amino acid sequence of the ligand-binding region of the ESR [24,37–41], variation in response could exist; that is, an estrogen in one species might not be estrogenic in another, or a weak estrogen in one species might be significantly more potent in another. Thus, the ability to have a species- or receptor-specific system to predict EDCs influences in wildlife would be useful.
One of the potential cascades by which EDCs can have an effect is through the NHR, which are ligand-dependent transcription factors. Once the ligand-binding domain (LBD) of the receptor binds the ligand, the DNA binding domain (DBD) of the receptor, with other various transcriptional cofactors and regulatory proteins, can attach to the hormone response element (HRE) of the promoter of a target gene and regulate transcription. The DBD of each NHR is highly conserved among vertebrate species, but this DBD is not conserved among the NHRs; thus, each receptor can regulate the transcription of a unique suite of genes. Typical HREs are half-palindromic sequences that can activate the transcription with most vertebrate NHRs. However, the HREs for each receptor type are not completely conserved among species. Thus, our research group has worked to produce two versions of the transactivation assay, one that requires ligand + receptor – HRE interaction and a second that requires ligand + receptor interaction but does not require recognition of a HRE. The second system works via the modified GAL4 (yeast transcription activator protein Gal4) system could be more useful for screening EDCs on different types of NHRs from different species (see Figure 9.5) [42]. To date, our research group has used the GAL4 system to examine endogenous estrogenic hormone and pharmaceutical interactions with the ESRs from several reptiles: a freshwater turtle, two snakes, and an alligator [24,38]. These data demonstrate that the GAL4 system would also be effective in determining whether contaminants commonly found in a wild environment, including wildlife blood plasma, have the potential to bind the receptor and induce gene expression. The GAL4 system has benefits over earlier systems that used purified receptors from the alligator uterus, where we could demonstrate binding of the receptor (both the ESR and progesterone receptors) and displacement of the endogenous hormone by various contaminants but could not show actual activation of transcriptional activity [43].
FIGURE 9.5 Dose response curve using the in vitro trans-activation assay with the American alligator estrogen receptors [ESR1 (ERα); ESR2 (ERβ)] and red-bellied slider turtle androgen receptor. The maximum induction by endogenous ligand was plotted as 100% induction. Each receptor revealed their own specificity in response to the exogenous ligands.
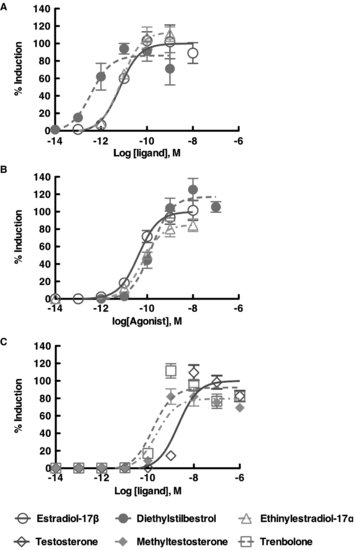
9.2.2 Source of RNA to Clone Nuclear Hormone Receptors for In Vitro Screening
In order to conduct the transactivation assay, a cDNA of the target receptor is needed. Ideally, it is best to have a full-length cDNA for the receptor of interest, but the ligand-binding domain alone could work, depending on the receptor type and species (see [38]). Fresh tissues from which to obtain intact RNA are readily available from common laboratory animals, such as mouse, rat, chicken, zebrafish, or similar species. Conversely, when working with wildlife, particularly endangered species, it is impossible to obtain fresh tissue as samples for RNA extraction. That is, one cannot kill these animals; thus, one has almost no chance to obtain fresh tissue from organs for RNA extraction. However, if less invasive samples (e.g., skin biopsy, blood sample) can be obtained, one can clone cDNAs for many genes including most nuclear hormone receptors, such as ESR1, androgen receptor (AR), progesterone receptor (PR), glucocorticoid receptor (GR), and thyroid hormone receptors (TR) as they are expressed at a sufficient level [42,44]. Nonmammalian vertebrates have nucleated red blood cells (RBCs) which in addition to a nucleus contain all cellular organelles differed from mammalian RBC [45]. In the American alligator, these cells have not only genomic DNA, but both white blood cells (WBCs) and RBCs express mRNA for various nuclear receptors at detectable and quantifiable levels by polymerase chain reaction (PCR) (Kohno and Guillette, unpublished data). Therefore, both WBCs and RBCs could be excellent indicators for transcriptome analyses in EDC studies as well as serve as a source of genomic DNA.
9.2.3 In Vitro Transactivation Assays
The in vitro transactivation assay (reporter gene assay) is ideal for screening the potential effects of EDCs on NHR activity. Not only does this assay detect binding to the NHR (i.e., the ability of the chemical to serve as a ligand), but it also can be used to determine if the chemical can function as an antagonist. In addition, the potency of the chemical(s) on receptor function can be assessed, based on its ability to alter transcriptional regulation. A review with significantly more details concerning in vitro methods can be found in the book Nuclear Receptors [46]. Our results to date have confirmed that a number of EDCs do have the potential to bind to various nuclear receptors and activate transcription (Figure 9.5), and they do so at the same concentrations found in circulation in organisms naturally exposed to these compounds [47,48]. Further, by using in vitro transactivation data, doses of EDCs for in vivo studies, such as in ovo embryo exposure experiments, can be determined. These data could also help to elucidate the mechanisms of experimental results from in vivo studies.
9.3 INDUCTION OF SEX REVERSAL IN OVO
In the first study showing that an exposure to an EDC can result in sex reversal in a reptile, Bergeron et al. [49] demonstrated that in ovo exposure to environmental contaminants, such as hydroxylated polychlorinated biphenyls (PCBs), produced females at male incubation temperatures in red-eared slider turtles. Since that study, others have reported that various persistent organochlorine pesticides or their metabolites alter sex determination in reptilian embryos. In the red-eared slider turtle, trans-nonachlor, cis-nonachlor, chlordane, or Aroclor 1242 induce sex reversal at ecologically relevant concentrations [50]. Likewise, trans-nonachlor, dicofol, and 2,3,7,8-Tetrachlorodibenzodioxin (TCDD) induce sex reversal in embryos of the American alligator [51,52]. In caiman, gonadal development was feminized following exposure to the estrogenic compound bisphenol-A (BPA) and the herbicide atrazine [53,54]. In ovo exposure to BPA and atrazine induced ovarian anatomical alterations at 1.4 ppm and 0.2 ppm, respectively, based on egg mass, whereas high-dose BPA (140 ppm) exposure induced sex reversal. The estrogenic nature of the active compound in the pesticide DDT (dichlorodiphenyltrichloroethane), o,p′-DDT, was reported in 1950 [55]. Likewise, metabolites of DDT such as DDT (dichlorodiphenyldichloroethane) or DDE (dichlorodiphenyldichloroethylene) had been known to have endocrine activity for decades, such as the antisteroidogenic actions of o,p′-DDD dichlorodiphenyldichloroethane on the adrenal gland of some species [56]. It is the species-selective nature of this antisteroidogenic action of o,p′-DDD [57] that stimulated us to begin examining possible differences in the response of various species to the endocrine disruptive action of DDT and its metabolites. Three metabolites of DDT, p,p′-DDD, o,p′-DDD, and p,p′-DDE, have been tested on various reptiles with TSD (Table 9.2). These are not matched studies with the same dose ranges, but eggs were treated using the same procedure; that is, applying an exogenous agent onto the eggshell immediately prior to the period of sex determination (see description below). When comparing the relatve estrogenicity of EDCs and estradiol-17β (E2), sex determination of crocodilians appears to be more sensitive to perturbation by EDCs than the models of mammals. Indeed, estrogenicity of BPA (caiman) and p,p′-DDE (alligator) was 1/100 and 1/1,000 that of E2, respectively, whereas BPA exhibited 1/4,000 to 1/10,000 estrogenicity of E2 in mammalian models [18,54]. Therefore, the crocodilian in ovo system is a sensitive model to detect the endocrine activity of EDCs but is not a good screening system for many reasons as compared to in vitro systems in terms of killing animals.
In the red-eared slider turtle, p,p′-DDD was not estrogenic at a relatively low dose, whereas in the American alligator it was estrogenic at similar doses based on the egg mass. In the American alligator, p,p′-DDE displays mixed results, as this compound induces sex reversal in embryos at high doses (1–10 mg/kg), can synergize with its isoform o,p′-DDE to produce 100 percent sex reversal and yet can act in an anti-estrogenic fashion when combined with ethynylestradiol in one study [58]. In another study, p,p′-DDE had weak potency in inducing sex reversal at an MPT (33.5°C), whereas at an intermediate temperature (32°C), it significantly altered the sex ratio in the American alligator [18]. We have shown previously that o,p′-DDE and p,p′-DDE exhibit an affinity for the alligator estrogen receptor purified from the uterus and can displace estradiol-17β, suggesting these isoforms have the potential for estrogenic and anti-estrogenic activity [43]. Recent data suggests that both forms can interact with both ESR1 and ESR2 from the alligator in an in vitro transfection assay but p,p′-DDE is very weak as an agonist (Katsu et al., unpublished data). Thus, species-specific differences can be accessed with the GAL4 transactivation system and compared to receptor-based testing of the same compounds.
It is also important to note that corticosterone exposure in ovo at an intermediate incubation temperature can alter the sex ratio in Australian lizards [59]. This suggests that sex alteration induced by in ovo exposure to endocrine-active compounds could involve various pathways and be a model for examining EDC action at the organismal level, especially when an intermediate temperature or an MPT is used. Further, the eggs incubated at intermediate temperature could be very sensitive to endocrine disruption.
It should also be noted that in vitro culture experiments have revealed that the bipotential gonad (without the adrenal gland or mesonephros) was independently sensitive to incubation temperature and differentiated into an ovary or testis-like structure. However, cell migration from the mesonephros was critical for functional testicular formation [26,60,61]. Gonadal exposure to estradiol in vitro at an MPT induced a reduction in the expression of SOX9, a gene that is characteristic of testicular development, in red-eared slider turtles [62]. These data indicate a direct sensitivity of the gonad to estrogenic compounds and, thus, potential sensitivity to estrogenic EDCs.
9.3.1 Stage Prediction
The timing of dosing the embryo with the chemical(s) of interest is critical for in ovo or in utero
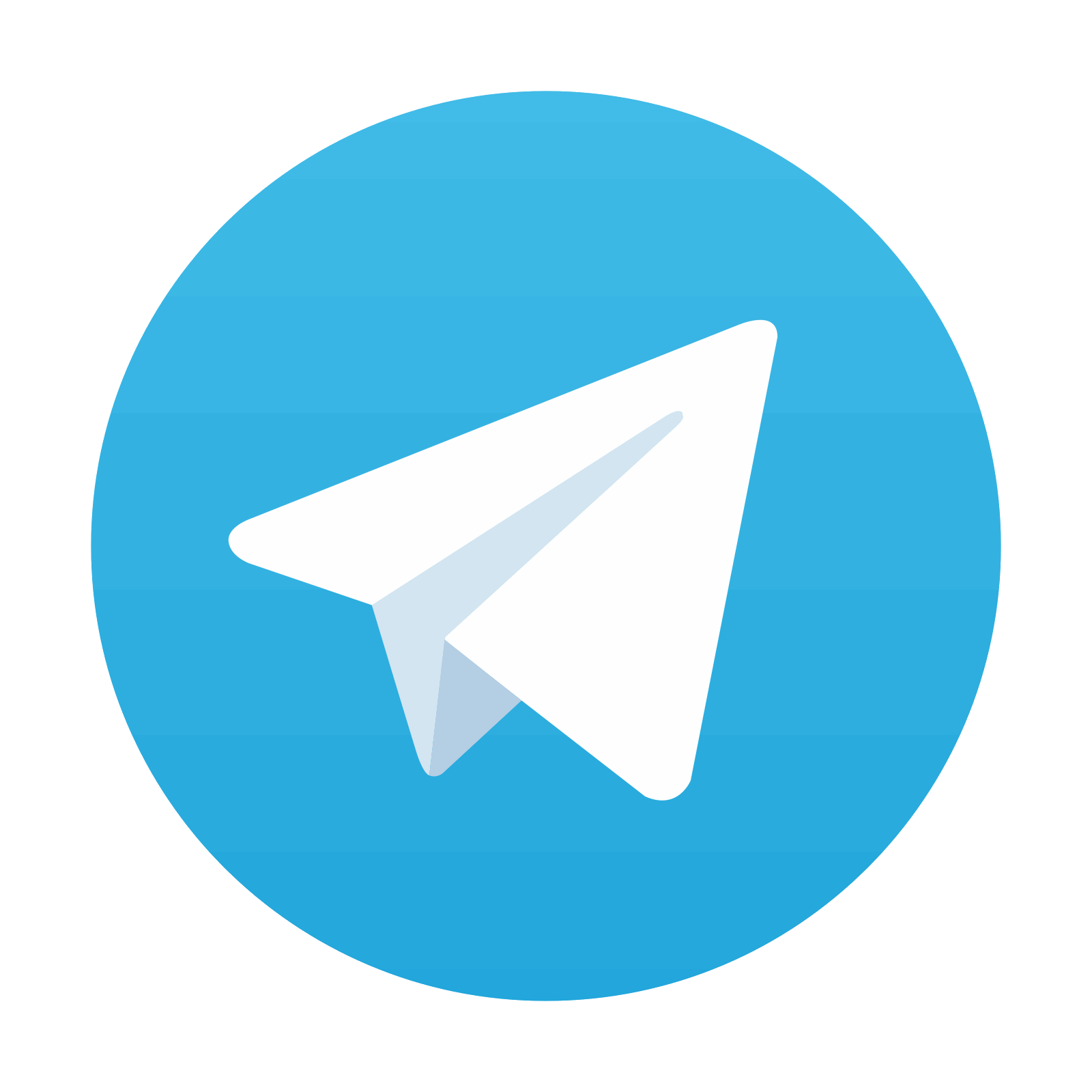
Stay updated, free articles. Join our Telegram channel

Full access? Get Clinical Tree
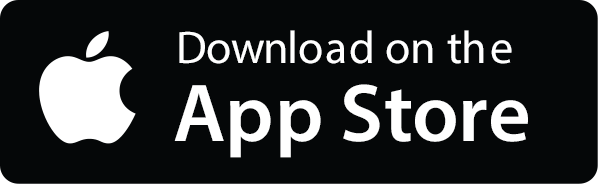
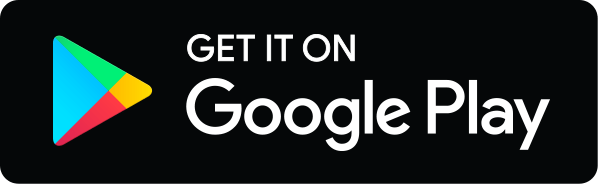