2St John’s Research Institute, Bangalore, India and ETH Zurich, Zurich, Switzerland
- Acute under-nutrition is suspected when an involuntary weight loss of greater than 10% of the body weight occurs over a 3–6-month period.
- Chronic under-nutrition is similar to chronic energy deficiency; this is characterised by a low body mass index (BMI) in weight-stable individuals.
- Under-nutrition that is uncomplicated by disease is associated with several nutrient-saving and homeostatic responses.
- When disease and under-nutrition coexist, the adaptive nutrient-saving responses are generally reversed, such that the depletion of body nutrient stores and tissue is accelerated.
- Nutritional screening is a rapid generic procedure, often undertaken by nonspecialist health professionals to identify individuals at risk of under-nutrition. Nutritional assessment is a more -depth evaluation of nutritional status, which is normally undertaken by a specialist in nutrition, such as a dietician, and is specific to the disease and the patient.
- The assessment of under-nutrition can be made in the clinic using simple clinical techniques. More complex techniques for measuring depletion and body composition or function are also available.
- Nutritional support in under-nutrition should be tailored to the clinical situation; in any case, a modest and balanced diet should be instituted as soon as possible. Monitoring the nutritional support given is essential for a successful outcome.
- There are dangers associated with aggressive and unbalanced diet administration.
5.1 Introduction
‘Under-nutrition’ is often used as a generic term to describe a variety of nutrient deficiencies. The common way to think of under-nutrition is in terms of body weight; this has often led to the terms ‘underweight’ and ‘under-nourished’ being used interchangeably. Under-nutrition is caused by a less-than-adequate intake of nutrients, most of which are related to the energy intake. In adults, this has led to the term ‘energy deficiency’, with a further subclassification into acute – which is sudden, and associated with a declining body weight – and chronic – which occurs over a long period of time, such that the body weight over the preceding few months may be low, but stable.
The definition of under-nutrition in a clinical setting needs to highlight the differences between cachexia, sarcopenia, and under- or malnutrition. There is much debate regarding the definitions of these conditions, and it may be reasonable to think that cachexia and sarcopenia are part of the syndromes of under-nutrition. Cachexia is a catabolic condition caused by disease-related inflammatory activity and negative nutrient balance due to anorexia and/or a decreased absorption of nutrients. In that sense, although this is a catabolic condition, inflammation is the key feature, and muscle or the fat-free mass (FFM), as well as fat mass (FM), decreases in size. The diagnosis of cachexia rests principally on a loss of body weight. In sarcopenia, however, which is now recognised as a multifactorial geriatric syndrome, there is primarily a loss of muscle tissue, as well as decline in muscle strength as a result of ageing and physical inactivity, along with the general wear and tear of the normal life course; therefore, the tissue loss occurs primarily in the FFM. The diagnosis of sarcopenia is thus dependent on an assessment of muscle mass as well as of muscle function. It may not need to be accompanied by a negative nutrient balance, although this can occur when sarcopenia happens in old age. While under-nutrition may be regarded as the inadequate consumption and absorption of nutrients, along with a possible increased loss of these nutrients from the body, cachexia is a multifactorial syndrome characterised by severe body-weight, fat, and muscle loss and increased protein catabolism due to underlying disease. It is rare to see pure under-nutrition in adult patients, and most often a combination of cachexia and under-nutrition occurs.
There are several factors that predispose to the occurrence of under-nutrition within a care setting; these include a very young or advanced age, apathy and depression leading to a decreased intake, and the simple inability to eat food. Equally, carers may fail to recognise the need for nutrition in a timely manner.
The consequences of under-nutrition can be phy sical, psychological, or behavioural. Examples of these are shown in Table 5.1. On a physical level, a loss of muscle and FM, reduced respiratory muscle and cardiac function, and atrophy of visceral organs occurs. At a psychological level, under-nutrition can be associated with fatigue and apathy, which in turn impact on food intake and can worsen the nutritional status. On a behavioural level, under-nutrition is also associated with an increased length of hospital stay, and such patients are more prone to experiencing complications.
Table 5.1 Some of the functional consequences of under-nutrition.
Physical | Muscle strength and fatigueHypothermiaReduced respiratory muscle function and reduced cough pressure, predisposing to chest infectionsImmobility, predisposing to venous thrombosis and embolismImpaired immune functionReduced wound-healingReduced final height in women, leading to small pelvic size and small-birth-weight infants |
Psychological and behavioural | DepressionAnxietyReduced will to recoverSelf-neglectPoor bonding with mother and childLoss of libido |
It has now been proposed that an aetiology-based terminology be used for the diagnosis of under-nutrition in adults in clinical settings, which gives recognition to the interacting effect of inflammation on nutritional status. Therefore, when there is chronic starvation without inflammation, the term ‘starvation-related malnutrition’ is used. An example of this condition is anorexia nervosa. When chronic inflammation is present, the term ‘chronic disease-related malnutrition’ is used. Examples of this condition include organ failure and sarcopenic obesity. When acute inflammation is present, the term ‘acute disease-related malnutrition’ is used. Examples include major infections, burns, and trauma.
5.2 Pathophysiology of under-nutrition
Body composition
Body-weight loss on starvation is initially rapid (up to 5 kg over a few days), due to the emptying of glycogen reserves, the utilisation of body protein, and the accompanying water loss. The later loss of body tissue that accompanies negative energy balance primarily comprises fat, although varying amounts of lean tissue are lost as well. This is demonstrated in a classic study on the effect of long-term semi starvation on healthy men by Keys et al. (1950). During their 6-month semi-starvation study, the lean-tissue loss observed in the subjects represented about half of the total weight loss. Although physical activity would be expected to maintain muscle mass in such a situation, it is clear that lean tissue is also catabolised to provide energy in the face of large energy deficits. The composition of weight loss is dependent on different conditions under which the negative energy balance is imposed: in healthy, normal young men, the weight loss is composed of fat and FFM, while in obese individuals, it may be primarily FM.
Energy metabolism
Accompanying the changes in body composition during acute negative energy balance are adaptive changes in energy expenditure. These changes can occur in one or more of: the basal metabolic rate (BMR), thermogenesis (the production of heat by the body in response to various stimuli), and physical activity (Figure 5.1).
Reductions in the BMR are partly mediated through weight loss itself, in which metabolically demanding tissues of the body are reduced in size, and partly through reductions in the metabolic activity of these tissues. In the semi-starvation study referred to before, the subjects’ BMR decreased by about 25% when expressed per kilogram of their FFM (or metabolically active tissue). This decline was most rapid in the first 2 weeks, indicating that the reduced metabolic activity of the fat-free tissue occurred quickly in response to energy deficiency.
The factors underlying the decrease in the activity of the FFM include reduced activity of the sym pathetic nervous system – which is a part of the autonomic nervous system and which partly drives the heat-producing activity of the FFM – as well as altered peripheral thyroid metabolism, lowered insulin secretion, and substrate utilisation designed to maintain glucose production and maximise fat usage. Leptin is a hormone that plays a key role in regulating energy intake and energy expenditure. It is expressed in adipose tissue (the ob gene product) and, once secreted into the circulation, acts at the hypothalamic level to inhibit appetite. It therefore forms a link bet ween the FM, energy intake, and expenditure, acting as a lipostatic mechanism through modulation of satiety and the activity of the sympathetic nervous system. Leptin levels are low in anorexia nervosa, suggesting that leptin may be important in under-nourished, low-FM states as a potential modulator of the energy-sparing response to energy deficiency. An additional modulator of the response to underfeeding is the genetic makeup of the individual. In an interesting study on monozygotic twin pairs, body weight and compositional change were studied after 93 days of negative energy balance induced by increased exercise with constant dietary intake. The within-pair variation was much less than the between-pair variation for loss of weight, body fat, visceral fat, and respiratory exchange ratio during exercise (see Figure 5.2 for weight change), showing that subjects with the same genotype were more alike in responses than subjects with different genotypes.
On the other hand, there are also findings of an increased BMR per kilogram body weight in a number of clinical studies arising from energy deficiency. These conflicting findings may be due to factors including whether or not the patient was in a state of recovery, and methodological errors during the conduct of BMR measurement. The increased BMR could also be due to the stress imposed by illness in clinical situations (see later in this chapter).
Even though thermogenesis only accounts for a small part of overall energy expenditure, changes which occur as a result of total or semi-starvation can add to the decreased energy expenditure observed in this condition. Compared to the dramatic fall in BMR during acute energy deficiency, the influence of thermogenesis is much less pronounced and less consistent, but still measureable. For example, in elderly, under-nourished women admitted into hospital with fractured femurs, the thermogenic response to a cold stimulus was diminished.
Figure 5.2 Changes in body weight with negative energy balance in seven pairs of twins. Twin A on the ordinate, twin B on the abscissa. Reprinted from Bouchard et al. (1994), with permission of Nature Publishing Group.
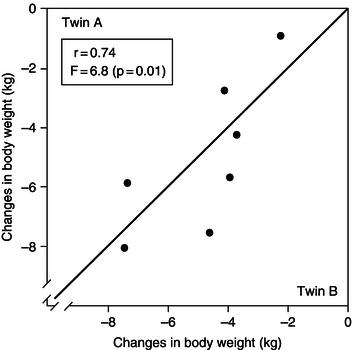
Figure 5.3 Interaction between factors leading to lowered energy expenditure as a result of negative energy balance. BMR, basal metabolic rate.
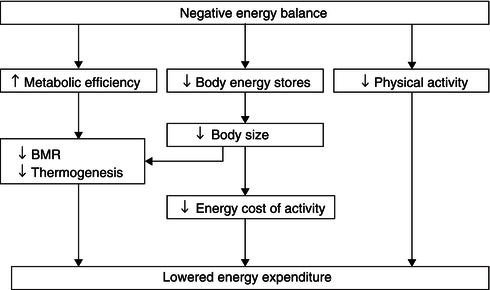
Even with otherwise healthy individuals, semi-starvation or starvation leads to a reduction in physical activity. From the behavioural viewpoint, the imposition of an acute energy deficiency also causes apathy, with a marked reduction in spontaneous activity. A decrease in food intake results in a change in selection of discretionary activity patterns, such that lower-activity discretionary patterns are selected. These behavioural changes are obviously designed to maximise the chances of survival under conditions of acute energy deficiency.
Overall, therefore, it appears that energy deficiency is associated with body-weight loss, along with changes in body composition and a reduction in BMR and physical activity. Figure 5.3 shows how these factors interact with each other to attain lower energy expenditure when an acute negative energy balance exists.
If the lowered energy expenditure is adequate to compensate for the decreased energy intake that caused the negative energy balance, and allows for a new neutral energy balance to be achieved, the person can survive, albeit at a lower plane of nutrition. This leads to a chronic energy deficiency (CED), discussed in Section 5.4. If a homeostatic response is not possible, due to a severe energy deficit, body energy and muscle stores continue to be lost until a lethal weight loss occurs, usually when body weight or FFM has fallen to about half its original value.
Protein metabolism
When nitrogen (protein) intake is reduced, the nitrogen output also decreases, although this can take some days to occur. In subjects who are put on low-protein diets, some 7–14 days may be required for the N output to stabilise at a new lower level (Figure 5.4). This is important when assessing N balance, particularly over a short time period, since the previous N intake influences the amount of N output.
This adaptation spares body protein and preserves essential functions in the body; since the protein loss never reaches zero, there will be losses of protein from the body, primarily from the skeletal muscle mass. In more chronic under-nutrition (but not starvation), the relatively greater loss of muscle mass leads to an increase in the viscera-to-skeletal muscle mass ratio of the FFM. An important point is that when the protein intake is acutely reduced, there is a transient loss of N over a period of days. This has been attributed to a ‘labile’ pool of body protein, which contracts when protein intake is reduced (and, conversely, expands when protein intake is increased), but the identity of this pool of body protein has not been established in humans.
The adaptive reduction in N output during protein deprivation can be mediated though a decrease in amino acid oxidation, reduced formation of ammonia in the kidney, or reduced formation of glucose (gluconeogenesis) in the liver. Glucose is primarily needed by organs such as the brain. This need is met in conditions of starvation by proteolysis. Although ketoacids, which derive from fat, become important fuels for the brain as starvation progresses, they do not eliminate brain glucose requirements. Increasing the energy intake will improve N balance, just as increasing the N intake would, and when both N and energy are provided at their minimum requirement level, the N balance is expected to be zero. Thus, energy can be said to have a protein-sparing effect in the submaintenance range of protein intakes. The effect of N and energy intake on N balance is interdependent and complex. The level of N intake determines the change in N balance that can be achieved with an increase in energy intake. On the other hand, the effect of energy intake on N balance depends on the intake range within which alterations in energy intake occur, and a value of 7.5 mg N/kcal has been reported for submaintenance energy intakes of up to about 15 kcal/kg (Figure 5.5). About one-third of the variation in N balance can be attributed to variations in energy intake relative to the requirement, and 1–1.5 mg N/kg body weight is gained (or spared) per extra 1 kcal/kg body weight of energy intake at maintenance levels of intake. This has led to the suggestion that in under-nutrition, the high slope of the N balance-to-energy intake relationship is due to replenishment or maintenance of the body cell mass, while at high energy intakes, the N retained may be needed to support the extra energy retained as fat.
Figure 5.4 Rate of change in nitrogen (N) excretion after changing from a high to a low protein intake. Data from Quevedo et al. (1994) © The Biochemical Society.
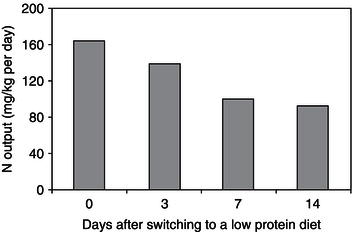
Figure 5.5 Relationship between N balance and energy intake. From Elwyn DH. Nutritional requirements of adult surgical patients. Crit Care Med 1980; 8: 9–20. Copyright © Wolters Kluwer Health.
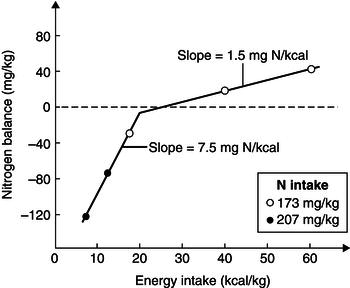
The type of energy source (carbohydrate or fat) is also important in determining the influence of energy on N balance. In the submaintenance range of protein intakes, it has been shown that carbohydrate is more effective than fat, perhaps because of the greater insulinogenic influence of carbohydrate. At maintenance intakes, as well as in the clinical setting, both carbohydrate and fat have been found to be equally effective, although some studies suggest that a fat/glucose regimen is better than a glucose-alone regimen. The message from these observations is that energy spares protein, and 100–150 g of glucose per day has been described to be effective in reducing urea N excretion by about half.
Measurements of the rate of protein turnover show that it changes little, if at all, with moderate reductions in protein intake, although with severe protein deficiency there is a reduction in whole-body protein turnover. Whole-body protein synthesis does not appear to change with feeding, and changes in proteolysis appear to mediate most of the acute regulatory changes associated with feeding and fasting. While whole-body protein synthesis rates are relatively unc ha nged, there may be differences in tissue-specific protein synthesis rates. In rats given a low protein intake, rates of protein synthesis are reduced in the muscle, but increased in the liver. The rate of synthesis of albumin is also decreased with a low protein intake; however, this is followed by a decrease in the rate of catabolism as well after a few days. In addition, there is a transfer of albumin from the extravascular to the intravascular pool, preserving serum albumin concentrations. Similarly, a relatively low protein intake does not change the concentrations of other liver secretory proteins such as retinol-binding protein and transferrin.
There are other nutrients that are important in the maintenance of the FFM. Intracellular constituents such as potassium and phosphorus are important in maintaining a positive N balance when a diet adequate in protein and energy is given to malnourished patients. Diets lacking in potassium or phosphorus lead to negative N balances even when all other nutrients are adequate, while a lack of sodium reduces the extent of the observed positive N balance in malnourished patients receiving an otherwise adequate diet.
Hormonal mediators
During starvation, the maintenance of blood glucose levels is paramount for the organism. Thus, starvation is associated with a decrease in the hypo gly caemic hormone insulin and an increase in counter-regulatory hormones that aim to increase glucose, including glucagon, which promotes hepatic glucose production. The lowered insulin concentrations also allow for a reduced peripheral utilisation of glucose and an increased mobilisation of fat. Thus, fatty acids become an important oxidative fuel for peripheral tissues during starvation. Thyroid hormone levels also show changes, in that the active form of the hormone (T3) is reduced, while the inactive form (reverse T3) is increased in the serum. The activity of the sympathetic nervous system is also reduced, as are serum concentrations of catecholamines. The implication of a decrease in these hormones in nutritional terms is that energy expenditure decreases, thus reducing the magnitude of the negative energy balance.
Immune function in under-nutrition
The immune system depends on cellular and secre tory activities, which require nutrients in much the same way as other processes in the body. Severe protein-calorie malnutrition causes a decline in immune function, in terms of lymphocyte number, cell-mediated immunity, antibodies, and phagocytosis, and therefore an increase in infective morbidity. The levels of other immune mediators, including most complement factors (complement is an important mediator of inflammation activated by antibodies) are also decreased in protein–energy malnutrition. The decreased immunocompetence seen with under-nutrition is a type of acquired immunodeficiency, and several adverse clinical effects, such as opportunistic infections, marked depression of the delayed cutaneous hypersensitivity response, and increased frequency of septicaemia, occur. A low protein intake on its own also results in a decline in both cell- mediated and antibody-based immunity.
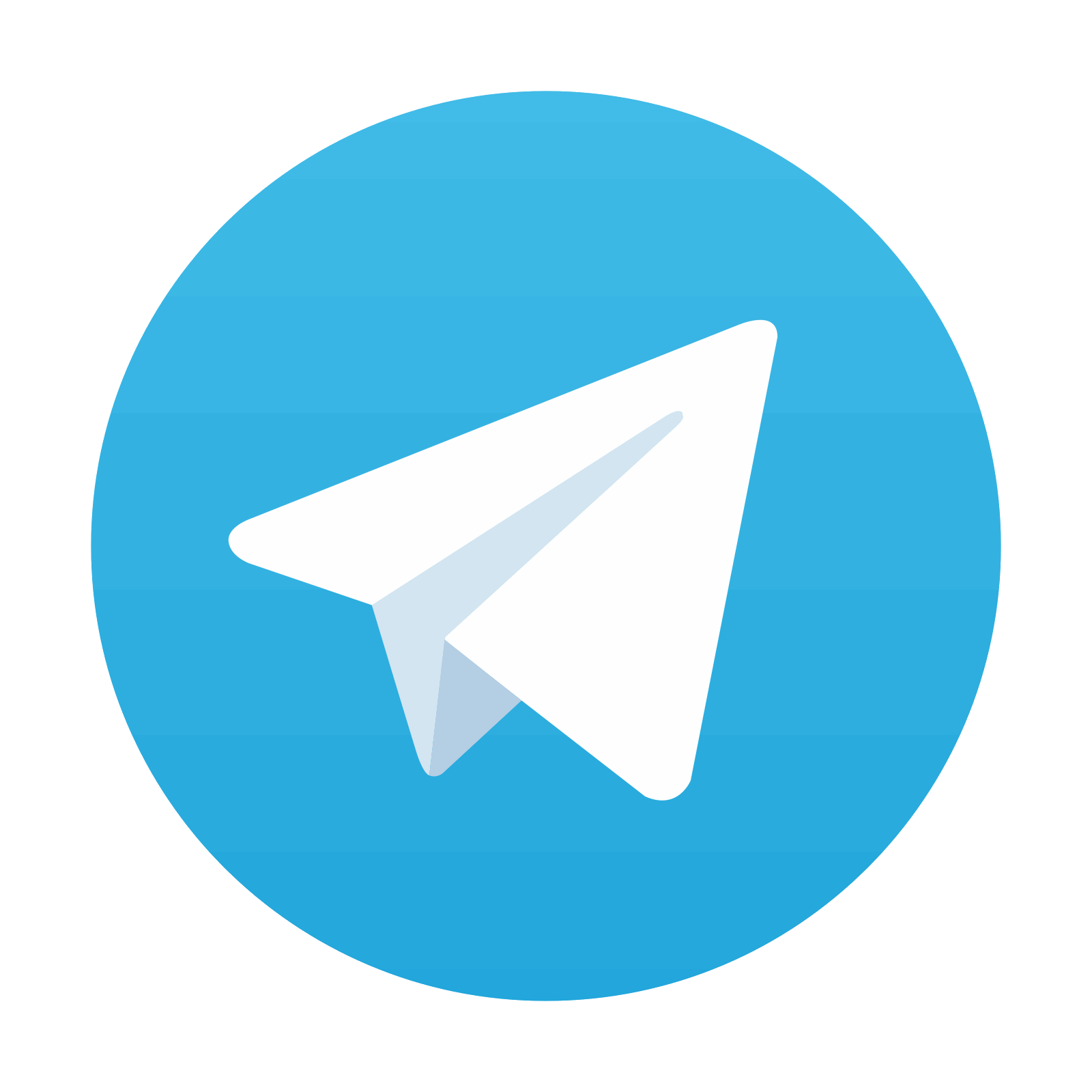
Stay updated, free articles. Join our Telegram channel

Full access? Get Clinical Tree
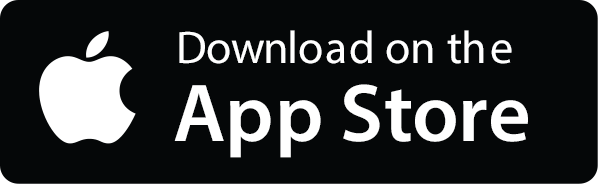
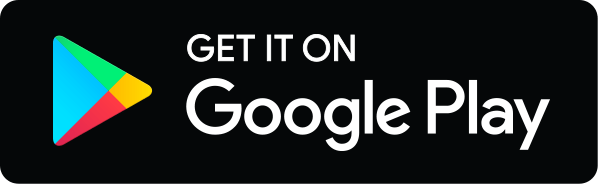