Fig. 12.1
The design and general method of conduct of investigations of lipoprotein and apoprotein metabolism which employ isotopes. Studies investigating lipoprotein metabolism in plasma frequently employ isotopic labeling of the apoprotein moiety of the particles. Both radioisotopes and nonradioactive, stable nuclides (e.g., 2H, 15N) are frequently used to label lipoprotein protein. Studies of lipoprotein metabolism fall into one of two general classes: studies in which lipoproteins are initially isolated and the apoprotein radiolabeled before the lipoprotein is injected back into the study subject and studies in which isotopes are infused at a constant rate until they are absorbed into the liver and subsequently incorporated into lipoprotein proteins. In the latter type of study, the lipoprotein particles which are secreted de novo by the liver contain the stable nuclides which permit these lipoproteins to be distinguished from those already present in the circulation. Both approaches require frequent sampling of plasma from the study subject. Lipoprotein metabolism may be studied using whole plasma but more often individual Fig. 12.1 (continued) lipoprotein classes are separated and purified using ultracentrifugation or other methodology. Lipoprotein metabolism or “turnover” is quantitated as the appearance/disappearance of radioactivity in plasma and isolated lipoprotein fractions when radioactive tracers have been employed. When stable nuclides are infused, plasma and lipoprotein samples must be extensively processed to enable detection and quantitation of the stable isotopes using gas chromatography (GC) or liquid chromatography (LC) in combination with mass spectroscopy (MS). Both approaches ultimately require sophisticated, computer-aided, mathematical modeling to convert the patterns of isotope appearance/disappearance in plasma/lipoproteins into intuitive metabolic pathways
The methods described above rely on radioactively labeled lipoproteins or infusions of radioactivity. This approach, however, is considered by some to be non-ideal for several reasons:
(a)
Lipoproteins and apolipoproteins potentially can be modified, such as by oxidation or aggregation, during isolation and radioiodination which may influence their metabolic behavior in vivo.
(b)
A steady-state condition where production and clearance rates are taken to be constant is difficult or impossible to document and, therefore, must be assumed, an assumption that may not always be physiologically accurate.
(c)
Studies cannot be undertaken in young children or in pregnant women, nor can multiple studies be undertaken in the same volunteer due to exposure to potentially hazardous levels of radioactivity.
Stable Isotope-Based Studies
A new experimental approach to investigations of apoB metabolism has been developed which relies on modern instrumentation and which eliminates complications associated with the administration of radioactivity to humans. This method uses intravenous infusion of stable isotope-labeled amino acids after a priming dose that achieves and maintains an isotopic steady state [15, 16] that is necessary for the successful conduct of this type of study. The intricacies and advantages or disadvantages associated with each of these three types of lipoprotein, or lipoprotein precursor, tracer infusion studies have been discussed at length in other excellent articles [17].
Dual Radiolabel Studies
As discussed in the chapter on lipoprotein glycation, the incubation of human LDL with glucose results in a nonenzymatic formation of a Schiff base between the monosaccharide and lysyl residues in apoB. As a greater percentage of the lysyl residues of apoB in LDL become modified by glycation, the fractional catabolic rate of the glycated LDL decreases in in vivo studies [18]. The rates of catabolism of glycated LDL by cultured human skin fibroblasts are also decreased suggesting that glycated LDL is catabolized primarily via a receptor-independent process. Thus, radiolabeled LDL which has been extensively glycated is frequently injected concomitantly with native LDL radiolabeled using another isotope, and the rates of LDL metabolism via receptor-independent and receptor-dependent pathways, respectively, estimated from the ratio of the fractional catabolic rates determined using each uniquely radiolabeled lipoprotein preparation [18].
HDL-Related Turnover Studies
Studies investigating the synthesis and catabolism of HDL apolipoproteins, primarily apoA-I and apoA-II, are conducted in a manner similar to those described above for apoB. Lipoproteins containing radiolabeled apolipoproteins(s) are injected into each study subject, and the lipoprotein fraction of interest is isolated from serially collected blood samples to monitor radioactivity decay patterns. Investigating the metabolism of HDL apolipoproteins using this type of study technique is inherently more difficult because it has been documented that protein in HDL is freely exchangeable between HDL particles and lipoproteins in other density classes [19, 20].
It is these types of kinetic studies that have led to the knowledge regarding changes in VLDL, LDL, and HDL production and clearance in people with vs. without diabetes as is described in the chapter on lipoprotein metabolism in diabetes.
Lipoprotein Metabolism in Cultured Cells
Investigations of lipoprotein metabolism in cultured cells generally focus on three distinct stages of cell-lipoprotein interaction:
(a)
Lipoprotein binding to cell surface lipoprotein receptors
(b)
Internalization of the lipoprotein from the cell surface in preparation for subsequent metabolism by the cell (if binding of the lipoprotein to the receptor results in receptor internalization)
(c)
Degradation of the internalized lipoprotein in the cell lysosomal compartment
Lipoprotein Binding to Cells
The Nobel Prize in Physiology or Medicine in 1985 was awarded to Joseph L. Goldstein and Michael S. Brown. Their Nobel prize-winning research not only elucidated the molecular mechanism whereby exogenous cholesterol in VLDL and LDL downregulates cellular 3-hydroxy-3-methylglutaryl coenzyme A reductase enzymatic activity (HMG-CoA reductase), the rate-limiting step of cellular endogenous cholesterol biosynthesis, but it also developed many of the techniques necessary for the study of lipoprotein metabolism in cultured cells. Many of these research techniques remain in use today. Their earliest studies demonstrated that LDL radiolabeled with tracer 125I-iodine can be taken up by cultured fibroblasts from normal subjects in a temperature dependent process that is highly specific, reaches equilibrium with time, and is saturable at low levels of LDL [21].
These studies clearly demonstrated for the first time that the amount of 125I-LDL bound to the cells was reduced by the addition of a 50-fold excess of non-radiolabeled, native LDL, which suggested that the radiolabeled LDL and native LDL were competing for a limited number of specific binding sites. Furthermore, these studies introduced the concept of “specific” lipoprotein binding to cells, which would be reported from this juncture as the difference between the lipoprotein radioactivity bound to cells in the absence and presence of excess native LDL. The development of techniques to measure both “specific” lipoprotein binding as well as “nonspecific” binding (defined as the level of 125I-LDL radioactivity bound in the presence of a 50-fold excess of native LDL) enabled these investigators to conduct Scatchard analyses of the LDL binding to fibroblast receptors. These study results suggested the presence of a specific LDL binding site of high affinity which could be saturated at relatively low LDL concentrations (20 μg/ml). Most importantly, these studies demonstrated that specific LDL binding appeared to be required in the process by which LDL normally suppressed HMG-CoA reductase activity and further, the binding of LDL to fibroblasts from patients homozygous for familial hypercholesterolemia was defective and appeared to explain the previously reported failure of LDL to suppress the synthesis of this enzyme in fibroblasts isolated from these patients [21].
Lipoprotein Degradation by Cells
While studying the binding of 125I-LDL to normal fibroblasts, Brown and Goldstein noted that the 125I-LDL bound to cells was ultimately degraded to form a product that was dialyzable and which could no longer be precipitated with trichloracetic acid (TCA) [22]. In subsequent studies [23] they refined this technique to include an additional step in which the acid-soluble material appearing in the culture media harvested after cells were incubated with 125I-LDL at 37 °C for several hours was oxidized with hydrogen peroxide and then extracted with chloroform. This step eliminated the artifactual contamination of the small amount of radioactive free iodide that persisted in the 125I-LDL preparation despite extensive dialysis. Control studies conducted by incubating the 125I-LDL preparation at 37 °C in culture media without cells revealed that the formation of this iodide-free, acid-soluble degradative product of LDL was absolutely dependent on the presence of cells and, furthermore, was linear with time up to at least 30 h. The use of this cell-free, spontaneous degradation control became routine for all subsequent studies of lipoprotein metabolism by cells. Most of the TCA-soluble material secreted into the culture medium was identified to be 125I-tyrosine. Uptake of LDL by both the specific, high-affinity process and also the nonspecific, lower-affinity process results in degradation of the lipoprotein, and the degradation processes appear to be similar [22].
Lipoprotein Accumulation by Cells
To demonstrate the conversion of bound 125I-LDL to acid-soluble material, cultured human fibroblast cells were first preincubated at 4 °C with 125I-LDL. These conditions permit the LDL to bind to cell surface receptors as demonstrated by continued LDL susceptibility to protease degradation [21, 22] even after 4 h incubation, but without appearance of 125I-acid-soluble material in the media. Cells which had been preincubated at 4 °C with 125I-LDL were then transferred to medium without 125I-LDL and were additionally incubated at either 4° or 37 °C. At the beginning of this second incubation, all radioactivity bound to the cells was precipitable with TCA. When the cells were incubated at 37 °C, nearly all this bound radioactivity was released into the cell culture medium within 3 h, and approximately two thirds had been converted to acid-soluble material. In contrast, when the cells were incubated at 4 °C, only about one third of the 125I bound to the cells was released, and all the radioactivity was precipitable by TCA. The difference in the amounts of radioactivity localized to cells incubated at 37 °C (bound and internalized, but not degraded LDL) compared to that found in cells incubated at 4° (bound LDL only) is considered to be accumulated LDL. These types of studies are not conducted as often as those measuring lipoprotein binding or degradation.
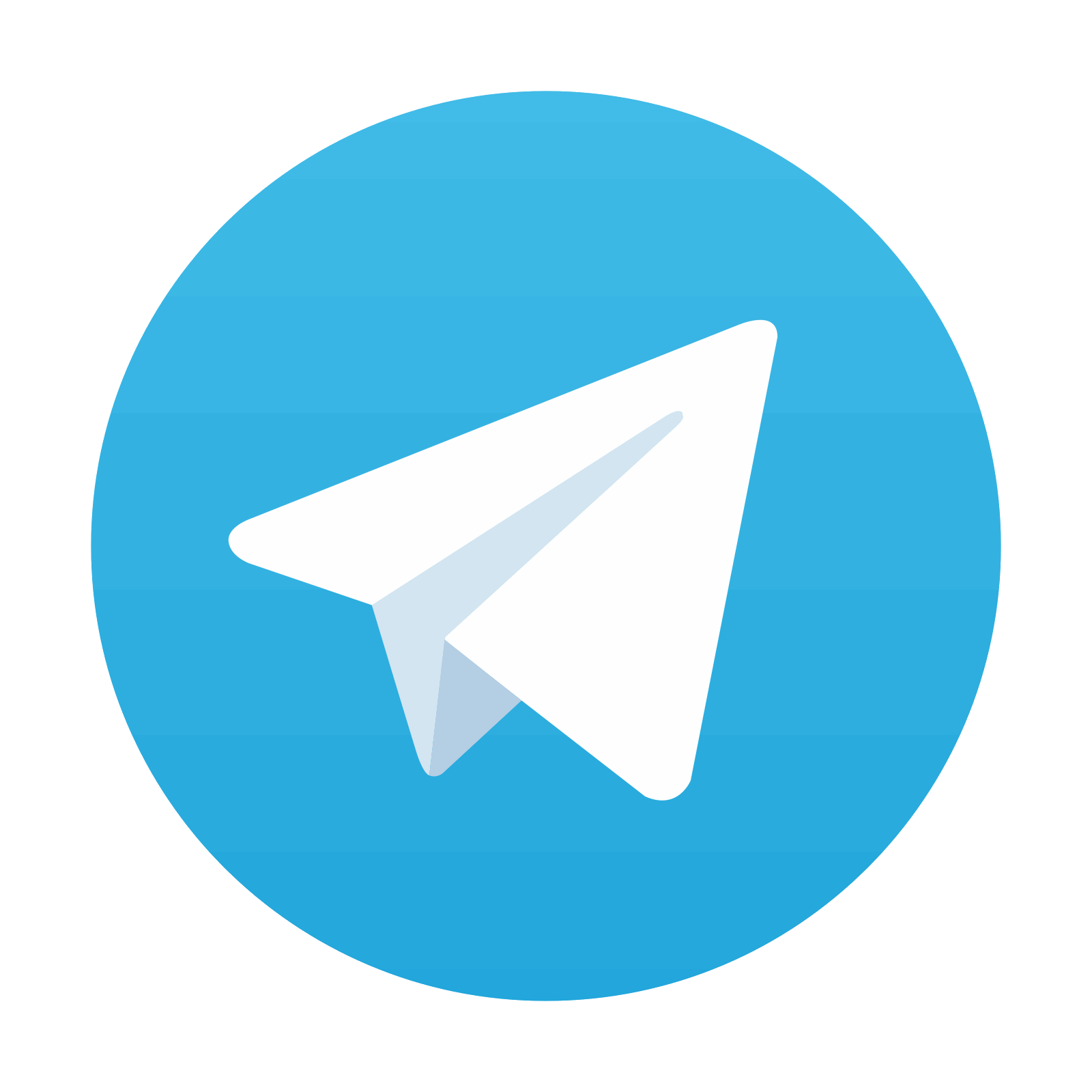
Stay updated, free articles. Join our Telegram channel

Full access? Get Clinical Tree
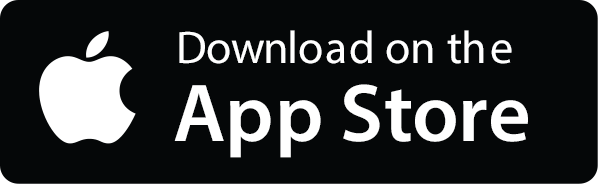
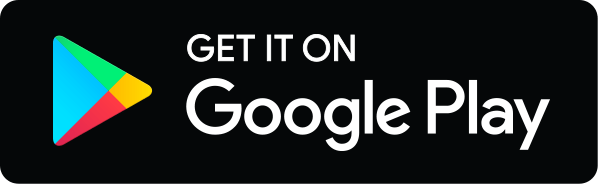