Thyroid Disorders During Pregnancy and Postpartum
John H. Lazarus
Jorge H. Mestman
Thyroid disorders are common. The prevalence of hyperthyroidism is around 5 per 1000 and hypothyroidism about 3 per 1000 in women. As the conditions are generally much more common in the female it is to be expected that they will appear during pregnancy. Developments in our understanding of thyroid physiology (1,2) and immunology (3) in pregnancy as well as improvements in thyroid function testing (4) have highlighted the importance of recognizing and providing appropriate therapy to women with gestational thyroid disorders (5). This chapter will discuss these aspects following an account of the effect of pregnancy on thyroid economy in addition to indicating changes in the immune state during gestation which may influence thyroid status and improve our understanding of autoimmune thyroid disease and its management in pregnancy. An account of postpartum thyroid disease will also be presented as this may be predicted from pregnancy-associated thyroid abnormalities.
Thyroid Function During Pregnancy
Pregnancy affects thyroid homeostasis. An increased excretion of iodine in the urine accounts for the increase in thyroid volume even in areas of moderate dietary iodine intake (6). Some studies, however, do not show an increase in urinary iodine during pregnancy. In either case the increase in thyroid volume is the result of imbalance between the intake and increased requirements of iodine during pregnancy. Iodine deficiency during pregnancy is associated with maternal goiter and lower than expected maternal thyroxine (T4) and free T4 (FT4) levels. While thyroid size increases in areas of iodine deficiency, it does not do so in those regions that are iodine sufficient; even in moderate iodine-deficient regions, urinary iodine excretion is higher in all trimesters than in non-pregnant women and may be the cause of maternal goiter formation as assessed by ultrasound. The gestational goitrogenesis is preventable by iodine supplementation in areas of severe (24-hour urinary iodine less than 50 μg) and milder iodine deficiency such as Belgium where trials of iodine supplementation have shown maternal thyroid volume reduction. The iodine supply to pregnant and lactating women should be at least 250 μg/day (7). Iodine deficiency in pregnancy is one of the most important causes of childhood mental impairment in the world. A non-randomized study of iodine supplementation given before the 10th week of gestation resulted in an improvement in childhood neurodevelopment (8) and absence of iodine supplementation before 14 weeks gestation led to neurodevelopmental delay in the offspring (9). It is essential to monitor iodine intake even when adequate ambient iodine concentrations have been achieved (10). Median urinary iodine concentration is the best indicator to use in population surveys to assess the iodine nutrition of pregnant women (7). It is desirable for pregnant women to take iodine supplements (150 μg/day) but this public health measure is not universally adhered to at present.
The major thyroid hormone transport protein, thyroxine binding globulin (TBG), increases due to enhanced hepatic synthesis, a reduced degradation rate due to oligosaccharide modification and an estrogen-induced increase in sialylation, which increases the half-life of TBG, leading to increases in total T4 concentrations early in gestation (1). Serum concentration of free thyroid hormones has been reported to be decreased, increased, or unchanged during gestation by different groups depending on the assays used. However, there is general consensus that there is a transient rise in free thyroxine (FT4) in the first trimester due to the relatively high circulating hCG (a weak thyroid stimulator) concentration and a gradual fall of FT4 in the second and third trimester albeit within the normal reference range. Changes in free triiodothyronine (FT3) concentrations are also seen in which they broadly parallel the FT4, again within the normal range. The precise reason for the decline in free thyroid hormones is not clear. In iodine-deficient areas (including marginal iodine deficiency seen in many European countries), the pregnant woman may become significantly hypothyroxinemic with preferential T3 secretion. The thyroidal “stress” is also evidenced by a rise in the median TSH (within the normal range) and serum thyroglobulin.
The profound changes that occur during the first few weeks following conception have consequences for thyroid hormone concentrations and thyroid function assessment. Also, dramatic increases in serum hCG lead to thyroid-stimulating activity, increased thyroid hormone secretion, and decreases in TSH concentrations and seem to be somewhat dependent on ethnicity. In addition, pregnancy-related increases in plasma volume and glomerular filtration rate (by approximately 50%), as well as changes in deiodinase activity in the placenta, can lead to small decreases in serum thyroid hormone concentrations as pregnancy progresses. Moreover, due to the general immunosuppressive state during pregnancy, thyroid autoimmune-related activity is decreased, leading to additional physiologic changes that result in changes in serum thyroid hormone concentrations.
Table 56.1 Physiologic Changes in Pregnancy That Influence Thyroid | ||||||||||||||||||
---|---|---|---|---|---|---|---|---|---|---|---|---|---|---|---|---|---|---|
|
Thus, pregnancy is associated with significant, but reversible changes in thyroid function shown in Table 56.1 (11). The findings associated with the hypermetabolic state of normal pregnancy can overlap with the clinical signs and symptoms of thyroid disease.
Human Chorionic Gonadotropin
The placenta secretes human chorionic gonadotropin (hCG), a glycoprotein hormone sharing a common alpha subunit with TSH but having a unique beta subunit which confers specificity. Evidence derived both from in vitro studies on thyroid
tissue and on eukaryotic cells stably expressing the human TSH receptor (TSHR), suggests that hCG, or a molecular variant, is able to act as a TSH agonist. In addition, the modification of carbohydrate structure attached to hCG may alter the biologic activities of the molecule; for example, asialo-hCG plays a critical role in hyperthyroidism during pregnancy and has increased thyrotropic effects. The structure and thyrotropic activity of hCG preparations is variable. This relates to heterogeneity in several properties including charge, sialic acid content [decreased sialic acid content enhances hCG bioactivity in both gestational trophoblastic neoplasia and gestational thyrotoxicosis (GTN and GT)], interchain nicks at positions β47–48 or β44–45, and, possibly, expression of as many as six β-subunit hCG genes (12). The precise relationship between hCG structure and bioactivity is not known, not only because of the number and complexity of hCG isoforms but also because bioactivity is difficult to measure in a way meaningful to human physiology. An important problem is the poor correlation between bioactivity as measured by in vitro and in vivo systems. Despite these reservations, it is noteworthy that the hCG produced by hydatidiform mole tissue as well as that produced in the first trimester of normal gestation, is reported to have high thyroid stimulating specific activity. Therefore, the development of thyrotoxicosis in GTN and GT may be related not only to the high serum hCG concentrations but also to the nature of the hCG isoforms that are produced in these settings. The sensitivity of the TSHR to hCG has also been demonstrated by a unique case report of familial gestational hyperthyroidism caused by a mutant TSHR (13). In this report, a woman and her mother were described who both had recurrent gestational hyperthyroidism and normal serum hCG. Both were heterozygous for a missense mutation in the extracellular domain of the TSHR which was more sensitive to hCG than the wild-type receptor. Whether hCG has any effect on thyroid antibody expression during pregnancy is unknown.
tissue and on eukaryotic cells stably expressing the human TSH receptor (TSHR), suggests that hCG, or a molecular variant, is able to act as a TSH agonist. In addition, the modification of carbohydrate structure attached to hCG may alter the biologic activities of the molecule; for example, asialo-hCG plays a critical role in hyperthyroidism during pregnancy and has increased thyrotropic effects. The structure and thyrotropic activity of hCG preparations is variable. This relates to heterogeneity in several properties including charge, sialic acid content [decreased sialic acid content enhances hCG bioactivity in both gestational trophoblastic neoplasia and gestational thyrotoxicosis (GTN and GT)], interchain nicks at positions β47–48 or β44–45, and, possibly, expression of as many as six β-subunit hCG genes (12). The precise relationship between hCG structure and bioactivity is not known, not only because of the number and complexity of hCG isoforms but also because bioactivity is difficult to measure in a way meaningful to human physiology. An important problem is the poor correlation between bioactivity as measured by in vitro and in vivo systems. Despite these reservations, it is noteworthy that the hCG produced by hydatidiform mole tissue as well as that produced in the first trimester of normal gestation, is reported to have high thyroid stimulating specific activity. Therefore, the development of thyrotoxicosis in GTN and GT may be related not only to the high serum hCG concentrations but also to the nature of the hCG isoforms that are produced in these settings. The sensitivity of the TSHR to hCG has also been demonstrated by a unique case report of familial gestational hyperthyroidism caused by a mutant TSHR (13). In this report, a woman and her mother were described who both had recurrent gestational hyperthyroidism and normal serum hCG. Both were heterozygous for a missense mutation in the extracellular domain of the TSHR which was more sensitive to hCG than the wild-type receptor. Whether hCG has any effect on thyroid antibody expression during pregnancy is unknown.
The Placenta
During the first trimester the human conceptus is surrounded by the placenta, which acts as an exchange unit for nutrients and waste products. The primary barrier to exchange between mother and fetus is the syncytiotrophoblast layer of the placental chorionic villi which has effective tight junctions and prevents the free diffusion of thyroid hormones across it.
The human placenta in addition to this cellular barrier also regulates the amounts of thyroid hormones passing from the mother to the fetus through its expression of placental thyroid hormone transporters, thyroid hormone binding proteins, iodothyronine deiodinases, sulfotransferases, and sulfatases (14,15). The transport of iodine through the placenta is also important as the organ has shown to actively concentrate the anion (16).
Fetal circulating concentrations of total T3 are at least 10-fold lower than total T4. Unlike adults, the proportion of free unbound T4 is also higher than bound T4 in early gestation. Free T4 levels are determined by the fetal concentrations of the thyroid hormone binding proteins in the circulation and coelomic cavity and the amount of maternal T4 crossing the placenta. The concentration of free T4 in the coelomic fluid in the first trimester is approximately 50% of that found in the maternal circulation and could, therefore, exert biologic effects in fetal tissues.
The human placenta expresses iodothyronine deiodinases type II (D2) (which activates T4 to T3) and type III (D3) (which inactivates T4 and T3). The principal subtype in the placenta is D3, having 200 times the activity of D2. D3 effectively metabolises most of the maternal T4 presented to the placenta: Still a physiologically relevant amount of T4 is transferred to the fetus. Both D2 and D3 activity per gram of placenta decrease with advancing gestation (15).
A range of thyroid hormone transporters including monocarboxylate transporters (MCT) 8 and 10, system-L amnio acid transporters (LAT1 and LAT2) and organic anion transporting polypeptides (OATP) 1A2 and 4A1 have been located at the apical and basolateral membranes of the syncytiotrophoblasts (17). These transporters may facilitate thyroid hormone transfer across the cell barrier from the mother to the fetus (Fig. 56.1).
In addition to the regulation of transplacental thyroid hormone transfer for fetal development, human placental development is itself responsive to thyroid hormone from early in gestation with evidence of trophoblastic expression of thyroid hormone receptors. T3 has been shown to promote proliferation, invasion, and production of epidermal growth factor by first trimester primary trophoblast cultures. In humans T3 has been shown to suppress apoptosis and down regulate Fas and Fas-ligand expression (18). It has been postulated that abnormal thyroid hormone levels could give rise to malplacentation which underlie the association between maternal thyroid dysfunction and adverse obstetric outcome.
Table 56.2 Immunologic and Hormonal Features of Pregnancy | ||||||||||||
---|---|---|---|---|---|---|---|---|---|---|---|---|
|
Immunologic and Hormonal Aspects of Normal Pregnancy
Pregnancy has a significant effect on the immune system, in order to maintain the fetal–maternal allograft, which is not rejected despite displaying paternal histocompatibility antigens (Table 56.2). While there is no overall immunosuppression during pregnancy, clinical improvement usually occurs in patients with immunologic disorders such as rheumatoid arthritis (RA) when they become pregnant. Clinical improvement occurs as well in psoriatic arthritis and Graves’ disease. On the other hand, systemic lupus erythematosus (SLE) may flare during pregnancy.
The trophoblast does not express the classical major histocompatibility complex (MHC) class Ia or II which are needed to present antigenic peptides to cytotoxic cells and T helper cells respectively. Instead HLA-G, a non-classical MHC Ib molecule is expressed which may be a ligand for the natural killer (NK) cell receptor so protecting the fetus from NK cell damage; it may also activate CD8+ T-cells that may have a suppressor function. Human trophoblasts also express the Fas ligand abundantly, thereby contributing to the immune privilege in this unique environment possibly by mediating apoptosis of activated Fas expressing lymphocytes of maternal origin.
T-cell subset studies in pregnancy are discrepant, as peripheral blood CD4+ and CD8+ cell levels have been variously reported to decline, remain unchanged, and increase during pregnancy. Although, the distinction between T cell helper 1 (Th1) and T cell helper 2 (Th2) immune responses in humans remains less clear than in the mouse, the general agreement is that in pregnancy there is a bias toward a Th2 response (19). This seems to be achieved by the fetal/placental unit producing Th2 cytokines, which inhibit Th1. Th1 cytokines are potentially harmful to the fetus as, for example, interferon alpha (IFNα) is a known abortifacient. The characterization of regulatory T cells (CD4+ CD25+), a T cell subset that can prevent experimental autoimmune disease, has improved the understanding of the immunologic maintenance of pregnancy. It is now thought that these cells are one of the main groups of T cell subsets which allow tolerance of the fetal semi-allograft. They may be found in the decidua as well as in the maternal circulation and regulate autoimmune responses (3).
Sex steroids are powerful negative regulators of B cell activity. Estrogen alone is effective in reducing B cell lymphopoiesis in pregnancy. Although progesterone is not effective on its own, it reduced the amount of estrogen required for suppression by up to 90% in a mouse pregnancy model and also acts at the maternal–fetal interface (20). The high concentrations of estrogen produced in normal pregnancy almost certainly contribute to the fall in autoantibody levels observed in pregnant patients with autoimmune thyroid disease (21). Despite the fall in autoantibodies, there are no significant changes reported in
the number of B cells in the circulation in normal human pregnancy. While progesterone may favor Th2 cells, evidence has indicated that estrogen delivers a negative signal to B cell function during pregnancy and this showed a slow reversal in the postpartum period. In keeping with these observations, autoantibody titres and inflammation fall throughout pregnancy as observed in all autoimmune diseases investigated. However, after most pregnancies, there is a marked increase in many different types of autoantibody secretion and an exacerbation of autoimmune diseases in the months after delivery (21). Cortisol, norepinephrine and 1,25-dihydroxyvitamin-induced inhibition and subsequent rebound of interleukin 12 (IL-12) and tumor necrosis factor alpha (TNFα) production may represent a major mechanism by which pregnancy and postpartum alters the course of or susceptibility to various autoimmune disorders. Galectin-1, an immunoregulatory glycan-binding protein, also has a pivotal role in conferring fetomaternal tolerance (22).
the number of B cells in the circulation in normal human pregnancy. While progesterone may favor Th2 cells, evidence has indicated that estrogen delivers a negative signal to B cell function during pregnancy and this showed a slow reversal in the postpartum period. In keeping with these observations, autoantibody titres and inflammation fall throughout pregnancy as observed in all autoimmune diseases investigated. However, after most pregnancies, there is a marked increase in many different types of autoantibody secretion and an exacerbation of autoimmune diseases in the months after delivery (21). Cortisol, norepinephrine and 1,25-dihydroxyvitamin-induced inhibition and subsequent rebound of interleukin 12 (IL-12) and tumor necrosis factor alpha (TNFα) production may represent a major mechanism by which pregnancy and postpartum alters the course of or susceptibility to various autoimmune disorders. Galectin-1, an immunoregulatory glycan-binding protein, also has a pivotal role in conferring fetomaternal tolerance (22).
Assessment of Thyroid Function in Pregnancy
Maternal thyroid dysfunction during pregnancy may affect maternal health, fetal health, and obstetric outcome. As there is significant overlap between the symptoms experienced by normal euthyroid pregnant women and those with thyroid dysfunction, clinical diagnosis is not always straightforward. Because thyroid physiology is altered in pregnancy it has become clear during the past decade that normative gestational reference ranges for thyroid hormone analytes are necessary. Most clinical laboratory reports only provide non-pregnant reference intervals for the interpretation of laboratory results.
Unfortunately, few if any FT4 immunoassay manufacturers provide appropriate normal pregnancy-related reference intervals that are method-specific (specific for the method used for hormone analysis). It is, therefore, imperative that method- and gestation-specific reference intervals for FT4 are derived in the appropriate reference populations to prevent misinterpretation of thyroid status in pregnant women While “gold standard methodology” (e.g., tandem mass spectrometry) is useful for accurate standardization of values, in practice the use of kit assays for free thyroid hormones as well as routine estimation of total bound hormones are used. Gestational reference ranges for theses hormones as well as TSH should be available in every hospital dealing with pregnancy (4).
In general, serum TSH concentrations provide the first clinical indicator for thyroid dysfunction. Due to the log-linear relationship between TSH and FT4, very small changes in T4 concentrations will provoke very large changes in serum TSH. However, in pregnancy, thyroid and pituitary functions are less stable. During early gestation, TSH is suppressed by 20% to 50% by week 10 due to the steep increase in hCG concentrations, resulting from increased hCG-induced thyroidal secretion of T4 and T3. Therefore, maternal serum TSH does not provide a good indicator for the control of treatment of thyroid dysfunction in the first trimester unless trimester specific ranges are available. False readings can lead to maternal under-replacement with LT4, or overtreatment with antithyroid drugs both of which can result in both maternal hypothyroidism and an increased risk for adverse fetal brain development. TSH is, however, the best measure of thyroid function during the second and third trimesters.
It has been easier to develop assays for total thyroid hormones compared to free as the former have increased serum concentrations in addition to being more accurate and valid than free hormone assays. However, changes in the concentration of hormone binding proteins have a big effect on the total hormone concentration; for example, by the second trimester, total T4 concentration increases to 1.5 times the concentration typical in the non-pregnant individual. Although total T4 assays generally agree quite well, unfortunately, many clinical laboratories only employ free hormone assays.
These are based on analogue methods that rely on the concentrations of binding-proteins, are method-dependent and may give misleading FT4 and FT3 values in pregnancy. For example, a commercially available FT4 assay has shown that it correlates more closely to total T4 assays than to FT4 measured following physical separation from binding proteins (23). A comparison of five different commercial assays for FT4 and FT3 showed significant interassay variation underlining the necessity for individual laboratory based reference ranges (24). FT4 assays are considered to be flawed and unreliable during pregnancy (25) but there are data showing that, despite susceptibility toward binding protein alterations, these assays may indeed reflect the gold standard assays (26). Direct assays with equilibrium dialysis are expensive, laborious to perform and not widely available. However, free T4 index (FTI) values (not always available in hospital and commercial laboratories) do closely reflect FT4 by equilibrium dialysis (25). The FT4 and the equilibrium dialysis methods both show a fall in FT4 mainly in the third trimester.
Although gestation-specific reference intervals for thyroid function tests are not currently in routine use in most laboratories, there has been intense activity worldwide in the development of such ranges (4). Since 2007, further country data has been documented (27,28,29,30,31). Emphasis has also been placed on obtaining first trimester ranges (32,33,34,35).
Reliable trimester-specific (or gestation-specific) reference intervals for TSH are also now available, being based on an adequate sample size comprised singleton pregnancies in an iodine sufficient, antibody-free population. The individual genetic set-points of a population may result in an intra-individual variability of the thyroid hormone levels, reflected by the reference intervals (34).
In summary, TSH levels may be misleading in the first trimester and T4 values either total or free will give a more accurate estimate of clinical status. Later in gestation, TSH levels are reliable, whereas T4 may fall especially in the third trimester but this does not indicate hypothyroidism. In some cases, serum anti-TPO antibodies, anti-Tg and/or TSHR antibody levels can provide further information; TPO antibodies can predict the risk of hypothyroidism. In pregnant women with low TSH hyperthyroidism, this is accompanied by TSHR antibodies in 60% to 70% of the cases.
Hyperthyroidism
Although Graves’ disease is the most recognized etiology of hyperthyroidism (2,37,38), other causes should be considered, particularly the syndrome of “Transient Non-autoimmune hyperthyroidism of early pregnancy” (39), induced by high serum levels of hCG or a molecular variant with higher metabolic activity (see section on thyroid function in
pregnancy). The proper diagnosis is of paramount importance, because medical management and obstetrical outcome are quite different (Table 56.3).
pregnancy). The proper diagnosis is of paramount importance, because medical management and obstetrical outcome are quite different (Table 56.3).
Table 56.3 Causes of Hyperthyroidism in Pregnancy | |||||||||||||||||||||||||
---|---|---|---|---|---|---|---|---|---|---|---|---|---|---|---|---|---|---|---|---|---|---|---|---|---|
|
Transient Non-autoimmune Hyperthyroidism of Early Pregnancy
Also known as Gestational Thyrotoxicosis (GT), Transient Hyperthyroidism (TH) or Transient Gestational Hyperthyroidism (GTT), is defined as transient hyperthyroidism of non-autoimmune etiology in the first trimester of pregnancy (39). The most common cause of the syndrome is the one associated with hyperemesis gravidarum (HG), known as “Transient Hyperthyroidism of Hyperemesis Gravidarum” (THHG) (40); other less common causes are multiple pregnancies and hydatidiform mole. Isolated cases reported in the literature from other etiologies are shown in Table 56.1.
Transient Hyperthyroidism of Hyperemesis Gravidarum
HG is reported to occur in 0.3% to 1.0% of pregnancies; it is defined as persistent nausea and vomiting in the first trimester of pregnancy, resulting in greater than 5% weight loss, ketonuria, dehydration, liver and electrolyte abnormalities (hypokalemia, metabolic alkalosis, hyponatremia, hypochloremia) in severe cases (41). The onset of nausea is at about 4 to 6 weeks’ gestation, with worsening by 7 to 9 weeks’ gestation, resolution by the end of the first trimester in 60% of cases, and complete resolution by 20 weeks in most women.
The incidence of hyperthyroidism in women with HG depends on the severity of symptoms, ethnic background, perhaps dietary iodine intake, interpretation of thyroid tests and other unknown factors. The diagnosis of THHG is based on the presence of clinical and physical clues: Lack of hyperthyroid symptoms before conception (since most patients with Graves’ disease diagnosed for the first time during gestation give a history of hypermetabolic symptoms antedating conception), similar symptoms in previous pregnancies, and absence of goiter or Graves’ ophthalmopathy. Serum FT4 or free thyroxine index (FT4I) are far above the reference range, serum TSH is suppressed or undetectable and markers of thyroid autoimmunity, such as TPO antibodies and TRAb are almost always absent. In less than 20% of affected women, serum TT3 is slightly elevated.
Ethnic variation in the incidence of HG has been suggested by several studies. A birth registry in Norway from 1967 to 2005, revealed an overall prevalence of 0.9%; however, when broken down by ethnicity, it affected 2.2% of Pakistani women; 1.9% of Turkish women and 0.5% of Norwegian women (42); a familial aggregation was suggested by Zhang et al. (43), sisters and mothers were more affected than controls, suggesting strong evidence for a genetic component of HG.
An association between HG and hyperthyroidism was first described in 1978 (44); the authors studied 35 women with HG, of whom 14 had a diagnosis of hydatidiform mole, and 57 normal control pregnancies. In both conditions, HG and hydatidiform mole, there was an accompanying increase in serum hCG concentrations as compared to control women. They reported high values of protein binding iodine (PBI) in most patients with hydatidiform mole and in almost one-third of HG women. In the largest series of HG women with hyperthyroidism (40), of the 67 HG patients, 66% of them had biochemical hyperthyroidism. Forty-two percent of women had abnormal liver function tests and 6% had an elevation in serum amylase titers. Abnormal electrolytes, hyponatremia, hypokalemia, and elevated bicarbonate levels were found in 28% of cases, mostly related to the degree of dehydration due to vomiting. FT3I elevation was detected in only six women related to severity of the reported vomiting. No patient received ATD therapy; all had spontaneous resolution of vomiting by 18 weeks’ gestation, with normalization of the thyroid tests by the time vomiting ceased; more severe vomiting was associated with a greater degree of thyroid stimulation and greater concentration of hCG (Fig. 56.2). Bioassay of serum HG for thyrotropic activity in thyroid cell culture, demonstrated a significant correlation between the serum concentration of hCG and the iodine uptake (40). Similar observations were reported in a group of 53 pregnant Asian women presenting with vomiting, five of them diagnosed with Graves’ disease. In the women with THHG, T4 levels normalized by 15 weeks’ gestation, and TSH levels normalized by 19 weeks. Birth weight was lower in mothers experiencing a weight loss of >5% of their prepregnancy weight, as compared to women who did not, although not significant (p = 0.093) (45). The syndrome may recur in future pregnancies.
Antithyroid medications are not required in most cases. In one series in which antithyroid medication was used, pregnancy outcome was not significantly different to a similar group
of patients receiving no therapy (46). Occasionally, severe vomiting and hyperthyroidism may require parenteral nutrition. Treatment with β-adrenergic blocking agents is effective in controlling the symptoms.
of patients receiving no therapy (46). Occasionally, severe vomiting and hyperthyroidism may require parenteral nutrition. Treatment with β-adrenergic blocking agents is effective in controlling the symptoms.
The differential diagnosis from Graves’ hyperthyroidism may be difficult, as vomiting may also be a presenting symptom of hyperthyroidism of Graves’ disease. The diagnosis of THHG should be considered in women with severe vomiting, no clinical manifestations of Graves’ disease, and biochemical evidence of hyperthyroidism. Vomiting should be persistent and severe with a significant weight loss, since most women with morning sickness of pregnancy have normal thyroid function tests (38). Thyroid gland color flow Doppler sonography was evaluated in 10 hyperthyroid patients in pregnancy, three with Graves’ disease, four with GT and three with destructive thyroiditis. Graves’ women had a greater thyroid gland volume, and greater thyroid vascularity as compared to the other two groups, on whom the ultrasonograms findings were not different between them (47).
Hyperemesis gravidarum may also occur in women with Graves’ hyperthyroidism and in those with a previous history of Graves’ hyperthyroidism in remission; this is explained by the thyrotrophic action of hCG early in gestation. The differential diagnosis between the two entities may be difficult, the presence of TRAb favoring the diagnosis of Graves’ hyperthyroidism (48).
Trophoblastic diseases, partial and complete hydatidiform moles, and choriocarcinoma are other causes of hyperthyroidism early in pregnancy (12). Patients may present without symptoms in spite of chemical hyperthyroidism, or with various degrees of severity, including congestive heart failure. Evacuation of the mole eliminates the source of the excessive hCG and reverses the clinical and biochemical features of hyperthyroidism.
Graves’ Disease
The natural course of Graves’ hyperthyroidism is characterized by an exacerbation of symptoms in the first trimester and during the postpartum period with an improvement in the second half of pregnancy. Stimulation of the thyroid gland by placenta hCG in the first trimester and an elevation in serum TRAb concentration have been suggested as the cause of disease exacerbation (49). Immunologic responses caused by changes in lymphocyte subsets could explain spontaneous improvement in the second half of pregnancy and recurrences in the postpartum period (3). Kung and Jones (50), in a study comparing Graves’ disease in pregnant and non-pregnant women, postulated that the amelioration of symptoms seen with progression of pregnancy was due to a decrease in the titers of TRAb with stimulating activity and an increase in TRAb with thyroid-blocking activity. The reverse was true in the postpartum period, when aggravation of Graves’ hyperthyroidism usually occurs. In another study, it was suggested that the amelioration of Graves’ disease in the last half of pregnancy is induced by a decrease of TRAb (TSI) but not by the appearance of thyroid stimulation-blocking antibodies (TSBAs) (51).
When hyperthyroidism is properly managed throughout pregnancy, the outcome for mother and fetus is good; however, maternal and neonatal complications for untreated or poorly controlled mothers are significantly increased (2,37,38,52). One situation in which the fetus may be severely affected, is the euthyroid pregnant woman previously treated for Graves’ hyperthyroidism by ablation therapy, surgery or 131 Iodine, with persisting high titers of TRAb with stimulating activity following ablation therapy. Careful follow-up during pregnancy with appropriately treatment will ensure that serious and sometimes irreversible complications are prevented (53).
Prepregnancy Counseling
Therefore, prepregnancy counseling plays a very important role in the care of young women with Graves’ disease. All women of childbearing age affected with Graves’ hyperthyroidism should
be strongly advised to seek contraception counseling, in order to avoid pregnancy while hyperthyroid (2,37). A discussion of the different hyperthyroid therapeutic choices is important for those women planning a pregnancy: Ablative therapy, by 131I or surgery, or medical therapy.
be strongly advised to seek contraception counseling, in order to avoid pregnancy while hyperthyroid (2,37). A discussion of the different hyperthyroid therapeutic choices is important for those women planning a pregnancy: Ablative therapy, by 131I or surgery, or medical therapy.
If ablative therapy is chosen, the following recommendations are suggested:
Pregnancy test prior to ablation
Delay of conception on average of 6 months following therapy in order to adjust LT4 doses to target values for pregnancy (serum TSH 0.3–2.5)
Determination of TSH-receptor antibody (TRAb); the gradual disappearance from the circulation post therapy depends on the type of treatment chosen; following thyroidectomy, there is a gradual disappearance of TRAb titers, while following 131I therapy, there is an increase in TRAb titers that may last for 12 months followed by a gradual fall in titers (54). Therefore, in patients with high TRAb titers, surgery appears to be the therapy of choice in women contemplating pregnancy (53).
For women on antithyroid drugs, the following discussion with the patient and her family is recommended:
If still in need of ATD for more than 2 years, the possibilities of remission during pregnancy are very low, the patient, most likely, will need ATD therapy through pregnancy.
PTU may induce liver toxicity with potential liver failure requiring liver transplantation; therefore, recent recommendations by the American Thyroid Association (55) limited the use of PTU to those patients allergic to MMI, in the treatment of thyroid storm and in the first trimester of pregnancy because of the rare instances of methimazole (MMI) embryopathy. In a woman on MMI therapy at the time of conception or planning a pregnancy, it is advisable to switch to PTU and reassume MMI after the first trimester. A potential risk of these recommendations is that the patient will be exposed to the potential adverse side effects of both drugs rather than one.
Close follow-up throughout the pregnancy for frequent blood tests and adjustment of ATD dose, since the need for dose adjustments is common.
Possibility of disease aggravation in the first trimester and recurrence in the postpartum period, due to postpartum thyroiditis (PPT) or recurrence of Graves’ (48). Recommendations regarding breastfeeding while on ATDs.
TRAb determination for prediction of potential fetal or neonatal complications.
Clinical Presentation
In most of the patients in whom the diagnosis of Graves’ hyperthyroidism is made for the first time in pregnancy, hyperthyroid symptoms antedate conception for several months. The clinical presentation of Graves’ hyperthyroidism in women of reproductive age spans a broad spectrum. There are five common scenarios in which women with a history of Graves’ disease may present at booking (38):
Initial diagnosis of hyperthyroidism in pregnancy, with the majority of cases having symptoms antedating pregnancy
Active disease on antithyroid therapy at the time of conception
Recurrence of hyperthyroidism early in pregnancy in patients on remission from antithyroid therapy
Prior treatment of hyperthyroidism by surgery or 131I
Previous pregnancies affected by birth of an infant with thyroid dysfunction
Clinical Manifestations
The clinical diagnosis of thyrotoxicosis may present difficulties during gestation, since many symptoms and signs are commonly seen in normal pregnancy, such as mild palpitations, heart rate between 90 and 100 beats/min, mild heat intolerance, shortness of breath on exercise, and warm skin. There are some clinical clues that increase the likelihood of autoimmune hyperthyroidism: Symptoms antedating conception, family history of thyroid disease or other autoimmune conditions, presence of goiter, ophthalmopathy, vitiligo, proximal muscle weakness, tachycardia with a pulse rate over 100 beats/min, pruritus, and weight loss or inability to gain weight in spite of a good appetite.
Severe ophthalmopathy is rare in pregnancy; glucocorticoid therapy and surgical orbital wall decompression may be required to restore visual activity as in one reported case (56).
Laboratory Tests
The diagnosis is confirmed by an elevated FT4 or FT4I value in the presence of undetectable serum TSH. In some unusual situations, the serum FT4 or the FT4I may be at the upper limit of normal or be slightly elevated, in which case the determination of TT3 or FT3I will confirm the diagnosis of hyperthyroidism. Markers for thyroid autoimmunity are essential in the evaluation of hyperthyroidism in pregnancy. Thyroid peroxidase antibodies (anti-TPO) are elevated in most patients with Graves’ disease; serum TRAb, measured by using a radioreceptor assay, TSHR-binding inhibitory antibodies (TBII) assay, or an in vitro bioassay measuring the generation of cyclic adenosine mono-phosphate (cAMP) when the patient’s serum is incubated with cells that express the TSHR is present in almost every patient at the time of hyperthyroidism diagnosis.
Table 56.4 Hyperthyroidism Complications | |||||||||||||||||||||||||
---|---|---|---|---|---|---|---|---|---|---|---|---|---|---|---|---|---|---|---|---|---|---|---|---|---|
|
Complications
They may be grouped as maternal, obstetric, and neonatal (Table 56.4). Untreated hyperthyroidism carries a high risk of complications (57) including maternal left ventricular dysfunction, congestive heart failure (58), thyroid crisis (59), miscarriage, gestational hypertension, intrauterine death, prematurity, low birth weight (LBW) infants (60), and transient central hypothyroidism (61). Left ventricular decompensation in hyperthyroid pregnant women may develop early in pregnancy because of hyperthyroidism aggravation, late in pregnancy due to superimposed preeclampsia, at the time of delivery or at any time in pregnancy because of undercurrent complications such as anemia or infection (58). Millar et al. (60) compared the obstetric outcome of 57 women that remained hyperthyroid at the time of delivery, with 34 treated or in remission
who remained euthyroid through pregnancy. The incidence of preeclampsia was 14%, prematurity 33.3%, and LBW infants (<2500 g) was 43.8% as compared to 6.2%, 5.8%, and 6.2% respectively. Phoojaroenchanachai et al. (52) reported 35 pregnancies remaining hyperthyroid in the third trimester compared to 153 women achieving euthyroidism by then. The prevalence of infants with LBW (<2500 g) was 22.9% as compared to 9.8% in the euthyroid group (OR 2.7, 95% CI = 1.3-6.1 p = 0.038); multiple logistic regression analyses showed that maternal hyperthyroidism during the third trimester of pregnancy was an independent factor for LBW infants (OR 4.1, 95% CI = 1.1-15.0 p = 0.037). Mitsuda et al. (62) correlated the risk of delivering a small-for-gestational-age (SGA) infant to the presence of maternal thyrotoxicosis lasting more than 30 weeks of pregnancy, a duration of Graves’ disease for most of 10 years, and onset of Graves’ disease before age 20. Neonatal goiter was an early recognized complication after the introduction of ATD in pregnancy, as a result of excessive ATD doses, and avoided if the dose was reduced in the last trimester of pregnancy or discontinued all together. On occasion, injecting levothyroxine in the amniotic fluid resolves the fetal goiter (63). In the last 20 years, however, there has been a significant decrease in the incidence of maternal, obstetric, and neonatal complications directly related to several factors, including preconception counseling, a professional team approach concept with the close collaboration between endocrinologists, obstetricians, maternal fetal medicine specialists and neonatologists, better understanding of the natural course of the disease, proper interpretation of thyroid tests including TRAb values, patient selection in assessing the need for fetal wellbeing testing and proper use of antithyroid medications (2,37,38,57).
who remained euthyroid through pregnancy. The incidence of preeclampsia was 14%, prematurity 33.3%, and LBW infants (<2500 g) was 43.8% as compared to 6.2%, 5.8%, and 6.2% respectively. Phoojaroenchanachai et al. (52) reported 35 pregnancies remaining hyperthyroid in the third trimester compared to 153 women achieving euthyroidism by then. The prevalence of infants with LBW (<2500 g) was 22.9% as compared to 9.8% in the euthyroid group (OR 2.7, 95% CI = 1.3-6.1 p = 0.038); multiple logistic regression analyses showed that maternal hyperthyroidism during the third trimester of pregnancy was an independent factor for LBW infants (OR 4.1, 95% CI = 1.1-15.0 p = 0.037). Mitsuda et al. (62) correlated the risk of delivering a small-for-gestational-age (SGA) infant to the presence of maternal thyrotoxicosis lasting more than 30 weeks of pregnancy, a duration of Graves’ disease for most of 10 years, and onset of Graves’ disease before age 20. Neonatal goiter was an early recognized complication after the introduction of ATD in pregnancy, as a result of excessive ATD doses, and avoided if the dose was reduced in the last trimester of pregnancy or discontinued all together. On occasion, injecting levothyroxine in the amniotic fluid resolves the fetal goiter (63). In the last 20 years, however, there has been a significant decrease in the incidence of maternal, obstetric, and neonatal complications directly related to several factors, including preconception counseling, a professional team approach concept with the close collaboration between endocrinologists, obstetricians, maternal fetal medicine specialists and neonatologists, better understanding of the natural course of the disease, proper interpretation of thyroid tests including TRAb values, patient selection in assessing the need for fetal wellbeing testing and proper use of antithyroid medications (2,37,38,57).
Fetal Thyroid Dysfunction (See Chapter 53)
Fetal goiter and thyroid dysfunction are potential complications in fetuses of mothers with past history or active Graves’ hyperthyroidism; inappropriate high doses of ATD may induce fetal hypothyroidism even if maternal thyroid function is within the normal reference range, while high serum TRAb concentration in the second half of pregnancy may trigger fetal hyperthyroidism.
Fetal Hyperthyroidism
Although rare, this is a preventable complication with potential severe sequelae, including death. A very incisive clinical evaluation at the time of maternal hyperthyroidism diagnosis, will allow physicians to sort out the few women at risk, such as women with: (a) active Graves’ hyperthyroidism, (b) previous history of Graves’ disease treated with ablation therapy, either surgery or 131I, and (c) mothers with active Graves’ hyperthyroidism undergoing therapeutic thyroidectomy in the second trimester of pregnancy (53). A determination of TRAb titer should be obtained between 22 and 26 weeks’ gestation, a value of 3 to 5 times above normal is an indication for fetal evaluation for detection of potential fetal thyrotoxicosis. The fetal thyroid TSHR starts responding to thyroid stimulating immunoglobulins (TSI) stimulation during the second trimester. The placental transfer of IgG from mother to fetus increases by the end of the second trimester, reaching a level in the fetus similar to that of the mother around 30 weeks’ gestation (2). Therefore, the symptoms of fetal hyperthyroidism are not evident until 22 to 26 weeks of gestation. Early signs of disease include presence of fetal goiter first and then fetal tachycardia, defined as persistent fetal heart rate of >160 bpm. A fetal heart monitor tracing showing a sustained baseline of 170 to 180 beats/minute with moderate variability that exhibits acceleration with a lack of deceleration was stated to be a pattern unique to fetal thyrotoxicosis (64). Other signs developing later are intrauterine growth restriction (IUGR), oligohydramnios or hydrops, and accelerated bone maturation. This last sign is difficult to detect. However its presence is highly predictive of the disease. Normal distal femoral ossification by 33 weeks is <3 mm in diameter and not visible consistently; accelerated bone maturation is diagnosed by the presence of distal femoral ossification center before 31 weeks’ gestation (65,66). Serial cordocentesis for monitoring drug therapy has been proposed, but its value has been questioned, restricted to centers with expertise (67).
Neonatal Hyperthyroidism
Neonatal hyperthyroidism is infrequent, with an incidence of less than 1% of infants born to mothers with Graves’ disease, therefore, affecting 1 in 50,000 neonates. If neonatal hyperthyroidism is not recognized and treated properly; neonatal mortality could be as high as 30%. Since the half-life of the antibodies is only a few weeks, complete resolution of neonatal hyperthyroidism is the rule (68).
Sporadic cases of neonatal hyperthyroidism without evidence of the presence of circulating TSI in mother or infant are
due to activating of mutations in the TSHR molecule (69,70). It is inherited as an autosomal dominant trait and, in contrast to Graves’ neonatal hyperthyroidism, the condition persists indefinitely. Treatment with antithyroid medications followed by thyroid ablation therapy will eventually be needed.
due to activating of mutations in the TSHR molecule (69,70). It is inherited as an autosomal dominant trait and, in contrast to Graves’ neonatal hyperthyroidism, the condition persists indefinitely. Treatment with antithyroid medications followed by thyroid ablation therapy will eventually be needed.
Neonatal Central Hypothyroidism
Infants of untreated hyperthyroid mothers may be born with transient central hypothyroidism (pituitary or hypothalamic origin). High levels of thyroxine crossing the placenta barrier feedback to the fetal pituitary with suppression of TSH. The diagnosis is made in the presence of low FT4 and normal or low TSH in cord blood. This is another preventable complication with proper management of maternal hyperthyroidism (61)
Management of Hyperthyroidism
Controlling hyperthyroidism is essential to prevent maternal, fetal, and neonatal complications. The aim of treatment is normalization of thyroid tests as soon as possible, with the minimum amount of antithyroid medication. The exception are those few patients in whom TRAb titers are above three- to fivefold above reference range, in which cases higher doses may be needed in cases of fetal hyperthyroidism. The risk criteria for serum TRAb values varies in the reported literature, mainly because of the different methods used to assess TRAb (71).
Patients should be assessed at regular intervals, every 2 to 4 weeks at the onset of treatment and every 4 weeks thereafter, to allow for proper medication adjustments to keep the FT4 or FT4I within target goals. Clinical clues of good therapeutic response are improvement in symptoms and weight gain. The use of lower dose of ATD was suggested in the past in order to avoid fetal goiter. Momotani et al. (72) showed that neonatal serum TSH was elevated even in mothers with serum FT4 values within the normal range; furthermore, they demonstrated that at the time of normalization of maternal serum TT3 during ATD therapy, maternal serum FT4 was well within normal limits, a risk factor for the development of fetal hypothyroidism, supporting the recommendations that serum TT3 determination should rarely be used to assess maternal function during gestation.
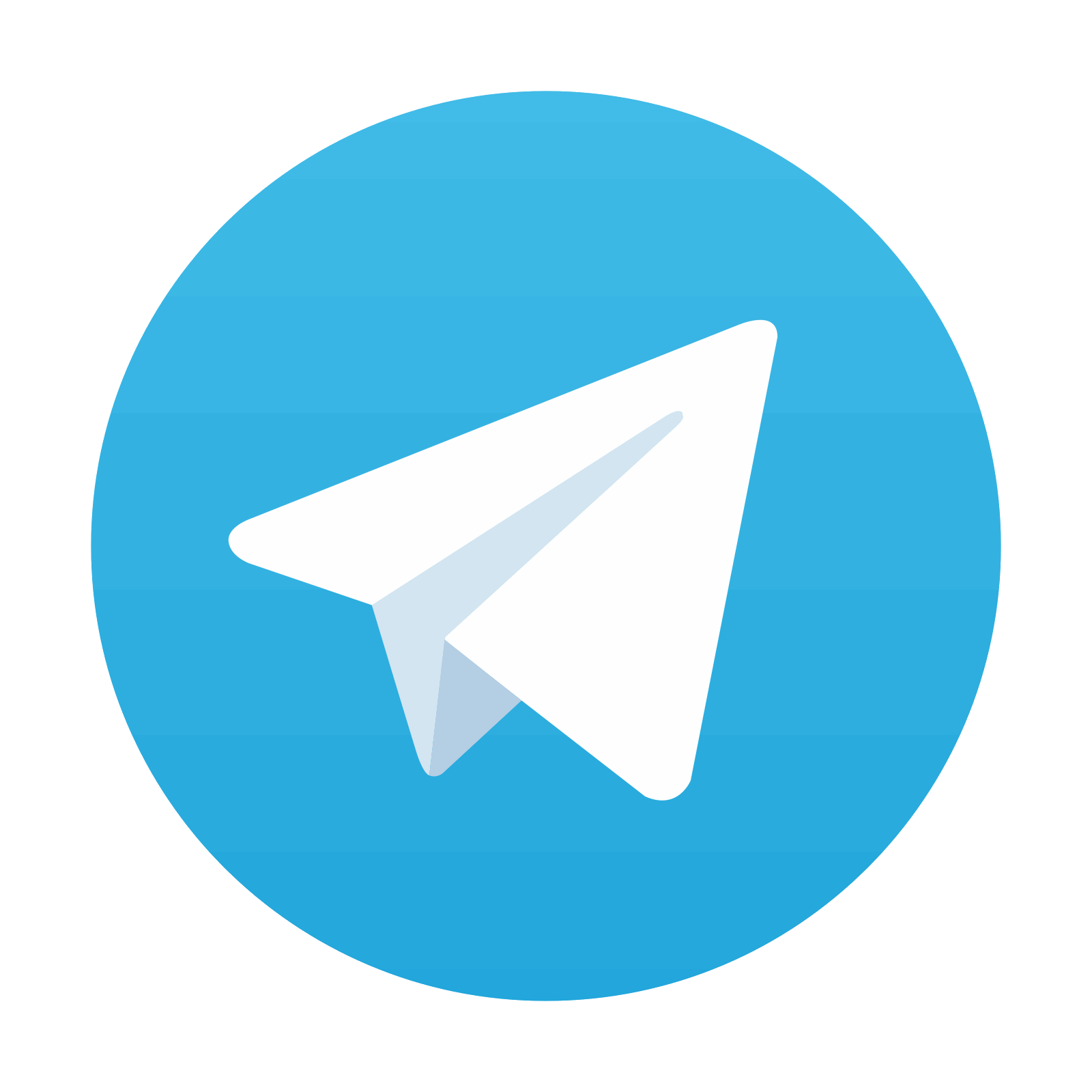
Stay updated, free articles. Join our Telegram channel

Full access? Get Clinical Tree
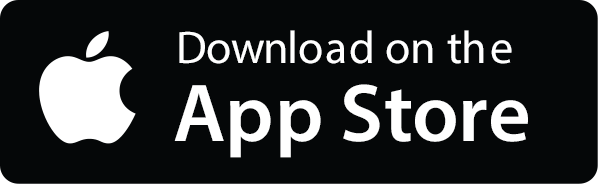
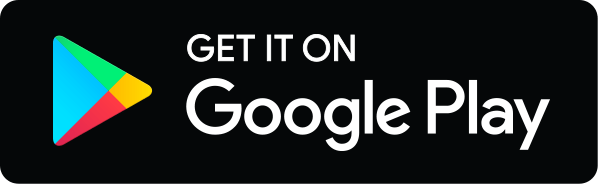